Chemistry
Objectives
After completing this chapter you should be able to:
Fundamental Concepts
1. Identify the plasma components in peripheral blood, and describe their function and significance.
2. Describe the proper specimen collection for various chemistry tests.
3. Explain the basic principles of glucose and fat metabolism.
CLIA-Waived Chemistry Tests
Advanced Concepts
1. List eight critical chemistry tests performed during medical emergencies.
2. Match chemistry panels with the tests performed.
Key Terms
absorbance photometry indirect measurement of the amount of light that a solution absorbs
anion negatively charged ion
atherosclerosis formation of plaque along the inside walls of blood vessels
Beer–Lambert Law law stating that intensity of color change is directly proportional to the concentration of an analyte in a solution
carbohydrates sugars and starches
catalysts chemicals that produce specific changes in other substances without being changed themselves
cation positively charged ion
clinical diagnosis diagnosis based on the patient’s initial signs and symptoms
clot activator chemical additive that speeds up the clotting of a blood specimen
definitive diagnosis final, confirmed diagnosis based on clinical signs and symptoms and the results of diagnostic tests
dyslipidemia abnormal amounts of fat and lipoproteins in the blood
endogenous cholesterol cholesterol manufactured in the liver
exogenous cholesterol cholesterol derived from the diet
galvanometer instrument capable of measuring the intensity of light
glycogen stored form of glucose found especially in muscles and the liver
glycosylated hemoglobin hemoglobin A molecule within red blood cells that becomes permanently bound to glucose
gout form of arthritis caused by accumulation of uric acid crystals in the synovial fluid
hyperglycemia elevated blood sugar
hyperinsulinemia excessively high blood insulin levels
hyperlipidemia excessive fat in blood, which gives plasma a milky appearance
hypoglycemia low blood sugar
insulin resistance condition in which insulin is not effective at moving the glucose from the blood into the cells (seen in type 2 diabetes)
ions electrolytes consisting of positively or negatively charged particles
lipoproteins protein-linked lipids
myoglobin iron-containing, oxygen-binding protein found in muscles
occult hidden or not visible to the naked eye
panels groups of tests that focus on blood cells, particular organs, or metabolic functions
reflectance photometry indirect measurement of the light that reflects off a solution
trans fats synthetic hydrogenated fats
transmittance photometry measurement of the amount of light passing through a solution
troponin I and T heart-specific indicators of a recent myocardial infarction
Abbreviations
2 hr PP 2-hour postprandial (after eating)
A1c hemoglobin A1c, glycosylated hemoglobin, or glycated hemoglobin
ALP or AP alkaline phosphatase (also abbreviated as alk phos)
AST aspartate aminotransferase
Ca calcium
Cl− chloride ion
HCO3− bicarbonate ion
HDL high-density lipoproteins, or “healthy” cholesterol
IDDM insulin-dependent diabetes mellitus (type 1)
Ig immunoglobulins (antibodies)
IGT impaired glucose tolerance
LDL low-density lipoproteins, or “lousy” cholesterol
Na+ sodium ion
NIDDM non–insulin-dependent diabetes mellitus (type 2)
OGTT oral glucose tolerance test
T4 thyroxine
TC/HDL ratio total cholesterol compared with high-density lipoprotein
TSH thyroid-stimulating hormone
VLDL very-low-density lipoprotein (e.g., protein and triglyceride)
FUNDAMENTAL CONCEPTS
Clinical chemistry is the testing of the chemical analytes found in various liquid body specimens, such as urine, whole blood, serum, plasma, synovial fluid (from joints), pleural fluid (from the chest cavity), pericardial fluid (from the sac surrounding the heart), peritoneal fluid (from the abdominal cavity), and cerebrospinal fluid. The specimens most commonly tested in the ambulatory setting are urine and blood.
Chapter 5 detailed the various cellular elements found in blood (red blood cells [RBCs], white blood cells, and platelets) and the blood coagulation process. This chapter deals with the liquid portion of blood: plasma.
Blood Plasma
Plasma is a pale yellow, sweet-smelling, sticky fluid occupying slightly more than 50% of the total blood volume. For a complete understanding of the numerous blood chemistry tests and their significance, the composition of plasma must be reviewed. Refer to the numbered flow chart (Fig. 6-1) while reading about each component.
