Chapter 8 Endocrinology
Basic concepts
1 What is the cellular mechanism of action of the steroid hormones?
Steroid hormones are lipophilic. Therefore, they diffuse across the plasma membrane and form complexes with cytosolic or nuclear receptors; the bound complexes then activate transcription of various genes. Because steroid hormones rely on the intermediary process of gene expression and protein translation, it can take hours to days for their effects to manifest. Examples of steroid hormones are testosterone, estrogen, progesterone, cortisol, and aldosterone. Cholesterol is the precursor to all steroid hormones. Although thyroid hormone is not a steroid hormone, it uses the same cellular mechanism as the steroids (Fig. 8-1).
2 What is the cellular mechanism of action of the peptide hormones and the catecholamines?
The peptide hormones and catecholamines are not highly lipid-diffusible and thus cannot cross the plasma membrane. They bind to cell surface receptors (see Fig. 8-1), which initiate a variety of biochemical events, including activation or inhibition of enzymes, alteration of membrane proteins, and mediation of cellular trafficking. These processes can occur within seconds to minutes. Nevertheless, the peptide hormones can stimulate gene expression as well, and this effect is delayed as it is with the steroid hormones. Examples of peptide hormones are insulin, parathyroid hormone (PTH), vasopressin (antidiuretic hormone), and oxytocin. Table 8-1 shows a comparison of polypeptide and steroid hormones.
3 Why is the total serum hormone level not an accurate reflection of hormone activity?
Note: Free hormone is in equilibrium with bound hormone:
4 How does a hormone’s binding to the same type of receptor have different effects in different cell types?
5 What are the four primary classes of membrane-spanning receptors to which peptide hormones bind?
The four primary classes of membrane-spanning receptors to which peptide hormones bind are (1) tyrosine and serine kinase receptors, (2) receptor-linked kinases, (3) G protein–coupled receptors, and (4) ligand-gated ion channels (Fig. 8-2 and Table 8-2). As a gross simplification, the “prototypical” agonists for these receptor types can be considered to be growth factors, growth hormones (GHs), peptide hormones, and neurotransmitters, respectively.
Table 8-2 Classes of Receptors Used by Various Hormones
Receptor Class | Hormones and Related Substances |
---|---|
cAMP | LH, FSH, ACTH, TSH, PTH, hCG, CRH, glucagon |
cGMP | NO, ANP |
IP3 | GnRH, GHRH, oxytocin, TRH |
Steroid receptor | Estrogen, testosterone, glucocorticoids, vitamin D, aldosterone, progesterone, T3/T4 |
Tyrosine kinase | Insulin, growth factors (e.g., IGF, PDGF), GH, prolactin |
ACTH, adrenocorticotropic hormone; ANP, atrial natriuretic peptide; cAMP, cyclic adenosine monophosphate; cGMP, cyclic guanosine monophosphate; CRH, corticotropin-releasing hormone; FSH, follicle-stimulating hormone; GH, growth hormone; GHRH, growth hormone–releasing hormone; GnRH, gonadotropin-releasing hormone; hCG, human chorionic gonadotropin; IGF, insulin-like growth factor; IP3, inositol triphosphate; NO, nitric oxide; PDGF, platelet-derived growth factor; PTH, parathyroid hormone; T3, triiodothyronine; T4, thyroxine; TRH, thyrotropin-releasing hormone; TSH, thyroid-stimulating hormone.
6 How do the tyrosine kinase receptors transduce their messages?
As depicted in Figure 8-3, binding of peptide hormone to the extracellular domain of the receptor initiates a signal transduction cascade by promoting autophosphorylation of the kinase receptor and subsequent phosphorylation of downstream target proteins, thereby activating or inhibiting these proteins.
8 How do the G proteins transduce their signals?
Binding of hormone/agonist to G protein–coupled receptors causes an αβγ subunit complex to exchange guanosine diphosphate (GDP) for guanosine triphosphate (GTP). Once GTP is bound to the subunit complex, it dissociates into the α subunit and a separate βγ subunit. These dissociated subunits then activate or inhibit enzymes (adenylate cyclase, phospholipase) and ion channels (Ca2+ channels) (Fig. 8-4).
