Barbara Lauritsen Christensen
Care of the patient with a respiratory disorder
Objectives
1. Differentiate between external and internal respiration.
2. Describe the purpose of the respiratory system.
3. List and define the parts of the upper and lower respiratory tracts.
4. List the ways in which oxygen and carbon dioxide are transported in the blood.
6. Identify those signs and symptoms that indicate a patient is experi encing hypoxia.
7. Differentiate among sonorous wheezes, sibilant wheezes, crackles, and pleural friction rub.
11. Discuss nursing interventions for the patient with a laryngectomy.
13. List five nursing interventions to assist patients with retained pulmonary secretions.
14. Differentiate between tuberculosis infection and tuberculosis disease.
15. List four medications commonly prescribed for the patient with tuberculosis.
17. Discuss three risk factors associated with pulmonary emboli.
19. Differentiate between medical management of the patient with emphysema and the patient with asthma.
20. Discuss why low-flow oxygen is required for patients with emphysema.
21. State three possible nursing diagnoses for the patient with altered respiratory function.
Key terms
adventitious (d-v
nt-T
-sh
s, p. 379)
atelectasis (-t
-L
K-t
-s
s, p. 410)
bronchoscopy (brng-K
S-k
-p
, p. 381)
cor pumonale (kr p
l-m
-N
-l
, p. 421)
embolism (M-b
-l
z-
m, p. 416)
epistaxis (p-
-ST
K-s
s, p. 384)
exacerbation (g-z
s-
r-B
-sh
n, p. 424)
extrinsic (k-STR
N-z
k, p. 426)
hypercapnia (h-p
r-K
P-n
–
, p. 424)
hypoventilation (h-p
-v
n-t
-L
-sh
n, p. 410)
intrinsic (n-TR
N-z
k, p. 426)
orthopnea (r-th
p-N
–
, p. 379)
pleural friction rubs (PL-rl FR
K-sh
n r
bz, p. 379)
pneumothorax (n-m
-TH
-r
ks, p. 411)
sibilant wheezes (SB-
-l
nt w
z-
z, p. 379)
sonorous wheezes (s-N
R-
s w
z-
z, p. 379)
stertorous (STR-t
r-
s, p. 385)
thoracentesis (th-r
-s
n-T
-s
s, p. 378)
Anatomy and physiology of the respiratory system
For the millions of cells throughout the body to carry out their specialized activities, they must have a continuous supply of oxygen. External respiration, or breathing, is the exchange of oxygen and carbon dioxide between the lung and the environment. As air is inhaled, it is warmed, moistened, and filtered to prepare it for use by the body. The respiratory system works with the cardiovascular system to deliver oxygen to the cells, where it provides energy to carry out metabolism. Internal respiration is the exchange of oxygen and carbon dioxide at the cellular level. Oxygen enters the cells while carbon dioxide leaves them. The gases diffuse across the cell membrane into the bloodstream, which plays the role of transporter. Failure of the respiratory system or cardiovascular system has the same result: rapid cell death from oxygen starvation. Figure 9-1 shows the structure of the respiratory organs.
Upper respiratory tract
Nose
Air enters the respiratory tract through the nose. The air is filtered, moistened, and warmed as it enters the two nasal openings (nares) and travels to the nasal cavity. The nasal septum separates the nares. This entire area is lined with mucous membrane, which is vascular. The mucous membrane provides warmth and moisture and secretes 1 L of moisture every day.
Lateral to the nasal cavities are three scroll-like bones called turbinates or conchae (Figure 9-2), which cause the air to move over a larger surface area. This increase in surface area provides more time for warming and moisturizing the air. Lining the nasal cavities are tiny hairs, which trap dust and other foreign particles and prevent them from entering the lower respiratory tract.
Communicating with the nasal structures are paranasal sinuses (Figure 9-3). They are called the frontal, maxillary, sphenoid, and ethmoid cavities. These are hollow areas that make the skull lighter and are believed to give resonance to the voice. They are lined with mucous membranes that are continuous with the nasal cavity. Because of this, nasal infections can cause sinusitis, which is uncomfortable and difficult to treat.