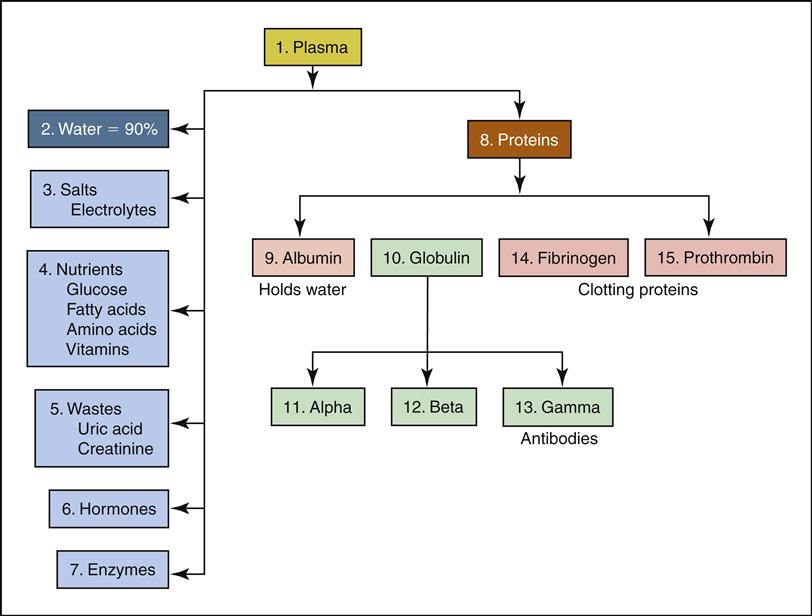
Approximately 90% of plasma (1) is water (2), with hundreds of dissolved substances and gases, including the following:
• Hormones (6)—from various endocrine glands (e.g., thyroid, pancreas)
• Proteins (8)—a major portion of plasma that can be divided into three categories: albumin, globulin, and clotting proteins
• Albumins (9)—hold the water within the blood vessels
Blood plasma is a dynamic, ever-changing liquid that reflects the inner workings of internal organs and tissues. In good health, each organ is constantly producing or removing its various chemical products within the plasma to maintain homeostasis. When disease strikes a particular organ or body system, homeostasis is interrupted and a change is seen in the blood chemistry. The measured change in blood chemistry allows a physician to detect or confirm the clinical diagnosis (diagnosis made on the basis of the patient’s initial signs and symptoms). Follow-up chemistry tests allow the physician and patient to monitor the progress of the disease.
Blood Chemistry Specimens
The required blood specimens for chemistry testing differ depending on what analyte is being tested and what testing method is used at the reference laboratory or ambulatory setting.
Reference Laboratory Specimens for Blood Chemistry Testing
Blood chemistry tests derived from a reference laboratory generally require a serum specimen, which is obtained from a coagulated (clotted) blood specimen. The vacuum tube of choice for serum specimens is usually the gold serum separator tube (SST) containing a gel and a clot activator (a chemical additive that speeds up the clotting of the blood specimen). The SST containing the blood specimen must sit for 30 minutes after drawing to form a dense clot. The clotted specimen is then centrifuged for 10 to 15 minutes. The centrifugation forces the clotted cells to the bottom of the tube. Also during centrifugation, the gel migrates up over the cells, separating the serum from the clot. Fig. 6-2 shows the SST specimen (gold-topped tube) before and after centrifugation and the serum from a centrifuged red-topped “clot” tube.