G proteins are a five-star topic on boards. Study the pathways for Gs, Gi, and Gq signaling depicted in Figure 8-4. You should know the details of these pathways, including the predominant cellular changes that occur with activation of each protein (e.g., cyclic adenosine monophosphate [cAMP] increase with Gs activation, intracellular [Ca2+] increase with Gq activation).
9 How do endocrine, paracrine, and autocrine mechanisms of cell communication differ?
Endocrine secretions (i.e., hormones) affect their target organs at considerable distance from their site of secretion, so they must be carried by the bloodstream. Paracrine secretions act locally on adjacent cells and tissues. Paracrine communication seems particularly important in endocrine tissues, such as the pancreatic islets, where constant communication between adjacent cells (e.g., α and δ cells) is critical for optimal functioning. Autocrine secretions are secretions from a cell that bind to receptors on that same cell and exert regulatory actions on that cell. Neuroendocrine secretions involve the secretion of peptides into the blood from specialized neurons (hence the term neuroendocrine). Hypothalamic peptides released into the blood from the terminal boutons of axons located in the posterior pituitary are one example of this mechanism of regulation (Fig. 8-5).
10 Describe the concept of negative feedback. What is a feedback loop?
Hormone synthesis and release are governed at multiple levels. Hormone synthesis/release from an organ of interest typically involves regulation by a pituitary hormone, which itself is regulated by a hypothalamic hormone. This general pathway structure is commonly referred to as a hypothalamic-pituitary-(organ) axis (e.g., HPO axis refers to the ovary, HPA axis refers to the adrenal gland). Negative feedback occurs when a product downstream of an axis inhibits production of a reactant by which it is regulated; for example, thyroid hormone inhibition of thyroid-stimulating hormone (TSH). These relationships are often depicted using feedback loops. An example of the thyroid hormone feedback loop is depicted in Figure 8-6.
2 Why is the pituitary more susceptible to infarction in postpartum hemorrhage than in hemorrhagic shock unrelated to pregnancy?
3 Why is the posterior pituitary typically spared in Sheehan’s syndrome?
The posterior pituitary (neurohypophysis) differs in embryologic origin from the anterior pituitary and therefore has a different blood supply. Remember, the embryologic origin of the anterior pituitary is Rathke’s pouch (an endodermal evagination from the roof of the mouth), whereas the posterior pituitary is derived from a ventral outgrowth from the primitive hypothalamus. Table 8-3 shows a review of the functions of the posterior pituitary hormones.
4 Secretion of which pituitary hormones may be affected in this woman?
Depending on the extent of the infarction, all the anterior pituitary hormones may be affected (Fig. 8-7). This patient is unable to lactate, which is consistent with decreased prolactin secretion. (Whenever you see a patient who is unable to lactate shortly after delivery, consider Sheehan’s syndrome!) Note that her axillary and pubic hair is sparse, which is consistent with decreased gonadotropin (FSH, LH) secretion. The patient is also weak and lethargic, which is consistent with hypocortisolism due to decreased ACTH secretion.
5 Why may hypothalamic releasing hormone secretion increase because of an infarction of the anterior pituitary?
The loss of pituitary hormone secretion will decrease negative feedback on the hypothalamic hormones both from low pituitary hormone levels and from low target organ hormone production. Table 8-4 reviews the hypothalamic releasing hormones.
6 Why wouldn’t hypothalamic dopamine secretion be elevated from an anterior pituitary infarction?
Summary Box: Sheehan’s Syndrome
Postpartum necrosis of the anterior pituitary is termed Sheehan’s syndrome.
Anterior pituitary hormones include follicle-stimulating hormone (FSH), luteinizing hormone (LH), adrenocorticotropic hormone (ACTH), thyroid-stimulating hormone (TSH), growth hormone (GH), and prolactin. (Remember FLAT P(i)G and disregard the i.)
The posterior pituitary secretes vasopressin (antidiuretic hormone [ADH]) and oxytocin.
The embryologic origin of the anterior pituitary is Rathke’s pouch.
The posterior pituitary is derived from a ventral outgrowth of the primitive hypothalamus.