The receptors for the sense of smell are located in the mucosa of the nasal cavities. They are the nerve endings of the olfactory nerve, the first cranial nerve. The nasolacrimal ducts, or tear ducts, communicate with the upper nasal chamber. Hence, when an individual cries, there are copious nasal secretions.
Pharynx
The pharynx, or throat (a tubular structure about 5 inches [13 cm] long extending from the base of the skull to the esophagus and situated just in front of the vertebrae), is the passageway for both air and food. At the distal end of the pharynx are three subdivisions: (1) nasopharynx (superior portion), (2) oropharynx (posterior to mouth), and (3) laryngopharynx (directly superior to larynx) (see Figure 9-2).
The eustachian tubes enter either side of the nasopharynx, connecting it to the middle ear. Because the inner linings of the pharynx and the eustachian tube are continuous, an infection of the pharynx can spread easily to the ear. This is common in children. The adenoids (pharyngeal tonsils) are in the nasopharynx, whereas the palatine tonsils are in the oropharynx.
Larynx
The larynx (Figure 9-4, A), or organ of voice, is supported by nine areas of cartilage and connects the pharynx with the trachea. The largest area of cartilage is composed of two fused plates and is called the thyroid cartilage, or Adam’s apple. It is the same size in girls and boys until puberty, when it enlarges in boys and produces a projection in the neck. The epiglottis, a large leaf-shaped area of cartilage, protects the larynx when swallowing. It covers the larynx tightly to prevent food from entering the trachea and directs the food to the esophagus (Figure 9-4, B).
The larynx contains the vocal cords. During expiration, air rushes over the vocal cords, causing them to vibrate. This enables speech to occur. The opening between the vocal cords is the glottis.
Trachea
The trachea (Figure 9-5), or windpipe, is a tubelike structure that extends approximately 41⁄3 inches (11 cm) to the midchest, where it divides into the right and left bronchi. It lies anterior to the esophagus and connects the larynx with the bronchi. The ventral (anterior) surface of the tube is covered in the neck by the isthmus (narrow connection) of the thyroid gland. It contains C-shaped cartilaginous rings that keep it from collapsing. The open part of the C-shaped rings lies posterior to the column anterior to the esophagus, which allows the esophagus to expand during swallowing while maintaining patency of the trachea. This is necessary for uninterrupted breathing.
The entire structure is lined with mucous membranes and tiny cilia (small, hairlike processes on the outer surfaces of small cells, which produce motion or current in a fluid) that sweep dust or debris upward toward the nasal cavity. Any large particles initiate the cough reflex, which aids in the evacuation of foreign material. Sometimes, because of an airway obstruction, a physician performs a tracheostomy (a surgical opening into the trachea through which an indwelling tube may be inserted). Once this procedure is completed, the individual breathes through the tracheal opening rather than the nose. The opening is below the larynx, so air cannot pass over the vocal cords. The vocal cords cannot vibrate, and speech becomes physiologically impossible.
Lower respiratory tract
Bronchial tree
As the trachea enters the lungs, it divides into the right and left bronchi. The right bronchus enters the right lung. It is larger in diameter and more vertical in descent. The left bronchus enters the left lung. It is smaller in diameter and slightly horizontal in position. Because of this design, foreign objects that are aspirated generally enter the right bronchus.
The large bronchi continue to divide into smaller structures called bronchioles. These structures divide into smaller, tubelike structures called terminal bronchioles or alveolar ducts. All these structures are lined with ciliated mucous membrane, as is the trachea. The end structures of the bronchial tree are called alveoli. These saclike structures resemble a bunch of grapes. A single grapelike structure is called an alveolus (Figures 9-1 and 9-6). In this terminal structure of the bronchial tree, gas exchange takes place. Each alveolus is surrounded by a blood capillary, where diffusion of carbon dioxide and oxygen occurs. Alveoli are effective in gas exchange, mainly because they are extremely thin walled; each alveolus lies in contact with a blood capillary. In addition, each alveolus is coated with a thin covering of surfactant. Surfactant reduces the surface tension of the alveolus and prevents it from collapsing after each breath (see Figure 9-6).
The lungs contain millions of alveoli; they give shape and form to the lungs. They are filled with air, and lung tissue would float if it was put in water. This tiny, grapelike structure is the most important feature of the respiratory system. It is here that the oxygen diffuses into the cardiovascular system.