Some chemical analytes may be affected by the clot activator or gel in the SST tube, so the laboratory may request that the serum specimen be collected in a plain red-topped tube that allows the blood to clot with no additives. Be sure to check the reference laboratory requisition (or the laboratory reference manual) to confirm the correct tube to draw for the ordered analyte(s). In the sample laboratory requisition in Fig. 6-3, note the routine chemistry tests and the required tube for each of the following:
• G for gold or gel found in the SSTs —yields a serum specimen that is separated from the clot
• R for red-topped “clot” tubes—yields a serum specimen with no clot activators or gel
• P for purple ethylenediamine tetraacetic acid (EDTA) anticoagulant tubes—for hematology testing
• Lt. Blue for sodium citrate anticoagulant—for coagulation testing
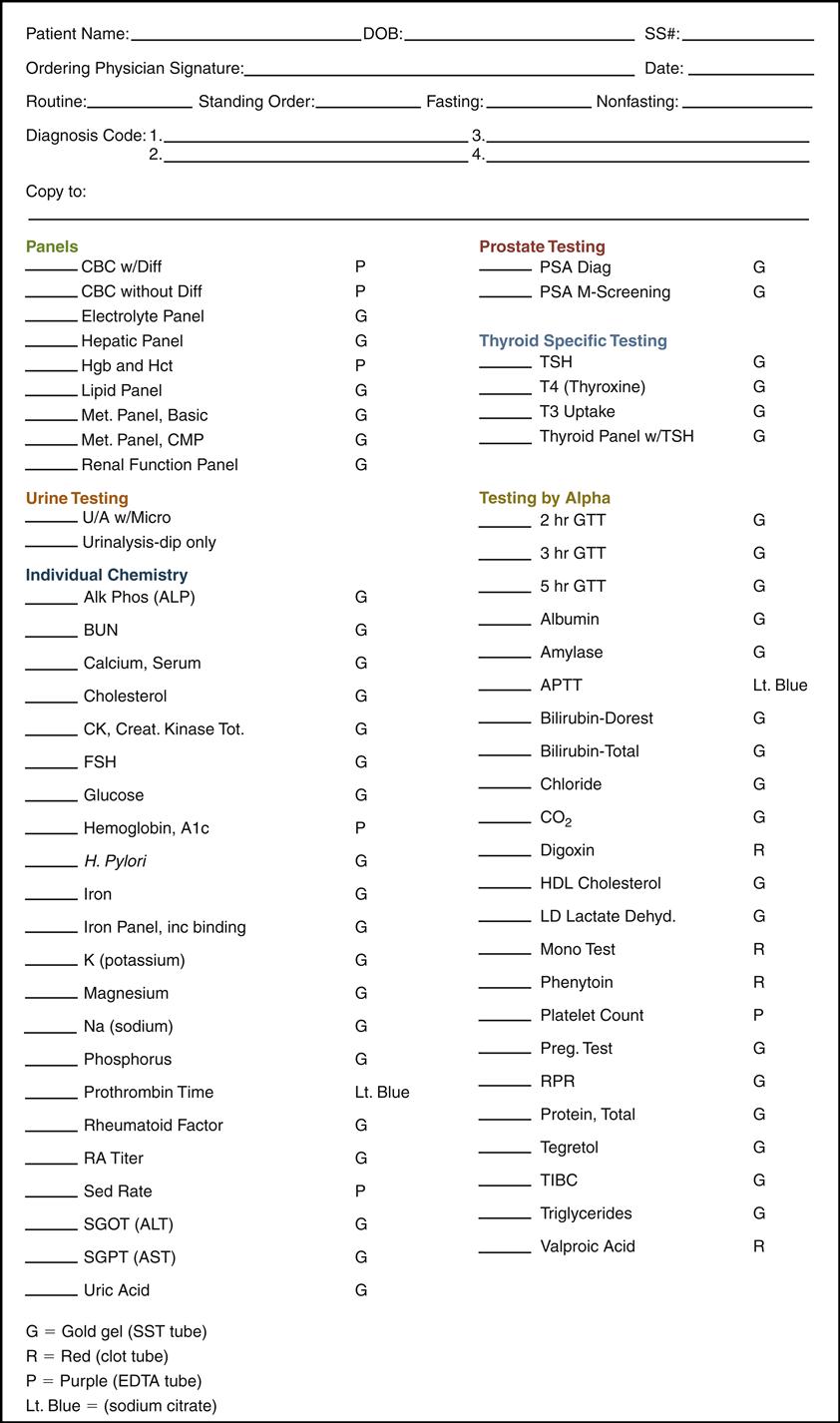
Also, notice the list of panels on the sample reference laboratory requisition. Panels are groups of tests that give results on either the blood cells (such as complete blood count [CBC] panels), particular organs (e.g., hepatic panel), or metabolic functions (e.g., Met. panels). A panel of tests can usually be run on one analyzer with one serum specimen at a much lower cost than a series of individual tests. (The various panels of tests run in reference laboratories are discussed later in this chapter.) The individual blood and urine tests are listed in alphabetical order on the requisition.
Physician’s Office Laboratory Specimens for CLIA-Waived Chemistry Tests
The most common specimen in the physician’s office laboratory (POL) for waived chemistry tests is the capillary puncture whole blood specimen. The blood is usually collected in a capillary tube containing an anticoagulant or directly into a testing device within a specified time frame. Testing devices may test the whole blood specimen directly, or they may separate the plasma from the whole blood before testing. The manufacturer’s instructions must be read for each procedure to determine the exact type of specimen that should be used for each waived test.
