Seating, Positioning, and Support Surfaces 11
Objectives
After completing this chapter, you’ll be able to:
- discuss tissue biomechanical properties, their measurement, and their relationship to soft tissue–loading tolerance
- identify support surface characteristics related to the maintenance of tissue integrity
- describe the categories, functions, and limitations of various support surfaces
- outline an assessment process for selecting an appropriate support surface (seat cushion or horizontal support) and related interventions (positioning).
Preventing Pressure Ulcers
Multiple intervention strategies are needed to prevent and treat pressure ulcers. Managing loads on the skin and associated soft tissue is one of these strategies. A comprehensive care plan should include pressure redistribution strategies for individuals both while in bed and when seated. Properly chosen support surfaces; adequate periodic pressure redistribution; protection of especially vulnerable bony prominences, such as the heels, sacrum, and coccyx; and consideration of special patient needs are all essential components of the care plan.
A support surface is a specialized device for pressure redistribution designed for management of tissue loads, microclimate, and other therapeutic functions. Types of surfaces include mattresses, integrated bed systems, mattress replacements, mattress overlays, and seat cushions. Unless specifically identified as a mattress or seat cushion, the term “support surface” will refer to both product categories in this chapter. Achieving a good match between the patient’s needs and the performance capabilities of the support surface has a profound, positive impact on a patient’s health and well-being; conversely, a poor match has an equally negative impact. Support surfaces redistribute the body’s weight and protect the skin’s tissue while providing for proper body alignment, comfort and, as part of a seating system, postural control during functional movement. These effects may conflict and require clinical decision making to strike a balance between protective and functional goals.
Ideally, an algorithm that incorporates an individual’s characteristics, conditions, environment, and preferences should be able to guide one to formulate a recommendation for an ideal, personalized support surface. The body of evidence is improving, but the research in this area has not yet produced a validated selection algorithm that matches specific products for any given situation. Some guidance is available for some conditions, but it’s not sufficient enough to replace good clinical decision-making and follow-up evaluations. Furthermore, existing clinical recommendations need to be updated regularly to reflect new research, technology, and treatment strategies as they become available.
Knowledge of a product’s composition and contents is a necessary part of the selection process. Although describing the materials and components of support surface technology may be informative, it isn’t always instructive. In terms of selecting a product, the information on the function or performance of the surface is most critical, regardless of composition. In a study by Krouskop and van Rijswijk, performance parameters were emphasized when identifying nine key support surface characteristics1 (Table 11-1).
Table 11-1 Support Surface Performance Parameters
Adapted from Krouskop, T., van Rijswijk, L. “Standardizing Performance-Based Criteria for Support Surfaces,” Ostomy/Wound Management 41(1):34–44, January-February 1995. Used with permission.
Although function- and performance-based categorization of seat cushions and support surfaces isn’t yet possible, the necessary data from clinical validation studies are being generated. Most significantly, standard tests for cushion and mattress performance have been and are being developed. These tests will provide researchers and industry with the tools needed to differentiate products based on relevant performance measures. This work is a global effort with participants from numerous countries. In the United States, the work is led by the Rehabilitation Engineering and Assistive Technology Association of North America’s (RESNA’s) Wheelchair and Related Seating Standards Committee.2 The mattress standards development effort is led by the National Pressure Ulcer Advisory Panel’s (NPUAP’s) Support Surface Standard Initiative (S3I) Committee,3 which is sanctioned by RESNA. The International Organization for Standardization (ISO) coordinates and publishes both cushion and mattress standards for worldwide use. On the clinical validation front, a recent study by Brienza et al.4 has shown that support surface technology can have a positive effect on preventing pressure ulcers. The best we can do now is to group the devices according to the technologies and materials used in their construction and relate the characteristics of these technologies to the factors believed to have significant effects on the prevention and healing of pressure ulcers.
Soft Tissue Biomechanics
Human soft tissue consists of a variety of macrostructures, including skin, fat, muscle, vessels, nerves, ligaments, and tendons. The relative amounts and arrangement of tissue macromolecules of the skin and supporting soft tissue are adapted to their specific functions and dictate their biomechanical properties.
In most connective tissue, fibroblasts secrete the macromolecules that make up the extracellular matrix. The matrix is made up of two main classes of macromolecules:
- polysaccharide chains of a class called glycosaminoglycans, which are usually found covalently linked to protein in the form of proteoglycans
- fibrous proteins that are either primarily structural (e.g., collagen and elastin) or primarily adhesive (e.g., fibronectin and laminin).
Glycosaminoglycans and proteoglycans form a highly hydrated, gel-like “ground substance” in which the proteins are embedded. The ground substance is analogous to glue that fills the lattice of collagen and elastin fibers, providing lubrication and shock absorbing qualities. The polysaccharide gel resists compressive forces on the matrix, while the collagen fibers along with elastin fibers provide tensile strength and resilience.
