Reader activities
Having read this scenario, consider the following:
- What are the clinical manifestations of hyperglycaemia?
- What effect does hyperglycaemia have on other organs?
- Why does hyperglycaemia occur in critical illness?
- How should hyperglycaemia be managed?
Pathophysiology
Hyperglycaemia has been varyingly defined as a blood glucose level of >6.1 mmol/L (Van den Berghe et al. 2001) to one of >10 mmol/L (Devos and Preiser 2004). In critical illness, hyperglycaemia has been thought to benefit organs by allowing them a greater energy supply during a period of stress (Parsons and Watkinson 2007). Stress experienced in critically ill patients causes an increase in cortisol, growth hormones, epinephrine and glucagon to be produced, all of which act to increase the hepatic production of glucose (Montori et al. 2002). The usual mechanism by which blood glucose is controlled relies on insulin produced by the pancreas. Insulin reduces glucose production by the liver and enhances the uptake of glucose by the skeletal muscle. This assists in the storage of glucose as glycogen or for use in the Krebs cycle for energy production (Robinson and Van Soeren 2004).
In critical illness, uptake of glucose by the peripheries (i.e. skeletal muscle) is reduced (Montori et al. 2002) possibly due to a decrease in exercise-stimulated glucose uptake, as a result of the patients’ immobility (Vanhorebeek et al. 2005). This decreased utilisation by the peripheries is known as insulin resistance (Robinson and Van Soeren 2004). This increase in hepatic glucose release and reduced peripheral uptake causes hyperglycaemia despite insulin release from the pancreas. Thus, as a patient in a high-dependency area, Lilly would have raised glucose levels due to her body increasing hepatic glucose production in response to stress and due to her immobility.
Lilly’s hyperglycaemia may also be exacerbated by excess calorie provision from dextrose infusions for fluid resuscitation and medication administration. Lipid-based infusions such as Propofol contain calories which increase blood glucose levels. Steroids and immunosuppressant drugs can also cause hyperglycaemia (Montori et al. 2002). The hydrocortisone being administered to Lilly will increase hepatic glucose production and decrease glucose utilisation (Neal 2009). Additionally, Lilly’s continuous salbutamol nebulisers could lead to a reduced level of serum potassium as it causes potassium to shift from the plasma into the cells. This, in turn, will have an effect on glucose levels as these two positive ions exchange with each other.
Effects of hyperglycaemia
When glucose levels rise, the body tries to reduce this by excretion of glucose by the kidneys. Glucose is an osmotic force when in excess, and as it is excreted, it attracts electrolytes such as sodium and potassium to be excreted with it. This leads to a situation whereby the body becomes fluid depleted with electrolyte imbalance. In order to maintain cardiac output, the heart rate will rise and the renal arteries will constrict, attempting to increase glomerular filtration. However, over time as the hyperglycaemia persists, the glomerular filtration rate decreases and the kidneys’ ability to excrete glucose and electrolytes is also reduced. Renal markers of dysfunction will rise and poor tissue perfusion may cause the release of lactic acid from anaerobic metabolism in the cells (Morton and Fontain 2008). Lilly’s blood results are illustrative of the start of renal dysfunction with electrolyte imbalance and poor tissue perfusion, leading to a raised lactate. Acute kidney injury is explained in detail in Chapter 9.
Hyperglycaemia and outcome
Studies on specific groups of patients have found that hyperglycaemia affects outcome. DiNardo et al. (2004) reported altered fluid balance and dehydration from increased urinary glucose, and McCowen et al. (2001) found increased inflammation and reduced immune functioning when hyperglycaemia occurred. In patients with myocardial infarction, hyperglycaemia was associated with a higher mortality rate and an increased risk of cardiogenic shock and congestive heart failure (Capes et al. 2000). The first major study looking at critical care patients was performed in 2001 by Van den Berghe et al. This is considered a landmark study. Findings appeared to be so beneficial to critical care patients that study recommendations to control blood glucose levels between 4.5–6.0 mmol/L were adopted worldwide (Wiener et al. 2008). Van den Berghe et al. (2001) found that controlling blood glucose to within this tight range significantly reduced mortality rates, length of critical care stay, ventilator time, septicaemia and the occurrence of neuropathy. The study was, however, performed solely on surgical patients in a single centre. When the same study was recreated in a medical critical care environment by Van den Berghe et al. in 2006, findings were less conclusive, reporting that critical care stay was reduced in patients who had their blood glucose controlled between 4.5–6.0 mmol/L but no difference was seen in mortality rates or bacteraemia. In patients who stayed in the critical care area for more than five days on intensive insulin therapy, mortality rates were, however, improved.