Some waived tests may also use a venous anticoagulated whole blood specimen in place of the capillary blood specimen. Once again, only tubes designated by the manufacturer should be used. The following are examples of CLIA-waived specimens:
Glucose Metabolism and Testing
In the ambulatory care setting, the most common CLIA-waived chemistry tests are designed to screen and monitor glucose and lipid metabolism. These in-office tests allow the physician to receive results immediately and counsel the patients while they are still in the office. The physician may then order additional diagnostic tests from the reference laboratory to reach a definitive diagnosis (the final, confirmed diagnosis based on the initial clinical signs and symptoms of the patient compared with the results of the tests from the reference laboratory).
Glucose Metabolism
Glucose is a simple six-carbon sugar that all cells and tissues require for life-giving energy. Glucose enters the blood after the digestion of carbohydrates. Once glucose is in the blood, it is distributed, metabolized, and processed in the following cells, tissues, and organs:
• Body cells—all take in blood glucose for energy
• Adipose tissues (fat)—take in fatty acids and glucose to produce triglycerides
Glucose levels in the blood must be maintained within a homeostatic range of 70 to 110 mg/dL. The monitoring and control of glucose levels within this range are controlled by two hormones: insulin and its antagonist, glucagon (Fig. 6-4). Both hormones are produced by the islets of Langerhans within the pancreas.
When glucose levels rise, as seen after eating, the hormone insulin is secreted from the beta cells within the islets of Langerhans. Insulin reduces the level of plasma glucose by (1) promoting movement of glucose from the blood into body cells, (2) stimulating the conversion of glucose to glycogen in the liver and muscles, and (3) stimulating the conversion of glucose and fatty acids into triglycerides within the liver and adipose tissues.
When blood glucose levels decrease, as during fasting, the hormone glucagon is secreted from alpha cells within the islets of Langerhans. Glucagon increases plasma glucose by stimulating the conversion of the stored glycogen in the liver and muscles back into glucose and then releasing the glucose into the blood.
Diabetes Mellitus
Diabetes mellitus (DM) is a disorder of carbohydrate metabolism characterized by hyperglycemia (elevated blood sugar) and glycosuria (sugar in the urine) resulting from the inadequate production or use of insulin. DM occurs when the pancreas is unable to produce enough effective insulin to move the glucose from the blood into the body cells. There are several types of diabetes.
Insulin-Dependent Diabetes Mellitus (Type 1)
Insulin-dependent diabetes mellitus (IDDM) occurs in 5% to 10% of the diabetic population. It is the result of an autoimmune destruction of the pancreatic beta cells, which are then unable to produce insulin. Without insulin the body cannot move glucose out of the blood and into the tissues. Consequently, patients with IDDM must constantly monitor their blood sugar levels and inject the appropriate amount of insulin to prevent the harmful effects of hyperglycemia. If glucose levels remain high for long periods, the following severe complications may occur:
By frequently monitoring glucose levels, the diabetic patient must administer insulin to keep any rising glucose levels down, thus preventing the harmful effects of hyperglycemia and ketoacidosis. The insulin-dependent diabetic patient can also keep glucose levels in control by following a low-carbohydrate diet and exercising.
Non–Insulin-Dependent Diabetes Mellitus (Type 2)
Non–insulin-dependent, or type 2, diabetes (NIDDM) accounts for 90% to 95% of the diabetic population. It has become epidemic in the United States because of the increasing numbers of obese, sedentary individuals. These individuals generally produce insulin, but it cannot effectively move the glucose from the blood into the cells, a condition known as insulin resistance. This resistance creates high blood glucose levels (hyperglycemia). Scientific research has found that the beta cells of the pancreas become sluggish and cannot release the right amount of insulin at the right time, causing fluctuating periods of hypoglycemia (low blood sugar) and hyperglycemia. The ineffectiveness of insulin’s response to glucose is referred to as impaired glucose tolerance (IGT). IGT may then lead to hyperinsulinemia (excessive blood insulin levels). Hyperinsulinemia causes abnormal amounts of fat levels in the blood (dyslipidemia) that lead to atherosclerosis (the formation of plaque along the inside walls of blood vessels) and hypertension. The cascade effect of NIDDM that begins with insulin resistance and hyperinsulinemia, which then leads to high blood pressure and heart disease, has been described as metabolic syndrome (Fig. 6-5).