1 What is the diagnosis?
Prolactinoma is a pituitary adenoma caused by abnormal proliferation of lactotrophs; it is the most common type of hypersecreting pituitary adenoma. Note that the pituitary is situated in the sella turcica. Table 8-5 reviews pituitary adenomas.
Table 8-5 Disorders Caused by the Deficiency or Excess of Anterior Pituitary Hormones
Hormone(s) | Deficiency | Excess |
---|---|---|
GH | Dwarfism in children or adults GH deficiency syndrome | Gigantism in children, acromegaly in adults |
LH and FSH | Gonadal insufficiency (decreased sex steroids) | Extremely rare but causes infertility |
ACTH | Adrenocortical insufficiency (decreased cortisol and adrenal androgens) | Cushing disease (increased cortisol and adrenal androgens) |
TSH | Hypothyroidism (decreased thyroid hormones) | Extremely rare but causes hyperthyroidism (increased thyroid hormones) |
Prolactin | Hypoprolactinemia (failure in postpartum lactation) | Hyperprolactinemia (impotence in males, amenorrhea in females, and decreased libido) |
ACTH, adrenocorticotropic hormone; FSH, follicle-stimulating hormone; GH, growth hormone; LH, luteinizing hormone; TSH, thyroid-stimulating hormone.
From Meszaros JG, Olson ER, Naugle JE, et al: Crash Course: Endocrine and Reproductive Systems. Philadelphia, Mosby, 2006.
2 What are the normal physiologic functions of prolactin preceding, during, and following pregnancy?
Preceding pregnancy: Prolactin levels are normal due to tonic hypothalamic inhibition via dopamine and to the absence of stimulatory factors such as suckling or high estrogen. It has numerous physiologic functions in countless organ systems in the nonpregnant woman, none of which are high yield for boards.
During pregnancy: Prolactin levels are high secondary to high estrogen levels (secreted by the placenta), which stimulate breast maturation and lactogenesis. However, actual lactation is prevented by high estrogen and progesterone (which antagonize actions of prolactin on the breast).
Following pregnancy: Estrogen levels drop, and prolactin levels also will drop unless stimulation by suckling occurs; levels increase and lactation occurs with suckling stimulation. It is important for you to know that prolactin will also inhibit GnRH secretion, often (but not always!) resulting in anovulatory infertility while nursing.
Figure 8-8 outlines the other physiologic functions of prolactin.
3 Why does this patient have galactorrhea, whereas pregnant women with similar levels of serum prolactin generally do not have this problem?
5 How does elevated prolactin prevent pregnancy (i.e., what is the mechanism of infertility and amenorrhea in this patient)?
6 Why is asking about a history of schizophrenia and use of antipsychotic medications a relevant question in the diagnostic workup of this patient?
7 What is the mechanistic basis for using bromocriptine (used to treat Parkinson’s disease) in the treatment of a prolactinoma?
8 How can head trauma with a severed pituitary stalk cause a similar increase in prolactin (assuming the anterior pituitary itself was not damaged)?
9 Why does hypothyroidism need to be considered in the evaluation of hyperprolactinemia?
Summary Box: Prolactinoma and Hyperprolactinemia
The pituitary is located in the sella turcica.
Prolactinoma is the most common type of hypersecreting pituitary adenoma.
Hyperprolactinemia can be caused by a prolactinoma, antipsychotics (via inhibition of hypothalamic dopamine secretion), hypothyroidism (via increased thyrotropin-releasing hormone [TRH]), and breast feeding or excessive nipple stimulation.
The increase in prolactin secretion that occurs with suckling is important in allowing for lactation. It can also inhibit gonadotropin-releasing hormone (GnRH) secretion and cause an anovulatory infertility, explaining why nursing women may have difficulty becoming pregnant.
In women, in addition to causing infertility, hyperprolactinemia can cause galactorrhea. It can also cause malaise and depression.
In men, hyperprolactinemia can cause impotence and lack of libido but only rarely will cause galactorrhea.
Treatment for prolactinoma includes the use of dopamine agonists (for small adenomas) or, less commonly, surgical resection (for larger adenomas).