Tissue Mechanical Properties
In general, soft tissues are anisotropic, incompressible biosolid, biofluid mixtures.5 Obviously, soft tissue contains a substantial amount of fluid and, as a result, is largely incompressible. Moreover, tissue’s response to loading has both short- and long-term phases. In the short term, tissue deforms in response to load, but the fluid within tissue also moves slowly over time from areas of greater pressure to areas of lesser pressure. This time-dependent response to loading characterizes the viscoelastic behavior of tissue. This viscoelasticity influences its mechanical properties that include stress relaxation, creep, and hysteresis.6
These phenomena may be graphically represented as stress–strain curves. Stress is represented as the deforming force on the y axis, and the tissue strain (deformation) is plotted on the x axis. When soft tissue is suddenly deformed (strained) and the strain is thereafter kept constant, the corresponding stress induced in the tissue decreases over time. This phenomenon is known as stress relaxation (Fig. 11-1). Alternatively, creep describes the progressive tissue deformation that occurs over time when stress remains constant (Fig. 11-2). During cyclic loading, such as that produced by a dynamic, or alternating pressure mattress, the stress–strain relationship demonstrated during the loading phase is different from that of the recovery, or unloaded, portion of the cycle. This effect is known as hysteresis.
Figure 11-1. Stress relaxation phenomenon. The stress versus strain curve, shown below, illustrates the stress relaxation phenomenon. With the compression of tissue (strain) held constant, the force (stress) generated in the tissue as a result of that compression reduces over time. The degree of stress relaxation—that is, the amount of reduction in the holding force—can be determined by measuring the distance along the vertical axis between the time when the load is first applied to the time when it reaches steady state (downward sloping ends).
Figure 11-2. Creep phenomenon. Creep reflects the ability of tissue to resist deformation over time when the force causing the deformation remains constant. The creep phenomenon shown here indicates that the tissue progressively deforms over time without any additional force being applied. If creep were zero, the curve would be a flat line, indicating that deformation was constant over time.
Tissue Loading and Pressure Ulcer Formation
Body weight resting on bony prominences, such as the scapula, sacrum, greater trochanters, ischial tuberosities, and heels, can cause significant concentrations of pressure at the skin’s surface and in the underlying soft tissue. The pressure peaks and the pressure gradients surrounding these peaks can put the soft tissue at risk for breakdown. However, high pressure alone usually isn’t sufficient to cause a pressure ulcer. Research has clearly demonstrated that the damaging effects of pressure are related to both its magnitude and duration.7–11 Simply stated, tissue can withstand higher loads for shorter periods of time10 (Fig. 11-3).
Figure 11-3. Guidelines for sitting duration. This graph provides guidelines on sitting tolerance based on the magnitude of localized pressure. (Reprinted with permission from Reswick, J., Rogers, J. Experiences at Rancho Los Amigos Hospital with Devices and Techniques to Prevent Pressure Sores. Bedsore Biomechanics. London, UK: University Park Press, 1976.)
How pressure and shear ultimately cause pressure ulcers is complex and not entirely understood. At the cellular level, the three mechanisms most commonly cited describing how external forces result in tissue damage are (1) ischemia resulting from tissue deformation,9–11 (2) reperfusion injury following pressure relief,12–14 and (3) mechanical damage to cells caused by excessive deformation.15,16 Certainly, combinations of these factors may be significant. Several investigators have shown that in muscle tissue, external pressure causes deformation, not ischemia, which can be directly linked to injury.17,18 Elevated skin temperature is another factor that appears to be more important than previously believed.16,19,20
Use of Interface Pressure to Assess Tissue Loading
Interface pressure is the force per unit area that acts perpendicularly between the patient’s body and the support surface. Interface pressure can be measured by placing a pressure sensor between the patient’s skin and the support surface. This measurement approximates the pressure on the tissue. Single sensors have been used to measure local pressure over a single bony prominence; multiple sensors integrated into a mat may be used to “map” the entire body area in contact with the support surface10,18,21–26 (Fig. 11-4).
Figure 11-4. Seating, positioning, and support surfaces. Multiple sensors integrated into a mat may be used to “map” the entire body area that comes in contact with the support surface. This pressure map of a patient lying face up on a horizontal support surface shows varying degrees of pressure exerted by the patient’s hees, calves, thighs, buttocks, shoulders, and head.
Interface pressure has been used extensively as a tool for predicting the clinical effectiveness of various support surfaces and for comparing products. Many research efforts have been directed toward establishing an interface pressure threshold beyond which pressure ulcers would form. However, research has not identified a specific threshold at which loads can be deemed harmful across either subject populations or various tissue body sites. This is because a tissue’s loading tolerance is highly individualistic and varies according to its composition, condition, location, age, hydration, and metabolic state. As a result, interface pressure should not be used as a singular indication to use a particular support surface; however, it does have clinical utility to prevent pressure ulcers.22,27,28 Measuring interface pressures for a particular person permits comparison of tissue loading across surfaces or postures. This can assist clinicians to identify unacceptable situations and help determine acceptable clinical interventions individualized to the patient or client.29
Clinical Implications of Aging
The gross morphology of the soft tissues undergoes significant changes due to aging, including decreased moisture content and decreased elasticity manifested as rough, scaly skin with increased wrinkling and laxity. Dry, inelastic skin with larger, more irregular epidermal cells leads to decreased barrier function. These changes are reflected in the tissue biomechanical properties and have been associated with increased risk of tissue injury.