If individuals with NIDDM do not bring their high glucose levels under control, they incur the same microvascular and cardiovascular complications as out-of-control insulin-dependent diabetics. Research has shown that if individuals with NIDDM are diagnosed early, they can begin diet management, exercise, and the use of oral hypoglycemic medication to bring their sugar levels down. They may then avoid developing any of the negative complications caused by abnormally high blood sugar.
Table 6-1 summarizes the differences between type 1 and type 2 diabetes.
TABLE 6-1
Comparison of Type 1 and Type 2 Diabetes Mellitus
From Chabner D: The language of medicine, ed 8, St Louis, 2007, Saunders.
Prediabetes
Prediabetes is the state that occurs when blood glucose levels are higher than normal but not high enough for a diagnosis of diabetes. Studies have shown that type 2 diabetes develops in most people with prediabetes within 10 years. Studies have also shown that people with prediabetes can prevent or delay the development of type 2 diabetes by up to 58% through changes in lifestyle that include modest weight loss with diets containing fewer refined carbohydrates (sugars and processed flour) and regular exercise.
Gestational Diabetes
Gestational diabetes occurs during pregnancy. The mother’s IGT usually subsides after delivery of the baby. Signs and symptoms of gestational diabetes include glucosuria, hyperglycemia, excessive weight gain in the mother, and a baby weighing 9 pounds or more. Women who have gestational diabetes are also prone to developing type 2 diabetes later in life.
Transient Diabetes
Individuals may have diabetes during acute illness or steroid therapy. This form of diabetes usually lasts as long as the condition or treatment continues, then subsides.
Blood Glucose Screening and Monitoring Tests
The American Diabetes Association recommends two tests to identify diabetes and prediabetes in the early stages: the fasting plasma glucose test (FPG) and the oral glucose tolerance test (OGTT). The blood glucose levels measured from these tests determine whether an individual has prediabetes or diabetes. The POL may perform CLIA-waived whole blood glucose tests that act as a screening test for potential diabetics. If the results of the screening tests are high, they must be confirmed by the plasma test or serum test methods that are usually performed by moderately complex reference laboratories.
Fasting Blood Glucose
For a fasting blood glucose test (FBG), a patient’s fasting sample is usually taken in the morning after a fast of 10 to 14 hours. Patients cannot have any food from 10 PM until the test the next morning. Usually, they may drink only water, but check your institutional protocol for fasting preparations regarding proper timing and dietary restrictions. If the FBG is between 100 mg/dL and 126 mg/dL on two different days, the patient is considered prediabetic. If the FBG is greater than 126 mg/dL, the patient is considered diabetic (based on American Diabetes Association criteria). CLIA-waived glucose tests are for screening only and should be followed up with the more complex plasma tests for blood glucose. A definitive diagnosis is usually made with a venous specimen that has been collected in a gray-topped tube.
Oral Glucose Tolerance Test or 2-Hour Postprandial Blood Sugar
Another method of screening for diabetes mellitus is the OGTT, also called a 2-hour postprandial blood sugar (2 hr PP). The OGTT begins with the measurement of FBG. If the results are within an acceptable range after the initial test, the patient is given a glucose-rich drink or meal and retested after 2 hours. Normal blood glucose levels should be brought down to less than 140 mg/dL 2 hours after the drink. If the glucose level does not fall below 140 mg/dL but is not greater than 200 mg/dL, the patient is considered prediabetic. If the glucose level is greater than 200 mg/dL after 2 hours, the patient is considered diabetic.
Random Glucose Test
A blood specimen can also be collected at any time to see if the glucose level is within the normal range of less than 140 mg/dL. A random blood specimen greater than 200 mg/dL is indicative of diabetes.
Glucose Tolerance Test
A more thorough test for measuring an individual’s glucose metabolism is the full glucose tolerance test (GTT), in which blood glucose levels are measured at fasting and then at 1-hour intervals for 2 to 6 hours. The test generally takes place at a reference laboratory or hospital outpatient laboratory.
The GTT begins the same way the OGTT does, in that the patient comes in fasting and is initially tested for blood glucose level. If the results of the fasting test show a high glucose level (above 126), no further testing is done. If the results are within an acceptable range, the patient is given a 100-g dose of glucose to drink. Blood and urine specimens are collected and tested for glucose levels after the first half hour and then every hour for a specified period of time. The urine is tested to see if and when the blood glucose levels exceed the renal threshold for glucose of 160 to 180 mg/dL. When the renal threshold is exceeded, glucose is present in the urine.