Mechanics of breathing
Thoracic cavity
The lungs occupy almost all the thoracic cavity except the centermost area, the mediastinum, which contains the heart and the great vessels. This cavity, the interpleural space, is enclosed by the sternum, the ribs, and the thoracic vertebrae.
Lungs
The lungs are large, paired, spongy cone-shaped organs (see Figure 9-5). The right lung weighs approximately 625 g; the left lung weighs approximately 570 g. The right lung contains three lobes; the left lung contains only two lobes. Located approximately 1 inch (2.5 cm) above the first rib is the narrow part (the apex) of each lung. The broad, inferior part (the base) lies in the diaphragm.
The lungs receive their blood supply, which comes directly from the heart, through the pulmonary arteries. By the time the blood reaches the lung capillaries, it is low in oxygen content. Because alveolar air is rich in oxygen, diffusion causes movement of oxygen from the area of high concentration. Carbon dioxide also diffuses between blood and lung capillaries and alveolar air. Blood flowing through the lung capillaries is high in carbon dioxide. After carbon dioxide is diffused into the alveoli and oxygen is diffused into the blood, carbon dioxide leaves the body by expiration of air from the lungs. The blood, now rich in oxygen, returns to the heart for circulation to the body via the pulmonary veins to the left atrium.
The surface of each lung is covered with a thin, moist, serous membrane called the visceral pleura. The walls of the thoracic cavity are covered with the same type of membrane called the parietal pleura. The pleural cavity around the lungs is an airtight vacuum that contains negative pressure. The air in the lungs is at atmospheric pressure—higher than in the pleural cavity. The negative pressure assists in keeping the lungs inflated. The visceral and parietal pleura produce a serous secretion, which allows the lung to slide over the walls of the thorax while breathing. Usually the body produces the exact amount of serous secretion needed. If too much serous secretion is produced, fluid accumulates in the pleural space; this is called pleural effusion. The pleural space becomes distended and puts pressure on the lungs, making it difficult to breathe. The physician may decide to remove the fluid by performing a thoracentesis—inserting a needlelike instrument into the pleural space and removing the fluid.
Respiratory movements and ranges
The rhythmic movements of the chest walls, ribs, and associated muscles when air is inhaled and exhaled make up the respiratory movements. The combination of one inspiration and one expiration equals one respiration. At rest the normal inspiration lasts about 2 seconds and expiration about 3 seconds.
Room air, when inhaled, contains about 21% oxygen; exhaled air contains 16% oxygen and 3.5% carbon dioxide. This represents the actual amount of oxygen used in a single breath.
The normal range of respiration for an adult at rest is 14 to 20 breaths/min. This rate can be affected by many variables, including age, sex, activity, disease, and body temperature. The respiratory rate is 40 to 60 breaths/min for a newborn, 22 to 24 breaths/min for an early school-age child, and 20 to 22 breaths/min for a teenager. The normal range for women is higher than that for men.
Members of the health care team should assess all factors influencing the patient’s respirations and should count the respirations without the patient’s awareness to prevent alterations in the breathing pattern.
Regulation of respiration
Nervous control
The medulla oblongata and pons of the brain are responsible for the basic rhythm and depth of respiration. The body’s demands can modify the rhythm. Other parts of the nervous system help coordinate the transfer from inspiration to expiration. Chemoreceptors in the carotid and aortic bodies are specialized receptors. When stimulated by increasing levels of blood carbon dioxide, decreasing levels of blood oxygen, or increasing blood acidity, these receptors send nerve impulses to the respiratory centers, which in turn modify respiratory rates.
Carbon dioxide, which is present in the blood as carbonic acid, is considered the chemical stimulant for regulation of respiration. Therefore the more carbon dioxide in the blood, the more acidic the blood becomes. After exhalation the blood becomes more alkaline. The normal pH of the blood is 7.35 to 7.45—a narrow range. Deviation from this range causes the patient to develop either acidosis or alkalosis.
Assessment of the respiratory system
The function of the respiratory system is gas exchange (oxygen and carbon dioxide) at the alveolar-capillary level. This function depends on the lungs’ capability for contraction and expansion, which in turn is influenced by musculoskeletal and neurologic functions.