At the microscopic level, flattening of the dermal–epidermal junction (rete ridges) has been observed with the height of the dermal papillae declining by 55% from the third to ninth decade of life. As the space between the well-vascularized dermis and epidermis increases, several functional changes occur. Decreases have been reported in the area available for nutrient transfer, the number of cells within the stratum basale, and the skin’s resistance to shearing. A 30% to 50% decrease in epidermal turnover during the third to eighth decade of life has also been reported. This diminution in repair rate has been quantified as both decreased collagen deposition and diminished wound tensile strength. The loss of subcutaneous fat with aging decreases our protection from injury due to pressure and shearing forces between the bony prominences and the support surface. Moreover, decreased sensory perception increases the risk of injury by mechanical forces such as pressure. And, the stiffer, less elastic, drier nature of an elderly person’s skin can result in tissue that tears and bleeds more easily.
Support Surface Characteristics
Prevention of pressure ulcers is accomplished primarily by managing tissue loads. Support surfaces have been designed to reduce the effects of tissue loading by controlling the intensity and duration of pressure, shear, and friction. Also, attempts have been made to control the physical factors associated with increased risk through elimination of excess moisture and effective dissipation of heat.
Pressure Redistribution
Pressure redistribution is the ability of a support surface to distribute load over the contact areas of the human body. (This term replaces prior terminology of pressure reduction and pressure relief surfaces.3 The redistribution of pressure reduces the magnitude of pressure and shear forces, both of which can cause excessive tissue distortion and damage soft tissue. Pressure (stress) is defined as force per unit area; the pressure distribution is influenced by mechanical and physical characteristics of the support surface, mechanical properties of the body’s tissue, and weight distribution (posture).
Immersion
Immersion is defined as the depth of penetration into a support surface.3 The fundamental strategy for reducing pressure near a bony prominence is to allow the prominence to be immersed into the support surface. Immersion allows the pressure concentrated beneath a specific bony prominence to be spread out over the surrounding area, including other bony prominences. For example, when a person is sitting on a relatively hard cushion, a disproportionately large portion of his or her body weight is born by the tissue beneath the ischial tuberosities. On a softer surface, the ischial tuberosities and buttocks may immerse more deeply, even to the level of the greater trochanters. With greater immersion, the body weight divided by a greater surface area results in decreased average pressure. This definition of immersion doesn’t distinguish between immersion resulting from compression of the support surface and immersion resulting from the displacement of a support surface’s fluid components.
The potential for immersion depends on both the force–deformation characteristics of the cushion and its physical dimensions. For fluid-filled support surfaces, immersion depends on the thickness of the surface and the flexibility of the cover. For elastic and viscoelastic support surfaces, immersion depends on their stiffness and thickness. Consider how the thickness of a seat cushion might limit the potential for immersion. If the seat cushion is 1.5 inches (3.8 cm) in depth and the vertical distance between the ischial tuberosities and greater trochanters is 2 inches (5 cm), the potential for immersion isn’t enough to significantly unload the ischial tuberosities.
Envelopment
Envelopment is the ability of a support surface to conform to or mold around irregularities in the body.3 Good envelopment implies that the surface conforms to the body without a substantial increase in pressure. Examples of irregularities are creases in clothing, bedding, or seat covers, and protrusions of bony prominences. A fluid support medium would envelop perfectly. However, surface tension plays an important role in envelopment. For example, a fluid-filled support surface such as a water bed doesn’t envelop as well as water alone. The membrane containing the water has surface tension, which has a hammocking effect on irregularities of the interface. Poorly enveloping support surfaces may cause high local peak pressures, thereby potentially increasing the risk of tissue breakdown.
Pressure Gradient
Pressure gradient, also known as pressure differential, is defined as the change in pressure over a distance. Although various distances have been reported in the literature, pressure gradient is expressed most commonly as a change in millimeters of mercury (mm Hg) per square centimeter or square inch. When the pressure across a surface is plotted on a graph, the slope of the curve is the pressure gradient. Because the skin and other soft tissue at risk for breakdown consist of a mixture of interstitial fluid and ground substance into which structural elements are embedded, a pressure differential between adjacent regions will result in a slowing of the flow of the tissue’s fluid elements from a region of high pressure to one of lesser pressure. This flow is analogous to the movement produced when one compresses the surface of a bucket of wet sand with one’s hand.