In another study on a large sample of general critical care patients, Krinsley (2003) found that a blood glucose between 4.4–5.5 mmol/L was significantly associated with reduced mortality. Mortality rates were observed to rise as blood glucose increased until patients with blood sugars exceeding 16.5 mmol/L had a 42.5% mortality rate. The sepsis care bundle also includes tight glucose control of <8.3 mmol/L as the best practice for the treatment of severe sepsis (Dellinger et al. 2008). Despite this evidence the biggest study to date looking at glucose control in a general critical care population found no benefit of keeping glucose between 4.5–6.0 mmol/L and, therefore, could not recommend it as the best practice for critical care patients (Finfer et al. 2009).
Tight glucose control has been found to have improved outcomes in some patient populations but not in all. Falciglia et al. (2009) found that the effects of hyperglycaemia on mortality were different depending on the admission diagnosis. Patients admitted with unstable angina, myocardial infarction and heart failure were found to be more at risk of higher mortality rates in the presence of hyperglycaemia than patients with chronic obstructive airways disease or post-coronary artery bypass graft. It needs to be recognised, therefore, that hyperglycaemia may affect the mortality rates in certain patients, so controlling hyperglycaemia is necessary in order to reduce associated complications in these patient groups. Lilly is therefore started on an infusion of insulin at a concentration of 1 unit/mL, titrated against a sliding scale.
Tests and investigations
Blood glucose testing
Several methods of blood glucose testing are available in the critical care setting. Arterial blood sampling via an arterial catheter is a common procedure to measure levels of oxygen, carbon dioxide, bicarbonate, lactate and electrolytes using a blood gas analyser. Many of the studies performed to look at glucose control used this method for all glucose results (Van den Berghe 2001, 2006; Finfer et al. 2009). This method is considered to be accurate and was found to be the most reliable method of measurement when compared to laboratory testing (Kanji et al. 2005). However, frequent arterial line sampling may increase the risks of air embolus, infection and anaemia in critical care patients (Parsons and Watkinson 2007). Samples must be taken using a non-touch technique and there is some support for closed blood sampling circuits which allow the blood to be returned to the patient to reduce the occurrence of chronic anaemia.
Point-of-care testing using capillary blood sampling and a bedside glucometer (see Figures 7.1, 7.2 and 7.3) would prevent the nurse from having to leave Lilly unattended to use a blood gas analyser for glucose measurements (Parsons and Watkinson 2007). Using capillary sampling, however, has been found to be the least accurate method of measuring glucose levels when compared to laboratory results. Kanji et al. (2005) found that capillary sampling over-estimated blood glucose significantly, and in cases of hypoglycaemia, results using this method agreed with laboratory results only 26.3% of the time. The use of arterial blood for glucometer testing is therefore preferable to capillary blood sampling (Van den Berghe et al. 2006; Finfer et al. 2009), and is thus recommended for use to monitor Lilly’s blood glucose levels (Dellinger et al. 2008; Finfer et al. 2009).