Physical assessment of the patient’s general health always includes the respiratory system. More extensive assessments are required for patients with acute or chronic respiratory or cardiac conditions, those with a history of respiratory impairment related to trauma or allergic reactions, or those who have recently undergone surgery or anesthesia. Because physical and emotional responses are often correlated, also inquire about any accompanying anxiety or stress. This information should be obtained in an unhurried, matter-of-fact manner.
The respiratory assessment includes collection of subjective data. During the interview, encourage the patient to describe any symptoms, such as shortness of breath, dyspnea on exertion, or cough. Dyspnea, or difficulty breathing, is a subjective experience that only the patient can accurately describe. Data should include onset; duration; precipitating factors; and relief measures, such as position and use of over-the-counter or prescribed medications. If the patient reports a cough, ask for a description of the cough: productive or nonproductive; harsh, dry, or hacking; and color and amount of mucus expectorated. Record this information as direct quotes from the patient when possible.
Next, gather objective data. Begin the assessment with observation. Assess respiratory rate and oxygen saturation. The patient’s expression, chest movement, and respirations all provide valuable visual clues. At times the patient cannot verbalize distress, but has a wide-eyed, anxious look that reflects the fear of suffocating. Flaring nostrils indicate the patient is struggling to breathe, which is usually a late sign of respiratory distress. Initial observation yields information on the patient’s skin color and turgor. Note any obvious respiratory distress, wheezes, or orthopnea (an abnormal condition in which a person must sit or stand to breathe deeply or comfortably).
To continue the assessment, auscultate all lung fields, anteriorly and posteriorly, noting the presence of adventitious sounds (abnormal sounds superimposed on breath sounds, including sibilant wheezes [formerly called simply wheezes], sonorous wheezes [formerly called rhonchi], crackles [formerly called rales], and pleural friction rubs) (Table 9-1). Sibilant wheezes are musical, high-pitched, squeaking or whistling sounds, caused by the rapid movement of air through narrowed bronchioles. Sonorous wheezes are low-pitched, loud, coarse, snoring sounds. They are often heard on expiration. Crackles are short, discrete, interrupted crackling or bubbling sounds that are most commonly heard during inspiration. Crackles sound like hairs being rolled between the fingers close to the ear. They are thought to occur when air is forced through respiratory passages narrowed by fluid, mucus, or pus. They are associated with inflammation or infection of the small bronchi, bronchioles, and alveoli. Pleural friction rubs are low-pitched, grating or creaking lung sounds that occur when inflamed pleural surfaces rub together during respiration.
Table 9-1
TYPE | CHARACTERISTICS | COMMENTS |
Crackles (rales) | Brief, not continuous; more common in inspiration; interrupted crackling or bubbling sounds, similar to those produced by hairs being rolled between the fingers close to the ear | Caused by fluid, mucus, or pus in the small airways and alveoli. |
Fine crackles | As described above; high-pitched, sibilant crackling at end of inspiration | Found in diseases affecting bronchioles and alveoli. |
Medium crackles | As described above; medium pitch, more sonorous, moisture sound during midinspiration | Associated with diseases of small bronchi. |
Coarse crackles | As described above; loud, bubbly sound in early inspiration | Associated with diseases of small bronchi. |
Sonorous wheezes (rhonchi) | Deep, running sound that may be continuous; loud, low, coarse sound (like a snore) heard at any point of inspiration or expiration | Caused by air moving through narrowed tracheobronchial passages (caused by secretions, tumor, spasm); cough may alter sound if caused by mucus in trachea or large bronchi. |
Sibilant wheezes (wheezes) | High-pitched, musical, whistlelike sound during inspiration or expiration; sound may be several notes or one, and may vary from one minute to the next | Caused by narrowed bronchioles; bilateral wheeze often result of bronchospasm; unilateral, sharply localized wheeze may result from foreign matter or tumor compression. |
Pleural friction rub | Dry, creaking, grating, low-pitched sound with a machinelike quality during both inspiration and expiration; loudest over anterior chest | Sound originates outside respiratory tree, usually caused by inflammation; over the lung fields it suggests pleurisy; over the pericardium it suggests pericarditis with a pericardial friction rub. To distinguish the two, ask the patient to hold the breath briefly. If the rubbing sound persists, it is a pericardial friction rub because the inflamed pericardial layers continue rubbing together with each heartbeat; a pleural rub would stop when breathing stops. |
Also assess chest movement. Note whether the chest expands equally on both sides; chest expansion on one side only may indicate serious pulmonary complications. Look for retraction of the chest wall between the ribs and under the clavicle during inspiration. This can signal late-stage respiratory distress. Be alert for signs and symptoms of hypoxia (oxygen deficiency) (Box 9-1).