Several investigators have hypothesized that the flow of interstitial fluid caused by pressure gradients is the primary factor in the development of pressure ulcers.30,31 The flow of ground substance and interstitial fluids from an area of high pressure is believed to increase the likelihood of intercellular contact, resulting in cellular ruptures.30–32 This theory is consistent with the classic experimental results of several researchers showing a relationship between duration of pressure application and the magnitude of pressure that results in the formation of a pressure ulcer.10,30
Pressure gradient is intimately linked to pressure and is affected by immersion and envelopment in a similar manner. Under certain circumstances, it’s possible to have pressure gradients without high pressure, and vice versa. For example, the boundary of the contact area on a support surface necessarily demonstrates a significant pressure gradient where the pressure magnitude transitions from zero outside the area of support to a nonzero value in the supported region. Despite these significant gradients, boundary areas are typically areas of lower risk for pressure ulcer development, suggesting that pressure gradient only becomes an important factor when combined with high pressure. Further research is needed to test and investigate this hypothesis.
Shear and Friction Reduction
The term shear can be used to reference shear stress or shear strain, and that can be a source of confusion. Shear stress refers to forces applied tangentially over an area of tissue that induce deformation (shear strain). Within tissue, stress and strain are ever-present because of the effects of gravity. Shear strain is a part of the overall tissue deformation that occurs on all supporting surfaces.33 For example, when the head of a bed is raised or lowered, if the skin over the sacrum does not slide along the surface of the bed, or the bed does not absorb the resulting shear force by deforming in the horizontal direction, the effect will be a shearing of the soft tissue between the sacrum and the support surface. In engineering terms, the resulting shearing or deformation of the soft tissue would be referred to as “shear strain.” The characteristics of the support surface affecting this potentially harmful situation are the coefficient of friction of the surface and the ability of the surface to deform horizontally.
Friction is the resistance to motion of two surfaces in contact. Friction is a force that is dependent on the characteristics of the two interface surfaces and the contact force between them. With respect to support surfaces and tissue, friction is the force that resists sliding, and this force can induce deformation, including shear strain in the tissue.34–36
Shear strain and frictional forces can be illustrated using the elevation of the head of the bed. When raising the head of the bed, gravity will encourage the body to slide downward to the foot of the bed. This sliding is resisted by the friction between the body and the bed linens. However, even if the skin over the sacrum does not slide along the surface of the bed, deformation of the tissue (shear strain) can occur if the bony sacrum moves with respect to the overlying skin.
Friction cannot be fully eliminated in support surfaces. In fact, friction is needed to maintain stability whether sitting, standing, or lying. Therefore, the clinical goal is to understand friction in the context or minimizing its potential damaging influences. For example, wet skin has a higher coefficient of friction than does dry skin34–36 and, as will be discussed below, is more susceptible to damage. Pulling someone across a bed sheet during transfer exposes the tissue to significant friction. Unweighting the person before sliding will greatly minimize the friction, because, as mentioned above, friction is dependent on the force between the contact surfaces (Fig. 11-5).
Figure 11-5. Friction and shear forces. This illustration shows the friction and shear forces acting on a person lying in bed.
Some support surface technologies protect the skin from shear better than do others. Some surfaces use a low friction interface in an attempt to minimize shear strain. Others have a low horizontal stiffness that deflects under tangential forces to minimize shear strain. Shear strain as a contributing factor for pressure ulcers is difficult to study but has been the focus of several articles.37–39 Shear is a topic of international discussion and a task force is looking at ways to measure shear stress and strain and quantify its effects on skin.
Temperature Control
One of the extrinsic factors in pressure ulcer development, temperature, has not been definitively investigated. However, some clinical trials have shown that the application of repetitive surface loading alone induces an elevated skin temperature of 41°F or greater.40 In addition, peak skin temperatures have been found to be proportional to the magnitude and duration of the applied pressure.20,41,42 The conclusions of research vary depending upon the amount and duration of pressure that’s simultaneously applied with varying temperatures.20,41,42
In addition, higher ambient temperatures have been shown to cause an increase in tissue metabolism and oxygen consumption on the order of 10% for every 1.8°F increment.43 Thus, patients with compromised tissue already at risk for pressure ulcers may have increased demands for oxygen in excess of their metabolic capabilities. Any increase in temperature in combination with pressure is believed to increase the susceptibility of the tissue to injury either from ischemia or reperfusion injury when the pressure is relieved.44
Also, increased temperature causes an exponential increase in blood perfusion, which has been associated with either an increase in core body temperature or in local skin temperature.45,46 For example, in a study of operatively acquired pressure ulcers, the single greatest predictor of pressure ulcer development was the use of a warming blanket under the patient.47 These findings clearly indicate the need for additional studies to definitively determine the effects of skin temperature modulation on the development of pressure ulcers. Therefore, when choosing the right support surface for the patient, its heat transfer rate is an objective performance measurement related to its ability to control temperature effects.
