CHAPTER 11. Fluids and Electrolytes
Judy Hankins, BSN, CRNI®∗
Transport Mechanisms, 178
Fluid and Water Movement, 181
Acid-Base Balance, 187
Principles of Electrolyte Therapy, 194
Summary, 202
The specialty practice of infusion therapy evolved from the need to provide blood, fluids, and electrolytes to maintain life. With all its intricacies, the body relies on water, one of the simplest elemental forms, to sustain life. Water makes up almost two thirds of an adult’s body weight. The relationship and balance of water with electrolytes among the body’s compartments determine human health and well-being.
The continuous biochemical processes of the body maintain the body in a state of equilibrium. Water moves between various spaces and compartments. This movement depends on the types and amounts of solutes in the body. The body strives to match daily excretion with intake. Any alteration in intake or excretion results in an imbalance. Recognition and prevention of, or interventions to correct, these imbalances are among the most important roles the nurse has when caring for the patient requiring infusion therapy.
It is important to understand the purpose that water serves in the human body. It does the following:
• Provides a medium for cellular metabolism
• Helps transport materials into and out of cells
• Is the solvent in which many of the solutes available for cell function are dissolved
• Helps regulate body temperature
• Maintains the physical and chemical consistency of intracellular and extracellular fluids
• Helps digest food through hydrolysis
• Provides a medium for excreting waste
It is estimated that a normal healthy person needs approximately 2600 mL of fluids daily to meet the body’s water requirements. It has also been estimated that the absolute minimum amount of water required by that same healthy person is approximately 1500 mL/day. These facts demonstrate the importance of water in body function to prevent breakdown of the homeostatic regulating mechanisms. An alteration in the body’s normal fluid and electrolyte balance not only affects functions within the fluid compartments but also can eventually affect every body system. Therefore it is vital to understand how water and electrolytes work within the homeostatic framework.
Illness or disease states can easily disrupt the delicate balance of body fluid and its solutes, and treating these imbalances can lead to further complications. With proper knowledge of the intravenous (IV) fluids and electrolytes that are administered daily, it is possible to prevent further complications. It is therefore imperative that the nurse responsible for delivering fluids, electrolytes, and other medications has a thorough working knowledge of normal fluid and electrolyte balances and movements within the body. Understanding the physiological effects of IV fluids and electrolytes in the presence of an imbalance is an important aspect of infusion therapy.
TRANSPORT MECHANISMS
Understanding fluid and electrolyte therapy begins with understanding the intake, output, and utilization of water and electrolytes. Regulating mechanisms include osmosis, diffusion, filtration, and active transport, all of which affect the movement of water and electrolytes within the body. The cell membrane is selectively permeable to substances depending on the construction of the membrane and the ionic charge of particles or solutes attempting to move through it. Each cell is surrounded by a membrane, which is composed of phospholipids consisting of a hydrophilic head and a hydrophobic tail. The hydrophilic head faces the outside of the membrane, allowing for water retention and adhering to other cells. The lipid composition of the hydrophobic tail determines the fluid content of the cell membrane. The protein molecules in the membrane act as carriers to transport lipid-insoluble particles through the membrane while others form ion channels for the exchange of electrolytes (Baumberger-Henry, 2005).
REGULATING MECHANISMS
Osmosis
Osmosis is a passive transport mechanism that allows the movement of water freely through the semipermeable membrane (Figure 11-1). During osmosis, fluid moves in relation to the concentration of solutes; it moves through a membrane from an area of low solute concentration to an area of high solute concentration. This process continues until the solutions on both sides of the membrane are of equal concentration. Osmotic pressure or osmolality is the pressure exerted by the particles, ions, and molecules that moves the water from high concentration to a lower concentration to create an equal concentration (Chernecky, Macklin, Murphy-Ende, 2002). This is due to the number of particles versus the size of the particles, and is influenced primarily by sodium, the main extracellular electrolyte. The process of osmosis depends on how much of the membrane is involved and on certain characteristics of the solution—the temperature, solute solubility, and particularly the concentration.
![]() |
FIGURE 11-1 Osmosis through a semipermeable membrane. (Modified from Lewis, Heitkemper, Dirksen: Medical-surgical nursing: assessment and management of clinical problems, ed 6, St Louis, 2004, Mosby.) |
The number of solutes in a solution is expressed by a unit of measurement called the osmole (osm). Osmolality describes the number of osmoles per kilogram (kg) of water. The liter (L) is the usual unit of measure for water volume. Osmolality can be expressed as a total volume of 1 L of water plus a small volume occupied by the solutes in that liter of fluid. Osmolarity refers to the osmolar concentration in 1 L of solution (mOsm/L). Osmolality is expressed as osm/kg of water; osmolarity is expressed as osm/L. Osmolarity is usually used when referring to fluids outside the body and osmolality for describing fluids inside the body (see Chapter 13). Usually, there is little difference between these two measurements when expressed in clinical practice. The important fact to remember is that osmolality reflects the potential for water movement and water distribution between and within body fluid compartments.