Laboratory and diagnostic examinations
A variety of tests are used to evaluate respiratory status and identify respiratory conditions. Other tests include diagnostic imaging, laboratory work, and more invasive measures. Nurses should be familiar with these tests so they can adequately prepare the patient.
Chest roentgenogram
Usually referred to as chest radiographs, chest roentgenograms are an essential diagnostic tool for evaluating disorders of the chest. A chest radiograph provides visualization of the lungs, ribs, clavicles, humeri, scapulae, vertebrae, heart, and major thoracic vessels. This test gives information on alterations in size and location of the pulmonary structures and blood flow, and it identifies lesions, infiltrates, foreign bodies, or fluid. A chest radiograph also shows whether a disorder involves the lung parenchyma (the tissue of an organ, as distinguished from supporting or connective tissue) or the interstitial spaces. Chest radiographs can confirm pneumothorax, pneumonia, pleural effusion, and pulmonary edema.
The chest radiographic examination can be performed at different angles for greater clarification. Have the patient wear a hospital gown tied in back. Do not use pins. Any article of clothing containing metal (e.g., a bra with metal hooks) or jewelry must be removed, since the metal produces a shadow on the film.
Computed tomography
Chest CT scan
Computed tomography (CT) scans of the lungs take pictures of small layers of pulmonary tissue, usually to identify a pulmonary lesion. These views can be diagonal or cross-sectional, with a scanner rotating at various angles. Although this test is painless and noninvasive and results in little radiation exposure, patient teaching is necessary before the procedure to offer explanations and allay anxiety.
Helical or spiral CT chest scan
Helical (also called spiral or volume-averaging) CT scanning represents a marked improvement over standard CT scanning. The helical CT scan continuously obtains images. This produces faster and more accurate images. Because the helical CT can scan the abdomen and chest in less than 30 seconds, the entire study can be performed with one breath-hold. Furthermore, when contrast material is used, the entire region can be imaged in just a few seconds after the contrast injection (Pagana & Pagana, 2007).
Pulmonary angiography (pulmonary arteriography)
Pulmonary angiography (pulmonary arteriography) uses a radiographic contrast material injected into the pulmonary arteries to permit visualization of the pulmonary vasculature. Angiography is used to detect pulmonary embolism (PE) and a variety of congenital and acquired lesions of the pulmonary vessels.
When PE is suspected, lung scanning is performed first. If the lung scan is normal, PE is ruled out. If the scan is uncertain however, the diagnosis of PE is questionable because pathologic processes (e.g., emphysema, pneumonia) also may cause abnormalities on the lung scan. Definitive diagnosis for PE may require pulmonary angiography (Pagana & Pagana, 2007).
Ventilation-perfusion scan (V/Q scan)
Ventilation-perfusion (V/Q) scanning is used primarily to check for a PE. An intravenous (IV) radioisotope is given for the perfusion portion of the test, and the pulmonary vasculature is outlined and photographed. For the ventilation portion of the test, the patient inhales a radioactive gas that outlines the alveoli, and another photograph is taken. Normal scans show homogeneous radioactivity. Diminished or absent radioactivity suggests lack of perfusion or airflow (Lewis et al., 2007).
Pulmonary function testing
Pulmonary function tests (PFTs) are performed to assess the presence and severity of disease in the large and small airways. PFTs include various procedures to obtain information on lung volume, ventilation, pulmonary spirometry, and gas exchange. Lung volume tests refer to the volume of air that can be completely and slowly exhaled after a maximum inhalation (vital capacity). Inspiratory capacity is the largest amount of air that can be inhaled in one breath from the resting expiratory level. Total lung capacity is calculated to determine the volume of air in the lung after a maximal inhalation. Ventilation tests evaluate the volume of air inhaled or exhaled in each respiratory cycle. Pulmonary spirometry tests evaluate the amount of air that can be forcefully exhaled after maximum inhalation. These tests require the use of a spirometer.