Animal studies have found that increased skin temperature is related to greater tissue necrosis at all layers, and a shorter period of damaging conditions is required to cause the same amount of tissue damage when the skin temperature is higher. Kokate et al.48 first investigated increased skin temperature in a swine model. They applied 100 mm Hg of pressure for 5 hours on the back of swine at four different temperatures: 25°C, 30°C, 35°C, and 45°C. Histological examination revealed that there was severe necrosis in more layers of the soft tissue with higher temperatures. Iaizzo49 adopted the same animal model and evaluated various combinations of skin temperature and duration of localized pressure. The histological assessment showed that temperatures as high as 45°C could cause tissue damage under localized pressure for as short as 2 hours, while sites with 25°C showed no indication of tissue damage for any duration. The protective effects of cooling the skin has also been demonstrated in humans. In particular, Tzen et al.50 showed that temperature control reduces harmful effects of ischemia and demonstrated the effectiveness of local cooling for enhancing tissue ischemia tolerance in people with spinal cord injury51 Lachenbruch showed that temperature is a more important factor when compared to shear relative to the intensity of the reactive hyperemic response.37 The implication of this work is that support surfaces that control the skin temperature in the range of 25°C to 27°C facilitates pressure ulcer prevention.
Moisture Control
Moisture is another key extrinsic factor in pressure ulcer development. The sources of skin moisture that may predispose the skin to breakdown include perspiration, urine, feces, and fistula or wound drainage. Excessive moisture may lead to maceration of the skin.52 Increases may be due to the slight increase in friction that occurs with light sweating53 or to the increase in bacterial load resulting when alkaline sources of moisture neutralize the protection provided by the normal acid mantle of the skin.
The detrimental effect of an increase in moisture adjacent to the skin has been demonstrated by tensile tests on excised skin strips in a controlled humidity environment. In a study by Wildnauer et al.,54 the tensile strength of the strips decreased by 75% with an increase in relative humidity from 10% to 98%. Skin with such reduced strength may be more prone to mechanical damage from shear stress and could easily be abraded.
Materials and Components Used in Support Surface Systems
The components and materials described here are the most commonly used in support surface systems and may be used alone or in combination. They include foam, gel and gel pads, fluid-filled bladders, viscous fluid, and elastomers.
Foam
Foam may be elastic or viscoelastic and may be comprised of open or closed cells. Open-cell foam is defined as a permeable structure in which the cells are interconnected and allows air or fluids to pass between them. Closed-cell foam is a nonpermeable structure comprised of isolated cells that are not connected. As a result, closed-cell foam tends to be stiffer than open-cell foam simply because it does not compress as readily due to trapped air in which there’s a barrier between cells, preventing gases or liquids from passing through the foam.
Elastic Foam
Elastic foam is a type of porous polymer material that conforms in proportion to the applied load.3 Consequently, greater loads result in predictably greater deformations, and vice versa. If time is a factor in the load versus deformation characteristic, the response is considered to be viscoelastic, which is discussed separately. The response of support surfaces made from resilient foam is predominately elastic. Elastic foam is resilient, meaning that it returns to its nominal shape or thickness once load is removed. One indication of foam fatigue is an inability to return to its original unloaded condition.
Foam is characterized by density and stiffness. Density reflects the weight of the foam and stiffness reflects the force required to deflect the foam a given amount. Foam’s stiffness and thickness impact its ability to immerse and envelop; soft foams permit greater immersion and envelopment compared to stiffer foams, but must be thicker to avoid bottoming out. Foam seat cushions are typically contoured to improve their performance.
Many foam products consist of layers of varying stiffness. This laminar design is used to optimize the performance of different foams and materials. In a laminar foam product, the softer foam is on top, thereby allowing immersion and envelopment of the body. Foam used in seat cushions can also be contoured to provide a better match between the buttocks, and the cushion increases the contact area and immersion, thereby reducing average pressure and pressure peaks2 (Fig. 11-6). Foam is also used in combination with other materials such as elastomers, air, and fluid. Typically, a foam base offers postural stability while the elastomer, air, or fluid provides an enveloping interface. An ideal combination of characteristics for an elastic support surface is resistance that adjusts to the magnitude of compressive forces.55,56 The support surface should be soft and thick enough to provide good immersion and envelopment but have high enough compression resistance and thickness to fully support the load without approaching maximum compression. Near maximum compression, the foam becomes much more rigid and provides too high a reactive force and a concentration of high pressure. This effect is sometimes referred to as “bottoming out.” Over time and with extended use, foam degrades and loses its resilience. This decreased ability can result in higher interface pressures if it causes bottoming out. Krouskop55 estimated that in approximately 3 years, a foam mattress wears out.
Figure 11-6. Elastic foam seat cushions. These photos show four different types of elastic foam seat cushions: (A) Flat. (B) Coutoured. (C) Cut-out. (D) Segmented.