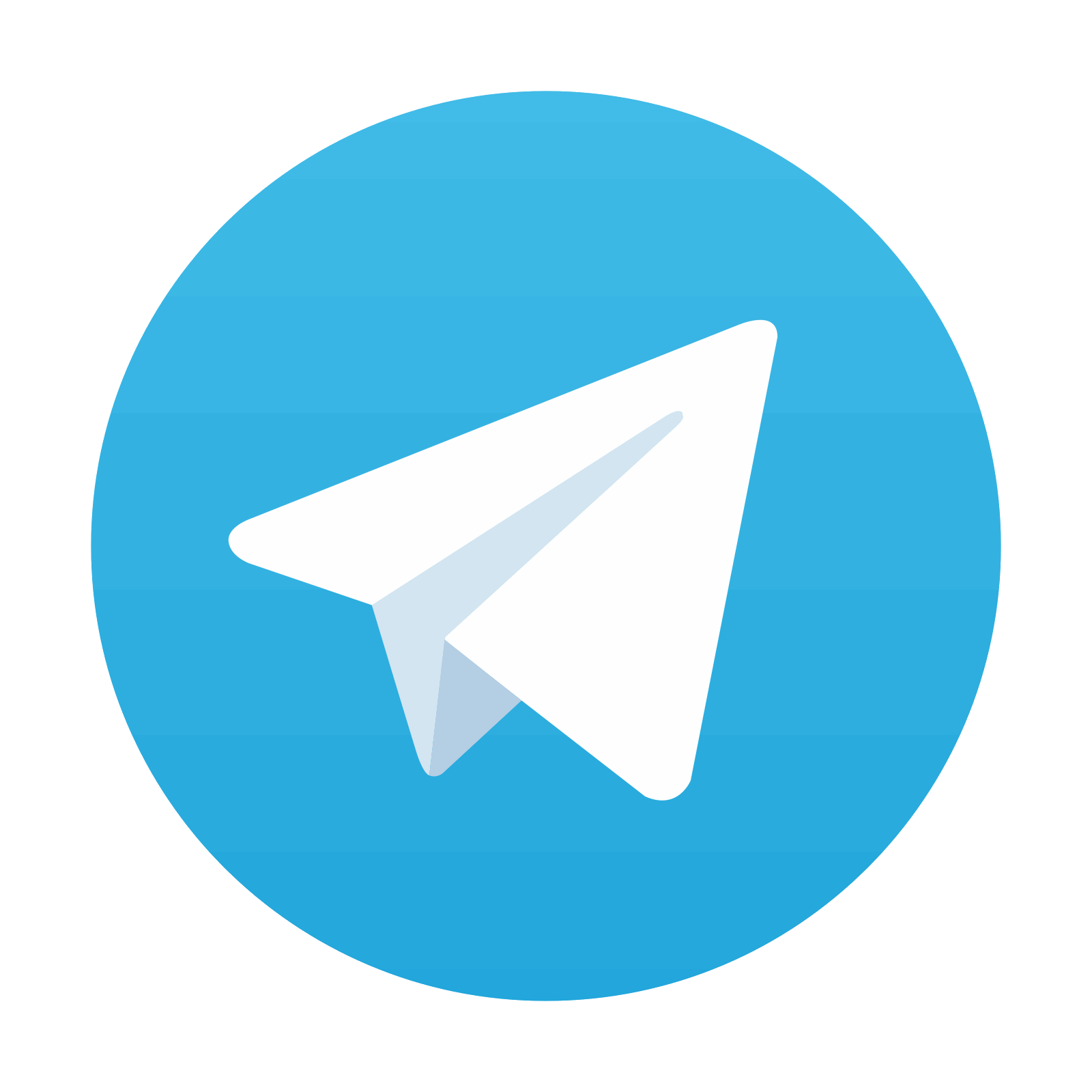
Stay updated, free articles. Join our Telegram channel

Full access? Get Clinical Tree
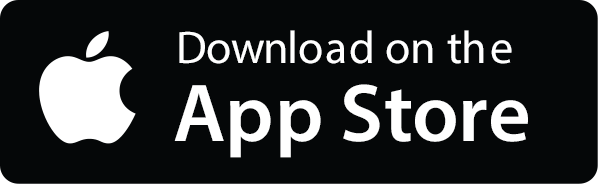
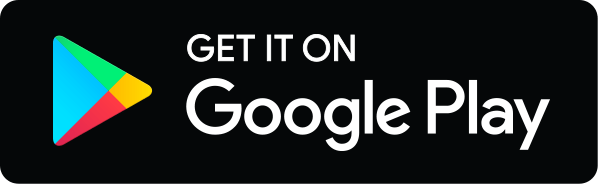