One of the most important tools for diagnosing respiratory diseases is gas exchange, which identifies the capacity for diffusion of carbon dioxide. This component of PFT determines the degree of function in the pulmonary capillary beds in contact with functioning alveoli.
Mediastinoscopy
Mediastinoscopy is a surgical endoscopic procedure in which an incision is created in the suprasternal notch, allowing the endoscope to be passed into the upper mediastinum. This is performed to gather a sample of lymph nodes for biopsy for tumor diagnosis. Because these lymph nodes receive lymphatic drainage from the lungs, they help diagnose malignant tumors. Tumors in the mediastinum (e.g., thymoma or lymphoma) can also be biopsied through the mediastinoscope (Pagana & Pagana, 2007). This procedure is performed in the operating room, with the patient under general anesthesia.
Laryngoscopy
Laryngoscopy can be performed for either direct or indirect visualization of the larynx. Indirect laryngoscopy is probably the most common procedure for assessing respiratory difficulties; this entails using a laryngeal mirror in the awake patient’s mouth for visualization. This procedure can be used for biopsy or polyp excision. Direct laryngoscopy requires local or general anesthesia and exposes the vocal cords with a laryngoscope passed down over the tongue.
Bronchoscopy
Bronchoscopy is performed by passing a bronchoscope into the trachea and bronchi. Using either a rigid bronchoscope or a flexible fiberoptic bronchoscope (the instrument of choice in most cases) allows visualization of the larynx, the trachea, and the bronchi (Figure 9-7). Diagnostic bronchoscopic examination includes observation of the tracheobronchial tree for (1) abnormalities, (2) tissue biopsy, and (3) secretions collected for cytologic (cell) or bacteriologic examination. A local anesthetic agent may be used, but an IV general anesthetic agent is usually given. The patient is treated as a surgical patient.
Nursing interventions for patients after bronchoscopy include (1) keeping the patient on NPO (nothing by mouth) status until gag reflex returns, usually about 2 hours after the procedure; (2) keeping the patient in a semi-Fowler’s position and turning on either side to facilitate removal of secretions (unless the physician specifies another position); (3) monitoring the patient for signs of laryngeal edema or laryngospasms, such as stridor or increasing dyspnea; and (4) if lung tissue biopsy is taken, monitoring sputum for signs of hemorrhage (blood-streaked sputum is expected for a few days after biopsy).
Sputum specimen
Sputum samples frequently are obtained for microscopic evaluation, such as Gram stain and culture and sensitivity (Box 9-2). For the range of sputum characteristics, see Box 9-3.
Cytologic studies
Cytologic tests can be performed on any body secretion, such as sputum or pleural fluid, to detect abnormal or malignant cells.
Lung biopsy
Lung biopsy may be done transbronchially or as an open-lung biopsy. The purpose is to obtain tissue, cells, or secretions for evaluation. Transbronchial lung biopsy involves passing a forceps or needle through the bronchoscope to obtain a specimen. Specimens can be cultured or examined for malignant cells. Nursing interventions are the same as for fiberoptic bronchoscopy. Open-lung biopsy is used when pulmonary disease cannot be diagnosed by other procedures. The patient is anesthetized, the chest is opened with a thoracotomy incision, and a biopsy specimen is obtained.
Thoracentesis
Thoracentesis is the surgical perforation of the chest wall and pleural space with a needle for the aspiration of fluid for diagnostic or therapeutic purposes or for the removal of a specimen for biopsy (Figure 9-8). Indications for fluid removal for diagnostic purposes include (1) examining the pleural fluid for specific gravity, white blood cell count, red blood cell count, protein, and glucose; and (2) culturing the fluid for pathogens and checking for abnormal or malignant cells. Record the gross appearance of the fluid, the quantity obtained, and the site of the thoracentesis.
Therapeutic indications for thoracentesis include removal of fluid when it is a threat to patient safety or comfort and instillation of medication into the pleural space.