Foam tends to increase skin temperature because its materials and the air they entrap are poor heat conductors. Patient movement can also increase heat transfer rates. Mean temperature increases of 6.1°F (3.4°C) and a 10.4% increase in moisture at the skin surface have been recorded on foam products after 1 hour of contact.57
Viscoelastic Foam
Viscoelastic foam is a special type of open cell foam that compresses under load like elastic foam but has other unique qualities. Viscoelastic foam is sometimes referred to as “memory foam” because it contours to the body and its elastic response diminishes over time. This is a characteristic of stress relaxation and allows viscoelastic foam to maintain its deformed shape for a few moments before returning to its original unloaded shape (ergo, “memory foam”). Viscoelastic foam comes in a variety of stiffness so it can allow immersion similar to a similar elastic foam. The difference lies in its enveloping quality. Because of the stress relaxation, viscoelastic foam envelops better than does elastic foam. However, viscoelastic foam also exhibits creep, meaning that, over time, it will continue to deform under the same load. Furthermore, viscoelastic foam products can be temperature sensitive and become softer at temperatures near that of the human body. Mean temperature increases of 5°F have been reported for viscoelastic foam.57 This characteristic allows the body to immerse more into the foam and can improve contact area and lower interface pressure. However, both creep and temperature sensitivity can lead to a greater potential of bottoming out. So, as with elastic foam, viscoelastic foam must be chosen according to the specific needs of the patient for both seating and mattress applications (Fig. 11-7).
Figure 11-7. Viscoelastic gel seat cushion.
Elastomers
Soft elastomers are rubber-like materials and are sometimes referred to as solid gels. For use in support surfaces, elastomers are often used on top of other materials because they tend to be heavy.
Elastomers have a high heat capacity and conductivity. This is evidenced by the many elastomeric products designed to be heated or frozen to provide relief to a sore body part. When used as a support surface, an elastomer will absorb body heat so the interface temperature will not rise as quickly.57 This allows elastomers to feel cooler once they are sat upon. Overtime, the elastomer will eventually reach capacity and the interface temperature will rise, unless the body is repositioned—via pressure reliefs or other repositioning—to allow the heat to dissipate. Depending on the elastomer and cushion cover, moisture at the interface can also rise.44
Fluid-Filled Bladders and Compartments
Fluid-filled products may consist of small or large chambers filled with air, water, or other viscous fluid materials, such as silicon elastomer, silicon, or polyvinyl. The fluid flows from chamber to chamber or within a single chamber in response to movement and requires no supplemental power. The term “air-flotation” is sometimes used to describe interconnected multichamber surfaces (Fig. 11-8).
Figure 11-8. Fluid-filled products. As the photos demonstrate, fluid-filled products come in a variety of forms: (A) Cushion. (B) Mattress.
Practice Point
Be careful to maintain the correct levels of inflation in air cushions to achieve optimal pressure reduction. Underinflation causes bottoming out, and overinflation increases the interface pressure. For surfaces with viscous fluid bladders, such as seat cushions, it’s important to monitor the distribution of viscous fluid and manually move it back to the areas under bony prominences if it has moved away from these areas.
Most fluid-filled products permit a high degree of immersion, allowing the body to sink into the surface. The surface conforms to bony prominences, effectively increasing the surface pressure distribution area and lowering the interface pressure by transferring the pressure to adjacent areas. These products are capable of achieving small-to-modest deformations without large restoring shear forces. In a direct comparison of interface pressures with air-fluidized and low-air-loss beds, the RIK mattress was shown to relieve pressure as effectively as the air-fluidized and low-air-loss surfaces used in the study.58
Skin temperature is affected by the heat capacity and thermal conductivity of the fluid material contained in the support device. Air has a low heat capacity, whereas water has a high heat capacity. The viscous material used in the RIK mattress also has a high heat capacity, and skin interface temperature will not rise as rapidly compared to foam cushions.58
Given the large variety of materials used as fluid bladders, it’s difficult to generalize on the moisture control characteristics of these products. However, the insulating effects of rubber and plastic used in some fluid-filled products have been shown to increase the relative humidity due to perspiration.57
Features of Support Surfaces
The features covered in this section can be used alone or in combination with other features. They include air-fluidized, low-air-loss, alternating pressure, and lateral rotation products.
Air-Fluidized
A support surface with an air-fluidized feature provides pressure redistribution via a fluid-like medium created by forcing air through beads as characterized by immersion and envelopment.4 These beds were originally developed in the late 1960s for use with burn patients. These products consist of granular materials such as silicon beads encased in a polyester or Gore-Tex sheeting. The granular material takes on the characteristics of a fluid when pressurized air is forced up through them. In some models, the fluidization feature is variable, permitting individualization based on the patient’s needs. These products aid in the reduction of evaporative water loss. Feces and other body fluids flow freely through the sheet; to prevent bacteriologic contamination, the bed must be pressurized at all times, and the sheet must be properly disinfected after use by each patient and at least once per week with long-term use by a single patient.59,60
Air-fluidized beds use fluid technology to decrease pressure through the principle of immersion while simultaneously reducing shear. Air-fluidized products permit the highest degree of immersion currently available among support surfaces. The surface conforms to bony prominences by permitting deep immersion into the surface—almost two-thirds of the body may be immersed.61 The immersion effectively lowers the interface pressure by increasing the surface pressure distribution area. The greater deformations possible with this technology enable the transfer of pressure to adjacent body areas and other bony prominences. Envelopment and shear force are minimized. A loose but tightly woven polyester or Gore-Tex cover sheet is used to reduce surface tension. Low surface tension enhances envelopment and minimizes shear forces.