During this test the patient should be monitored for signs of fainting or nausea. If these occur, or if the blood glucose levels exceed 300 mg/dL, the test should be terminated. If the doctor suspects hyperglycemia (diabetes), it is typically a 3-hour GTT. If the doctor suspects hypoglycemia, the test may continue for 5 or 6 hours. Fig. 6-6 shows the patterns of faulty glucose metabolism during a GTT.
Glycosylated Hemoglobin/Glycated Hemoglobin (Hgb A1c)
When hemoglobin A in the RBCs is exposed to high levels of glucose, the hemoglobin molecule is permanently glycosylated and changes into hemoglobin A1c (Hgb A1c). Because the life span of an RBC is 80 to 120 days, the percentage of glycosylated hemoglobin molecules will be proportional to the overall concentration of glucose levels in the blood over the average life span of the RBCs (approximately 2 to 3 months). A normal percentage range of glycosylated hemoglobin in a whole blood specimen from adults is 2.2% to 4.8%.
The Hgb A1c test result is especially valuable to the person who is diagnosed with diabetes (or prediabetes) because it provides an accurate long-term index of the patient’s average blood glucose level. Also, Hgb A1c test results are not subject to the daily fluctuations that occur in plasma glucose monitoring. Therefore the American Diabetes Association recommends the Hgb A1c as the best test to determine whether a patient’s blood sugar has been under control during the previous 2 to 3 months. For example, if an Hgb A1c test result rises above the recommended 6% to 8%, the patient is at risk of developing the microvascular and cardiovascular complications previously discussed. This information can be used to encourage the patient to better understand and follow the treatment plan to prevent those complications.
Lipid Metabolism and Testing
Lipids, or fats, are essential to the body. They are an alternate source of energy when blood glucose levels are down and are critical to cellular health. Research has shown that poor lipid metabolism and cardiovascular disease are directly linked. In an effort to help correct the growing problems of obesity and heart disease in the United States, annual lipid screening and monitoring tests are recommended. Lipid screening is approved as a CLIA-waived test.
Lipid Metabolism
Lipid metabolism begins with the ingestion of fats. Dietary fats and oils are digested into fatty acids, which are transported to the liver to be reassembled into lipids. These lipids, like glucose, are then transported by the blood to all the body tissues. The lipids are used in critical cellular metabolism or stored as body fat. Some of the essential functions and uses of lipids are the following:
• Production of steroidal hormones
• Transportation of fat-soluble vitamins (vitamins A, D, and E)
• Insulation against extreme temperatures
• Protection of vital organs and nerves
• Potential source of energy when glucose is not available
The two plasma lipids most routinely tested are cholesterol and triglycerides. These two lipids can be further categorized and tested according to the three ways they are transported in the blood in the form of lipoproteins (protein-linked lipids): high-density lipoproteins (HDL), low-density lipoproteins (LDL), and very-low-density lipoproteins (VLDL). Cholesterol, triglyceride, and lipoprotein levels are frequently ordered together in a lipid panel.
Cholesterol
Cholesterol is essential for the production of cell membranes, bile, myelin sheaths on nerves, and steroid hormones. Cholesterol is also required in the absorption of vitamin D. Cholesterol is found in the blood, bile, liver, brain, kidneys, and adrenal glands. Most of the cholesterol found in the blood is manufactured in the liver and is called endogenous cholesterol. In fact, the liver is able to produce all the cholesterol the body needs.
Another source of cholesterol, exogenous cholesterol, comes from what we eat. Foods high in saturated fats and trans fats tend to raise the blood level of cholesterol. Trans fats are synthetic hydrogenated fats found in partially hydrogenated margarines and oils used in cooking and baking. Saturated fats are found in meat, egg yolks, milk, and other dairy products that are usually hard or semisolid at room temperature. Both trans fats and saturated fats are known to elevate cholesterol levels, causing atherosclerosis (Fig. 6-7).
Not all dietary fats have the adverse effects of saturated fats and trans fats. Research has found that monounsaturated fats and polyunsaturated fats, which are naturally liquid at room temperature, actually lower the blood level of cholesterol. These fats can also reverse plaque buildup on blood vessel walls. Examples of “good” monounsaturated fats are canola oil, olive oil, and omega-3 oil from fish. Examples of good polyunsaturated fats are soybean, safflower, corn, and cottonseed oils.