Diffusion
Diffusion, a passive transport mechanism, is the random movement of molecules and ions from an area of higher concentration to an area of lower concentration in a solution, such as the exchange of oxygen and carbon dioxide between the alveoli and capillaries in the lungs (Figure 11-2). Several factors influence how diffusion occurs: membrane permeability, the size and number of the diffusing molecule or ion, and differences in electrical charges (positive and negative) of the ions involved. Temperature affects this process, with increased diffusion occurring as the temperature increases (Chernecky et al, 2002).
![]() |
FIGURE 11-2 Diffusion. (Modified from Lewis SM et al: Medical-surgical nursing: assessment and management of clinical problems, ed 6, St Louis, 2004, Mosby.) |
Filtration
Filtration is the movement together of solutes and water through selectively permeable membranes, always moving from an area of higher pressure to an area of lower pressure. Filtration involves the movement of solutes and water in relation to hydrostatic pressure. It differs from diffusion and osmosis in that diffusion and osmosis are a response to concentrations, and filtration is a response to pressure.
Hydrostatic pressure is generated by the action of the heart pumping blood to the capillaries and is opposed by oncotic pressure that moves the fluid, electrolytes, and waste back into the capillaries. Oncotic pressure is exerted mainly by plasma proteins, specifically albumin, because they are the only dissolved constituents in the plasma and interstitial fluids that do not readily pass through the capillary pores (Guyton and Hall, 2006). It is these proteins that are responsible for the osmotic pressure on both sides of the capillary membrane known as colloid osmotic pressure or oncotic pressure (different from the pressure exerted at the cell membrane). There is also a Donnan effect—caused by sodium, potassium, and other cations held in the plasma by the proteins—that impacts the colloid osmotic pressure (Guyton and Hall, 2006).
The movement of fluid into or out of the capillaries depends on the balance between opposing forces. These forces are referred to as Starling forces when they relate to the movement of water and solutes through capillaries. Again, it is oncotic pressure that opposes capillary hydrostatic pressure (Figure 11-3).
![]() |
FIGURE 11-3 Filtration and hydrostatic pressure. (From Elkin MK et al: Nursing interventions & clinical skills, ed 4, St Louis, 2007, Mosby.) |
Active transport
With osmosis, diffusion, and filtration, fluids passively move from higher concentrations/pressures to lower concentrations/pressures to equalize the concentrations on either side of the semipermeable membrane. There are times when a higher concentration is required on one side of the membrane than on the other.
What makes the uneven concentrations possible is a process called active transport. The active transport process requires energy to move the molecules or ions “uphill” against osmotic pressure to an area of higher concentration (Elkin, Perry, Potter, 2007). There are two types of active transport depending on the energy source: primary and secondary. The energy source for primary active transport is primarily adenosine triphosphate (ATP). The most familiar example of primary active transport is the sodium-potassium pump, allowing a higher concentration of potassium inside the cell while the sodium concentration is higher outside the cell.
There are two forms of secondary active transport including co-transport and counter-transport. With co-transport, the molecule or ion that has a higher concentration outside the cell creates a storehouse of energy that will be used for its transport into the cell. With appropriate conditions, the high concentrated molecules or ions may pull other substances through the cell membrane. The counter-transport process allows the transportation of molecules/ions in a direction opposite to the primary ion with both bound to the same carrier protein (e.g., sodium and calcium) (Guyton and Hall, 2006).
Ionization
An important factor in the movement of fluid in the body is the electrical charge of the particles, or solutes, found in a particular fluid. Solutes are chemical compounds that act in one of two ways when in solution: they either remain whole or develop an electrical charge when dissolved. Compounds that develop an electrical charge break into particles, called ions, in a process called ionization. Such chemical compounds are commonly known as electrolytes. Some electrolytes have a positive charge when placed in water, whereas others develop a negative charge. Ions are dissociated particles of an electrolyte, and they too carry either a positive charge (a cation) or a negative charge (an anion). Cations are electrolytes such as sodium, potassium, calcium, and magnesium. Examples of anions are chloride, bicarbonate, phosphate, and sulfate.
The number of electrically charged ions in a defined amount of fluid is measured as milliequivalents per liter (mEq/L) ( milliequivalent refers to the chemical activity of an element). To achieve electrical balance, the number of cations and anions in a solution (expressed in milliequivalents) must always be equal. Milliequivalents are used as the measure of ions rather than milligrams because milligrams measure only the weight of the electrolyte. Weight gives no indication of the number of ions or the number of electrical charges contained (Figure 11-4) (Chernecky et al., 2002 and Weinstein, 2007).
![]() |
FIGURE 11-4 Major cations and anions of the intracellular and extracellular fluids. The concentrations of Ca 2+ and Mg 2+ represent the sum of these two ions. The concentrations shown represent the total of free ions and complex ions. (From Guyton AC, Hall JE: Textbook of medical physiology, ed 11, Philadelphia, 2006, Saunders.) |
Other substances important in the homeostasis of fluids and electrolytes are glucose, protein, organic acids, oxygen, and carbon dioxide. Although these are not necessarily considered charged particles, they are important in the body’s state of balance.