Nursing interventions for the patient undergoing thoracentesis include explaining the procedure and obtaining a written consent. Try to relieve the patient’s anxiety. The procedure is usually carried out in the patient’s room. The patient sits on the edge of the bed with the head and arms resting on a pillow placed on an overbed table. If the patient cannot sit up, turn him or her to the unaffected side with the head of the bed elevated 30 degrees.
Monitor vital signs, general appearance, and respiratory status throughout the procedure. Usually no more than 1300 mL of pleural fluid should be removed within a 30-minute period because of the risk of intravascular fluid shift with resultant pulmonary edema. After thoracentesis, position the patient on the unaffected side. Label the specimen and send it immediately to the laboratory per physician’s orders.
Arterial blood gases
Blood gas analysis is an essential test in diagnosing and monitoring patients with respiratory disorders. The lungs’ ability to oxygenate arterial blood adequately is determined by examination of the arterial oxygen tension (PaO2) and arterial oxygen saturation (SaO2). Oxygen is carried in the blood in two forms: dissolved oxygen and oxygen in combination with hemoglobin. The PaO2 represents the amount of oxygen dissolved in the plasma and is expressed in millimeters of mercury (mm Hg). The percentage of hemoglobin binding sites that have oxygen bound to them is called saturation (SaO2) (Woodruff, 2006). The SaO2 is the amount of oxygen bound to hemoglobin in comparison with the amount of oxygen the hemoglobin can carry. The SaO2 is expressed as a percentage. For example, if the SaO2 is 90%, then 90% of the hemoglobin attachments for oxygen have oxygen bound to them. Oxygen must first dissolve in blood (PaO2) before it can bind to hemoglobin (SaO2) (Woodruff, 2006) (Box 9-4).
The PCO2 is a measure of the partial pressure of carbon dioxide in the blood. PCO2 is referred to as the respiratory component in acid-base determination because this value is primarily controlled by the lungs. As the carbon dioxide level increases, the pH decreases. Therefore the carbon dioxide level and pH are inversely proportional. The PCO2 level is elevated in primary respiratory acidosis and decreased in primary respiratory alkalosis. Because the lungs compensate for primary metabolic acid-based derangements, PCO2 levels are affected by metabolic disturbances as well. In metabolic acidosis the lungs attempt to compensate by “blowing off” carbon dioxide to raise pH. In metabolic alkalosis the lungs attempt to compensate by retaining carbon dioxide to lower pH.
The bicarbonate ion (HCO3−) is a measure of the metabolic (renal) component of the acid-base equilibrium. This ion can be measured directly by the bicarbonate value or indirectly by the carbon dioxide content. As the HCO3− level increases, the pH also increases; therefore the relationship of bicarbonate to pH is directly proportional. HCO3− is elevated in metabolic alkalosis and decreased in metabolic acidosis. The kidneys also compensate for primary respiratory acid-base derangements. For example, in respiratory acidosis, the kidneys attempt to compensate by reabsorbing increased amounts of HCO3−. In respiratory alkalosis the kidneys excrete HCO3− in increased amounts in an attempt to lower pH through compensation (Table 9-2).