Lipoproteins
Cholesterol and triglycerides are lipids that do not mix with the water in plasma. Consequently, the liver attaches a protein to these lipids, which then become lipoproteins. The following three types of lipoproteins are manufactured in the liver and are classified on the basis of their density:
Total Cholesterol/High-Density Lipoprotein Ratio
The total blood cholesterol level (TC) is compared with the HDL level in the form of a ratio. This ratio provides an important index for determining the cardiac risk a patient faces because of atherosclerosis. The TC/HDL ratio is more valuable than TC alone because it takes into account whether a high level of TC is made up of the HDL “healthy” cholesterol or the undesirable LDL cholesterol. A ratio above 4.5 indicates that the person either is not producing enough good HDL or is producing too much bad LDL. In either case, the individual will need to do the following to lower cardiovascular risk:
Triglycerides
Triglycerides are a direct result of diets rich in carbohydrates. Triglycerides account for 95% of the fat stored in adipose tissue. High levels of triglycerides are also associated with atherosclerotic risk. Like cholesterol, triglycerides are transported in the plasma bound to proteins as either LDL (15%) or VLDL (85%). When elevated, triglycerides produce a milky-white appearance in the plasma, a condition called hyperlipidemia. Triglyceride levels rise significantly in the blood after sweets or alcohol are ingested and when blood insulin levels are high (hyperinsulinemia). Therefore, when testing for triglyceride levels in the blood, most laboratories require the patient to fast 12 to 14 hours before testing and to refrain from alcohol 2 days before testing. Triglyceride levels can be lowered by exercising and decreasing dietary intake of sweets and alcohol.
The Lipid Panel
The lipid panel of tests consists of TC, HDL, LDL, VLDL, triglycerides, and the TC/HDL ratio. Medical research has established a direct link between blood lipid levels and coronary artery disease that may lead to subsequent myocardial infarcts (heart attacks). Myocardial infarction is the leading cause of death in the United States. An individual’s cardiovascular risk can be determined by comparing the results of the tests in the lipid panel with the individual’s age, weight, height, blood pressure, and smoking habits. Early detection of an at-risk individual can reduce the risk of cardiovascular disease and heart attack if the individual is placed on an appropriate preventive treatment plan consisting of exercise, diet, and medication therapy.
CLIA-WAIVED CHEMISTRY TESTS
Most of the CLIA-waived blood chemistry tests that are performed or observed in ambulatory settings are quantitative analyses. The numeric blood levels of glucose, lipids, glycosylated hemoglobin, electrolytes, BUN, and creatinine are all quantified by electronic photometers. The CLIA-waived fecal occult blood (hidden or not visible to the naked eye) test is a qualitative analysis that simply detects the presence or absence of blood in a fecal specimen.
Principle of Photometers and Spectrophotometers
Each blood chemistry testing method in this section involves collecting a capillary puncture whole blood specimen into a testing device containing premeasured reagents that react with the analyte being tested. The reagents change color as they react to the analyte in question (similarly to the color changes occurring in urine dipsticks). The testing devices are inserted into an electronic analyzer that sends a spectrum of light through the specimen. A photometer located within the analyzer measures the amount of light that passed through the specimen or reflected off the specimen. The intensity of the color changed by the specimen is directly proportional to the concentration of the analyte within the specimen. This equation is known as the Beer–Lambert law.
The chemical analyzers used in blood chemistry testing are highly sensitive photometers and spectrophotometers that directly measure the amount of light passing through the solution (transmittance photometry), indirectly measure the amount of light that the solution absorbs (absorbance photometry), or indirectly measure the amount of light the solution reflects (reflectance photometry). Once the light passes through the solution (or reflects off the specimen), it activates a photodetector that activates a galvanometer (an instrument capable of measuring the intensity of light). The galvanometer sends the information to an electronic program that converts the information into a quantitative digital readout (Fig. 6-8).