4 Given the normal physiology of growth hormone, how can we explain this patient’s presentation?
Note: Most of the metabolic effects of GH are mediated through IGF-1, which is secreted by the liver. IGF-1 acts on bone to stimulate linear and lateral bone growth. IGF-1 also promotes the growth of cartilage and other soft tissues (Fig. 8-9).
6 If this patient developed a growth hormone–secreting tumor in his early teens, how might the clinical manifestations differ?
7 What growth abnormality results from deficient secretion of growth hormone during the growing years?
Pituitary dwarfism is the most common result of this deficiency.
Summary Box: Acromegaly and Physiology of Growth Hormone
A growth hormone (GH)-hypersecreting pituitary adenoma can result in gigantism and acromegaly depending on the age at onset (i.e., whether the epiphyseal plates have fused).
GH promotes anabolic actions on skeletal muscle and bone, stimulates lipolysis in adipose tissue, and stimulates hepatic gluconeogenesis. It generally antagonizes the actions of insulin.
The metabolic actions of GH are largely mediated by insulin-like growth factor-1 (IGF-1).
GH secretion is stimulated by hypoglycemia, stress, and sleep. It is inhibited by somatostatin, glucose, and IGF-1. Glucose administration does not inhibit GH secretion in acromegaly.
Patients with acromegaly may present with prominent jaw (macrognathia), coarsening of facial features, hyperglycemia or frank diabetes, and organomegaly.
2 What is the cause of the hypercortisolism in this patient?
Cushing disease is due to the elevated cortisol and ACTH. One would expect suppressed ACTH levels if the adrenal glands were hypersecreting cortisol. Paraneoplastic ACTH secretion (e.g., small cell carcinoma) is suggested by the increased ACTH level, but ectopic ACTH secretion occurs independently of the HPA (hypothalamic-pituitary-adrenal) axis and does not normally suppress in response to glucocorticoids such as dexamethasone. In contrast, pituitary adenomas, which are well differentiated, typically retain some feedback responsiveness to glucocorticoids; thus, ACTH secretion may not suppress with low-dose dexamethasone but typically will with high-dose dexamethasone (Table 8-6).
7 How is hypercortisolism contributing to hyperglycemia in this patient?
Cortisol promotes hyperglycemia by stimulating hepatic gluconeogenesis and inhibiting the peripheral utilization of glucose (similar to GH). The increase in gluconeogenesis is due to stimulation of the synthesis of gluconeogenic enzymes by cortisol and also to greater mobilization of amino acids from skeletal muscle to participate in gluconeogenesis (hence the muscle wasting) (Fig. 8-10).
11 What are the three layers of the adrenal cortex, and which one is responsible for the excess production of cortisol in this patient?
Just think of glomerular filtration rate (GFR) for the three layers—zona glomerulosa, fasciculata, and reticularis—which secrete mineralocorticoids (e.g., aldosterone), glucocorticoids (e.g., cortisol), and androgens (e.g., dehydroepiandrosterone [DHEA]), respectively (Fig. 8-11).
12 One treatment for Cushing disease is to remove both adrenal glands (bilateral adrenalectomy). What might happen to the pituitary gland following such a surgery?
Hypercortisolism regardless of cause is termed Cushing syndrome. The most common cause of Cushing syndrome is the iatrogenic administration of steroids.
Hypercortisolism from a hypersecreting pituitary adenoma is termed Cushing disease.
Classic signs and symptoms of Cushing syndrome include:



Bilateral adrenalectomy for Cushing disease (rarely done!) can cause Nelson’s syndrome, which is characterized by diffuse hyperpigmentation as a result of increased melanocyte-stimulating hormone (MSH) production.