The pressurized air in these products is generally warmed to a temperature level of 82.4°F to 95°F (28°C to 35°C); however, warming may be beneficial or harmful depending on a patient’s needs. For example, heat may be harmful to patients with multiple sclerosis, but beneficial for patients in pain. The beneficial effects must be balanced against the increasing metabolic demands of the tissue.
The high degree of moisture–vapor permeability of the air-fluidized system is effective in managing body fluids (Fig. 11-9).
Figure 11-9.Air-fluidized (A) and low-air-loss (B) beds.
Practice Point
Air-fluidized beds are advantageous for burn patients due to their effectiveness in managing body fluids.
Low-Air-Loss
Low-air-loss is a feature of a support surface that provides a flow of air to assist in managing the heat and humidity (microclimate) of the skin3 Low-air-loss systems use a series of connected, air-filled cushions or compartments, which are inflated to specific pressures to provide loading resistance based on the patient’s height, weight, and distribution of body weight. An air pump circulates a continuous flow of air through the device, replacing air lost through the surface’s pores. The inflation pressures of the cushions vary with the patient’s weight distribution; some systems have individually adjustable sections for the head, trunk, pelvic, or foot areas. As with other fluid-filled surfaces, the temperature of the skin is affected by the specific heat of the fluid material. However, the constant air circulation and evaporation tend to keep the skin from overheating.
In low-air-loss systems, the patient lies on a loose-fitting, waterproof cover placed over the cushions. The waterproof covers are designed to let air pass through the pores of the fabric and are usually made of a special nylon or polytetrafluoroethylene fabric with high moisture–vapor permeability. Manufacturers have addressed the problem of skin dehydration by altering the number, size, and configuration of the pores in the covers.50,61 The material is very smooth, with a low coefficient of friction; in addition, it’s impermeable to bacteria and easy to clean.61 Low-air-loss devices have been shown to prevent buildup of moisture and subsequent skin maceration.62
Alternating Pressure
Alternating pressure is a feature of a support surface that provides pressure redistribution via cyclic changes in loading and unloading as characterized by frequency, duration, amplitude, and rate of change parameters over the “active area” of the surface.3 These systems contain air-filled chambers or cylinders arranged lengthwise or in various other patterns. Air or fluid is pumped into the chambers at periodic intervals to inflate and deflate the chambers in opposite phases, thereby changing the pressure distribution. The frequency of the alternating pressure feature can have an effect on its use. For example, very short peak inflation, and the cycling time appear to have a dramatic effect on increasing lymphatic flow.63
Rather than increasing the surface area for distribution through immersion and envelopment, alternating-pressure devices distribute the pressure by shifting the body weight to a different surface contact area. This may increase the interface pressure of that area during the inflation phase.
Alternating pressure technology has the same potential as any other fluid-filled support surface to influence temperature at the interface, thus care must be taken to maintain the correct levels of inflation. The skin moisture control and temperature control characteristics of alternating-pressure surfaces also depend on the characteristics of the cover and supporting material (Fig. 11-10).
Figure 11-10. Alternating-pressure integrated cushions. The illustration shows the characteristics of alternating-pressure integrated cushions.
Lateral Rotation
Lateral rotation is a feature of a support surface that moves the patient in a regular pattern around a longitudinal axis as characterized by degree of patient turn, duration, and frequency.3 Although these devices have been used for several decades for other medical purposes, such as pulmonary therapy, research is conflicting on their use for pressure ulcer treatment. Lateral rotation may be continuous (i.e., on an automatic timed cycle) or manual, where the bed is rotated and locked in that position.64 The therapy works by positioning the patient in such a way so that one lung is higher than the other to prevent pneumonia. This therapy is not intended for patients with cervical or spinal fracture, intracranial pressure instability, or long bone fractures.
According to the NPUAP, “Whenever lateral rotation features are used, the risk for shear injury exists. Shear force tangentially strains the skin (through stretching) and interrupts blood flow of the skin. Unless the individual is properly positioned and bolstered, shearing can occur with every rotation, causing a new ulcer or worsening existing ulcers”65 (Table 11-2).