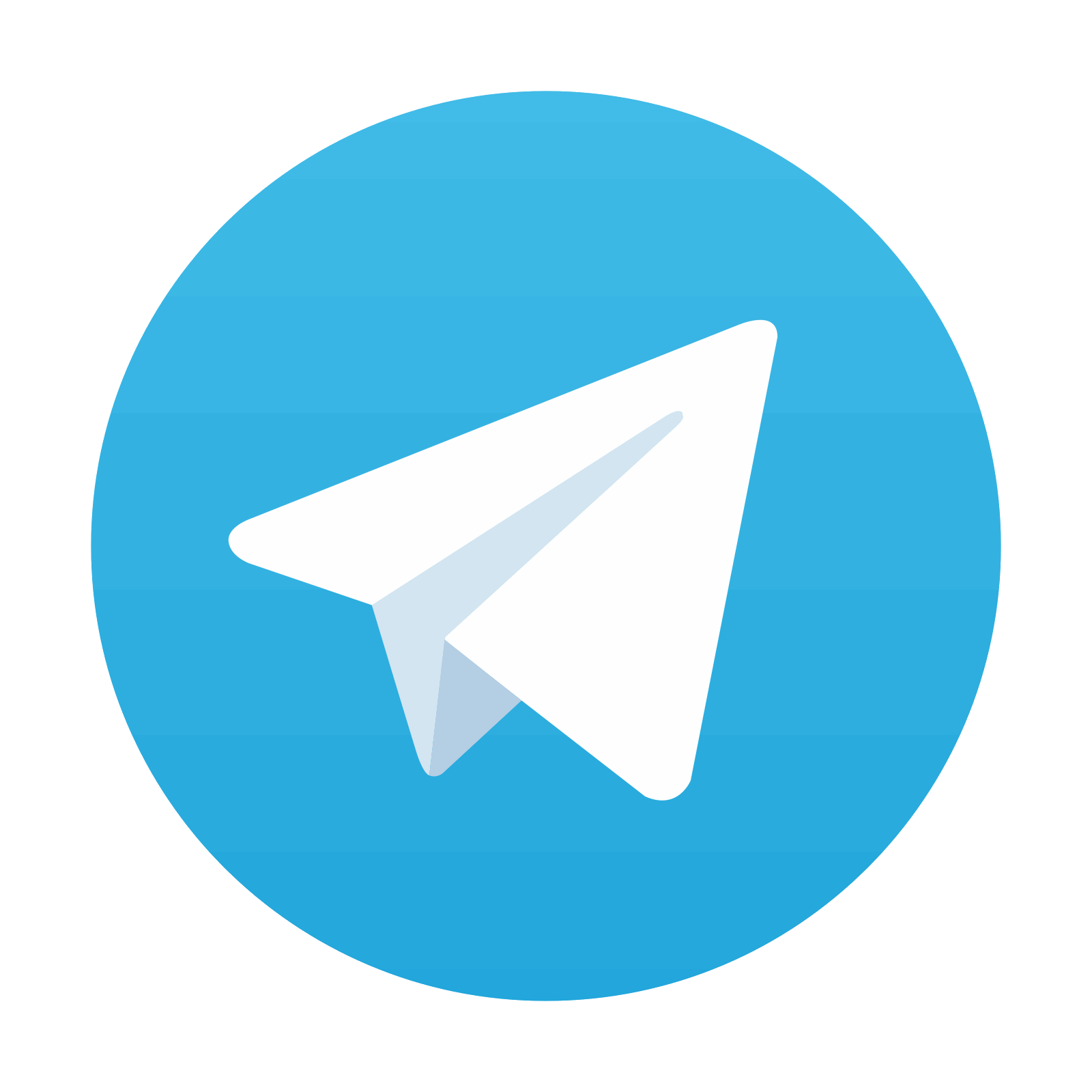
Stay updated, free articles. Join our Telegram channel

Full access? Get Clinical Tree
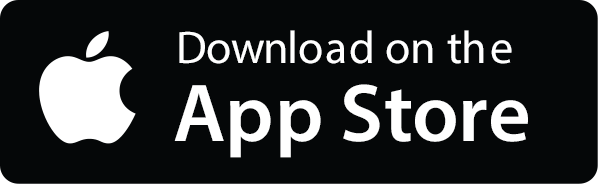
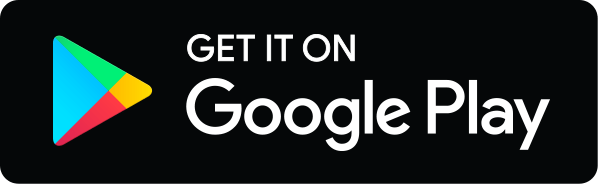