Table 9-2
Acid-Base Disturbances and Compensatory Mechanisms
ACID-BASE DISTURBANCE | MODE OF COMPENSATION |
Respiratory acidosis | Kidneys retain increased amounts of HCO3− to increase pH. |
Respiratory alkalosis | Kidneys excrete increased amounts of HCO3− to lower pH. |
Metabolic acidosis | Lungs “blow off” carbon dioxide to raise pH. |
Metabolic alkalosis | Lungs retain carbon dioxide to lower pH. |
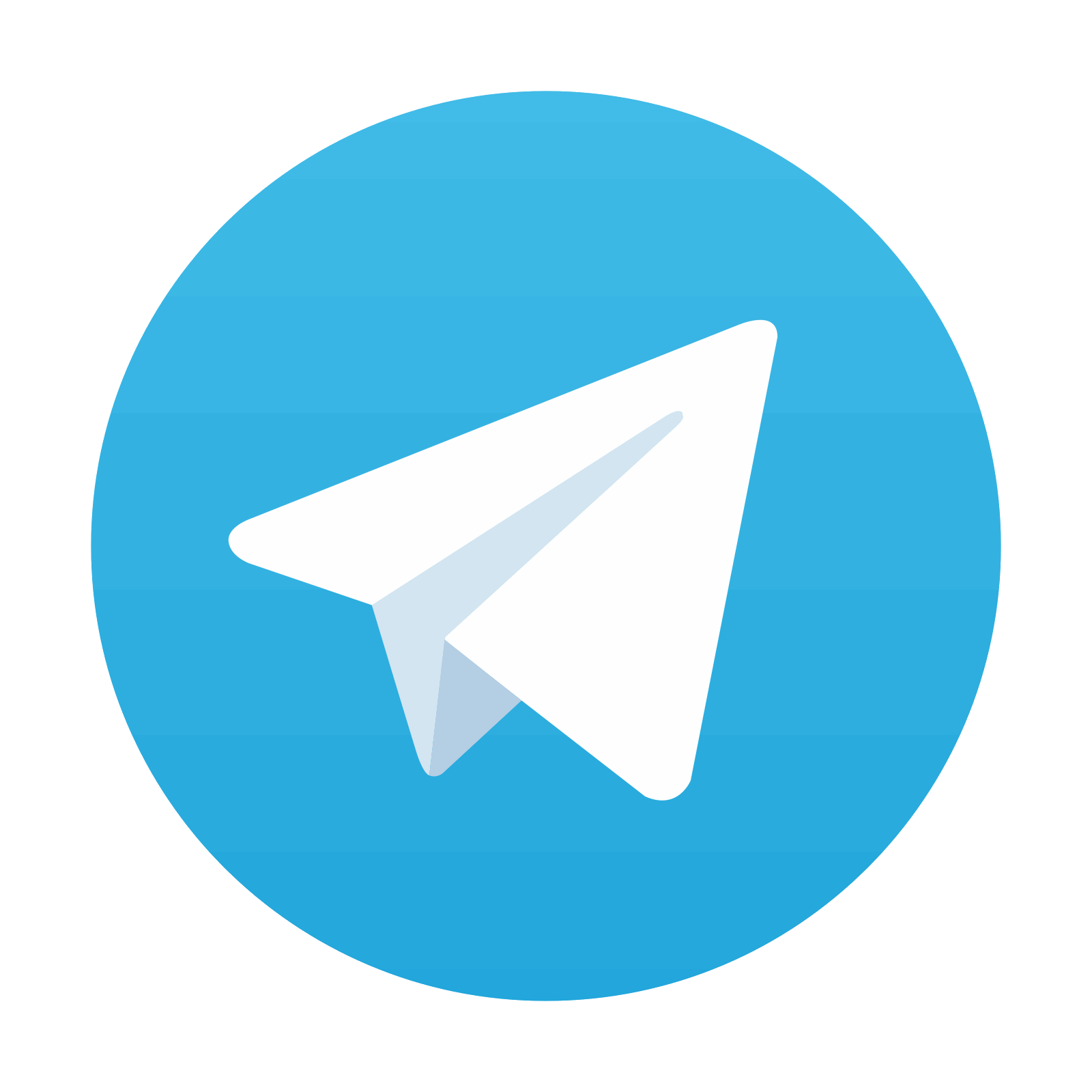
Stay updated, free articles. Join our Telegram channel

Full access? Get Clinical Tree
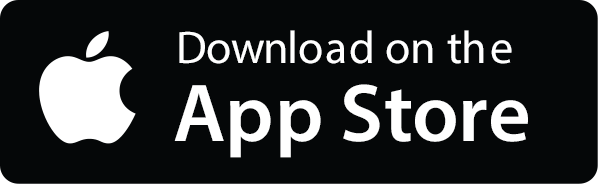
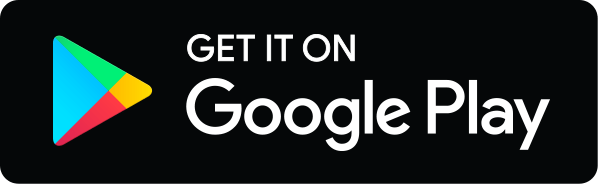