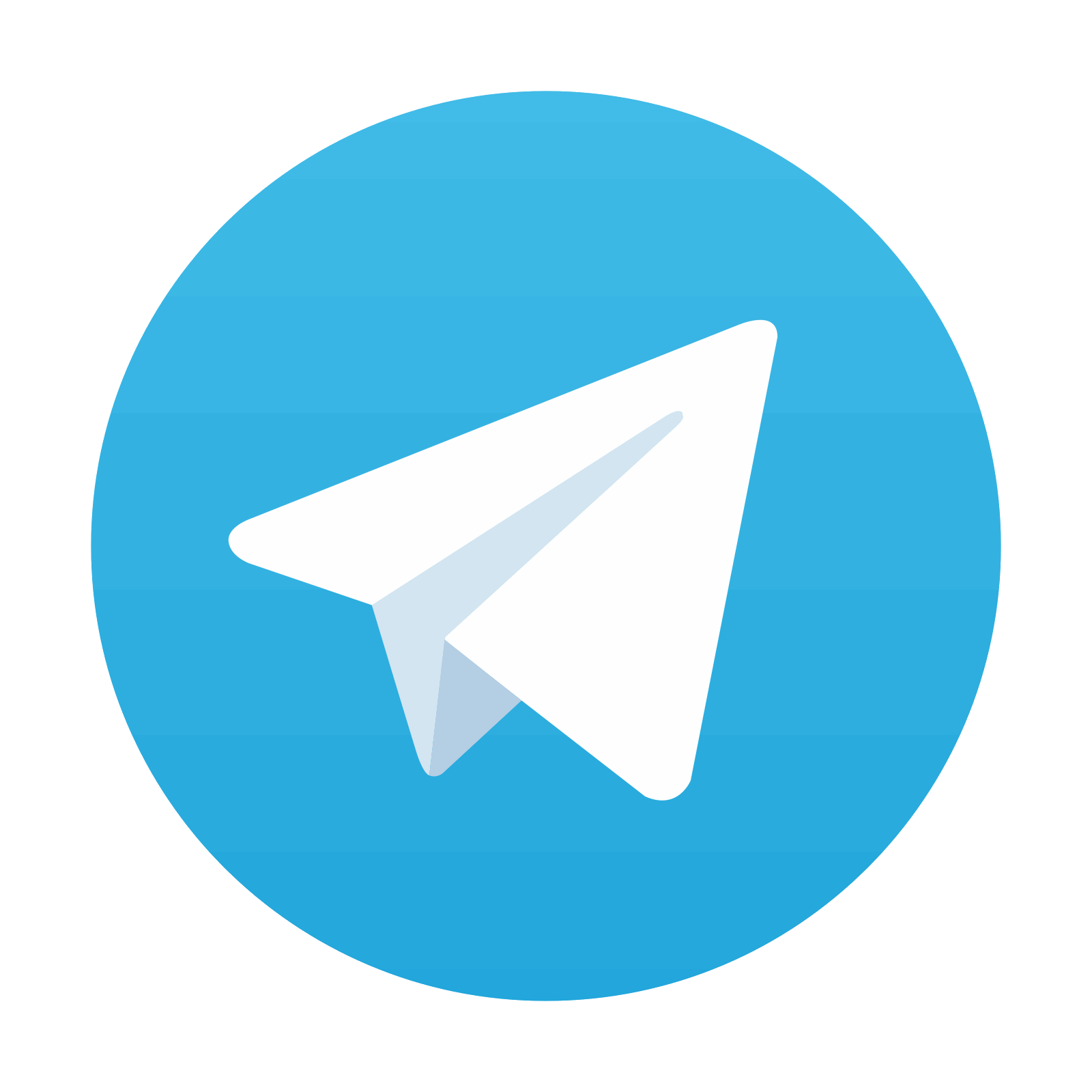
Stay updated, free articles. Join our Telegram channel

Full access? Get Clinical Tree
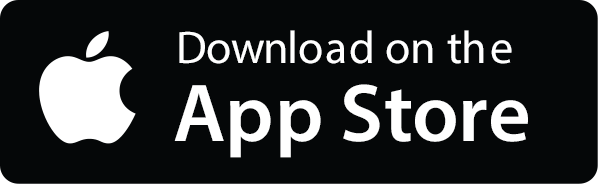
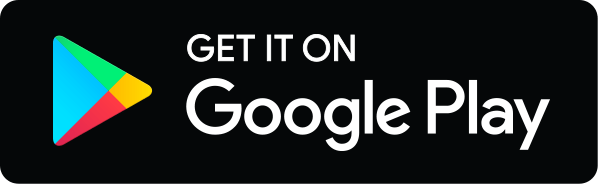