Table 11-2 Recommendations for Lateral Rotation
Adapted from Emily Haesler, ed. National Pressure Ulcer Advisory Panel and European Pressure Ulcer Advisory Panel, Pan Pacific Pressure Injury Alliance. Prevention and Treatment of Pressure Ulcers: Clinical Practice Guideline. Osborne Park, Western Australia: Cambridge Media, with permission.
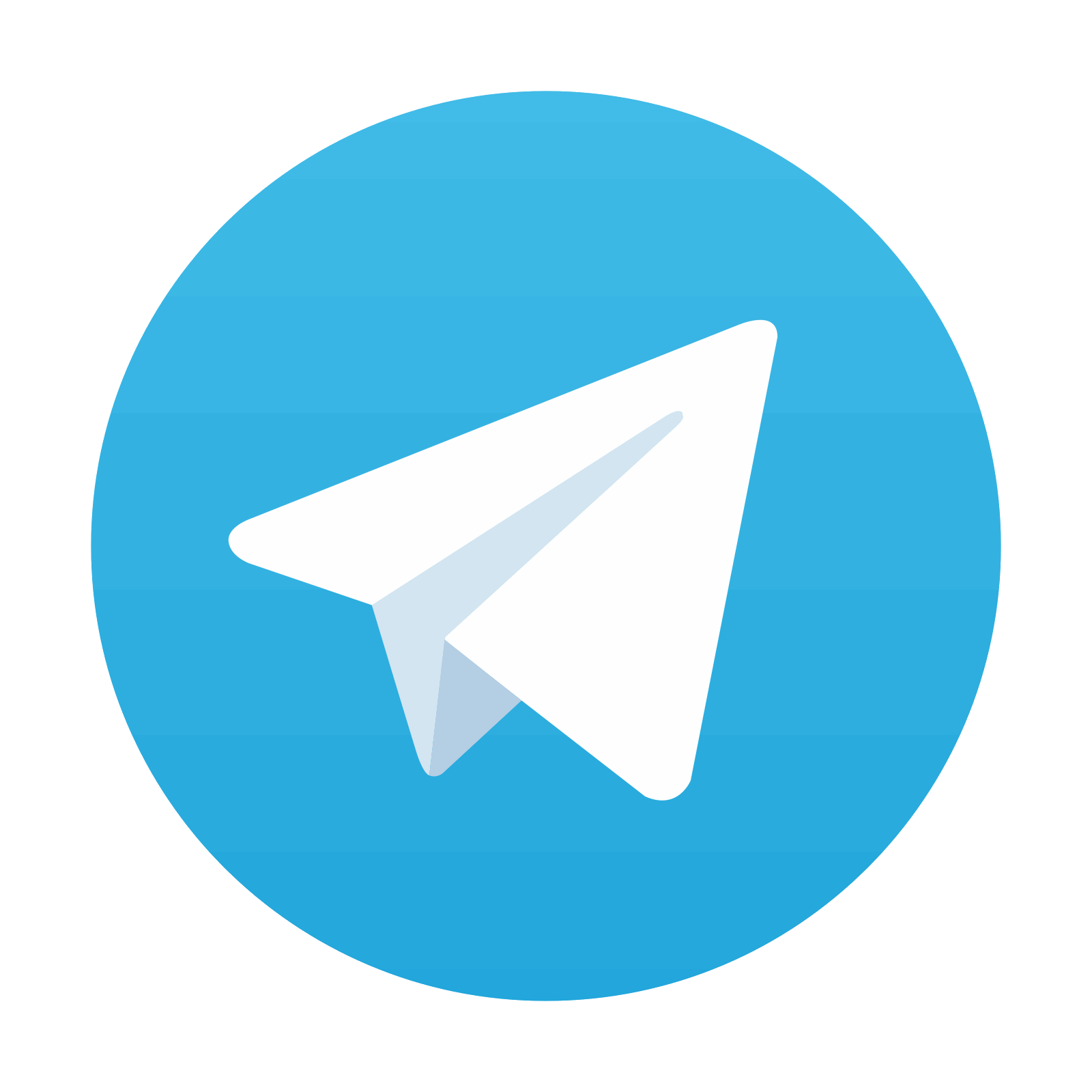
Stay updated, free articles. Join our Telegram channel

Full access? Get Clinical Tree
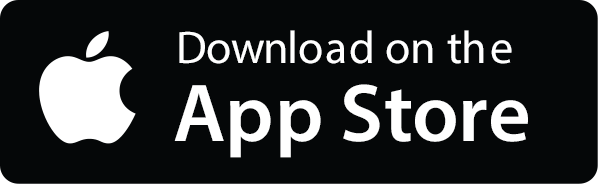
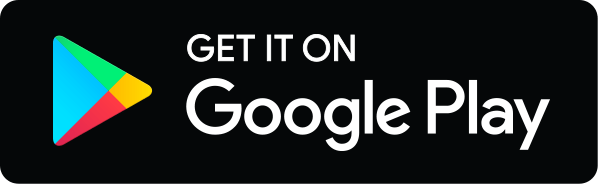