HOMEOSTATIC MECHANISMS
There are many homeostatic mechanisms that help keep the volume and composition of body fluids within the narrow range defined as normal. Before discussing the regulating organs, there are two principles that are helpful to remember when considering homeostasis in the body.
The first principle states that the overall amount and composition of fluid within each compartment must remain stable and that each compartment must be electrically neutral—that is, the ions must be balanced between anions and cations. There should be no net electrical charge in any compartment at any given time. In addition to maintaining balance, the compartments are constantly exchanging and replacing individual ions. The work required to maintain this balance can use up to 20% of the body’s ATP stores.
The second principle states that the osmolality among the intracellular, interstitial, and intravascular compartments needs to be equal. In osmosis, if there is a difference in the total number of active particles, the water moves into the compartment that contains the higher number of particles. A solution of higher osmolality has a lower water concentration (or higher particle concentration) than a solution of lesser osmolality.
REGULATORY ORGANS
Many organs, including the kidneys, heart and blood vessels, lungs, skin, adrenal glands, hypothalamus, pituitary gland, parathyroid gland, and gastrointestinal (GI) tract, are associated with maintaining the body’s homeostasis.
The kidneys are considered the primary force in homeostasis because the kidneys’ major function is to adjust the amount of water and electrolytes that leave the body to equal the amount that enters the body. The homeostasis is primarily due to the retention and excretion of sodium and water through the regulation of the glomerular filtration rate by the kidneys. There are a variety of hormonal factors involved in this regulation.
The hypothalamus secrets antidiuretic hormone (ADH) and stores it in the posterior pituitary gland. ADH is secreted in response to a decreased blood volume by increasing reabsorption of water through the kidney tubules, resulting in a decreased urine output. In reverse, as blood volume increases, ADH secretion is decreased and water is excreted through the kidneys.
The right atrium releases an atrial natriuretic peptide (ANP) hormone in response to atrial distention of the heart. ANP stimulates the kidney to initiate diuresis of water and sodium, resulting in a decreased intravascular volume (Elkin et al, 2007).
The renin-angiotensin-aldosterone system regulates sodium reabsorption in the renal tubules. The adrenal glands, positioned above the kidneys, consist of two different sections: the adrenal cortex and the adrenal medulla. The adrenal cortex secretes two mineralocorticoid hormones, aldosterone and cortisol, that affect homeostasis. Aldosterone acts on the kidney’s tubular cells, is active in the reabsorption of sodium and water, and can decrease potassium excretion. An increase in the level of aldosterone results in the retention of sodium and the loss of potassium. When sodium is retained, water is also retained. A decreased secretion of aldosterone results in the excretion of sodium and water and the retention of potassium. Cortisol, among its many functions, helps regulate blood pressure by regulating the amount of vasoconstriction necessary to maintain a normal blood pressure. Aldosterone is considered the more powerful of the two hormones, but when cortisol is secreted in large quantities, it can also affect sodium and fluid retention and potassium excretion.
The parathyroid glands are attached to the lateral lobes of the thyroid gland and consist of four to five glands. Parathyroid hormone (PTH), secreted from the parathyroid glands, has an effect on calcium and phosphate concentrations and influences the reabsorption of calcium. An increase in the PTH level increases the serum calcium concentration and lowers the serum phosphate concentration. The reverse is also true; a decreased secretion of PTH lowers the serum calcium and elevates the serum phosphate concentrations. Decreased serum calcium levels stimulate the release of PTH, which in turn increases the serum calcium level. The thyroid gland, which secretes calcitonin, also has an effect on calcium levels in the body. If there is an increase in the serum calcium level, this causes an increased secretion of calcitonin. The effect of calcitonin on calcium is opposite that of PTH.
The heart and blood vessels also play a major role in fluid balance. The pumping action of the heart and the resulting circulation through the blood vessels allow blood to reach the kidneys in sufficient volume to regulate water and electrolytes. Circulation of blood through the kidneys also allows urine to form. Adequate renal perfusion is the foundation for adequate renal function. In addition, there are special stretch receptors in the blood vessels and atrium of the heart whose purpose is to react to hypovolemic states by stimulating fluid retention.
The lungs also contribute to homeostasis through the ventilatory process. Under the control of the medulla oblongata and in response to hydrogen level changes in the blood, the lungs act rapidly to correct metabolic acid-base disturbances. The lungs also regulate oxygen and carbon dioxide levels.
Other organs that affect fluid and electrolyte balance in the body are the skin and the GI tract. Because the skin communicates with our environment, it allows water to escape from the body through perspiration. The GI tract also plays a role in water absorption and reabsorption.
All the organs of homeostasis can be likened to a symphony. To make music, each musician needs to play his or her part in harmony with the other musicians. When they play together, beautiful music can be made. So it is with the organs of homeostasis. Through their interdependencies and interaction, they all work together to meet a common goal: the maintenance of a balanced state in the human body.
FLUID AND WATER MOVEMENT
FLUID COMPARTMENTS
The internal environment of the human body is largely composed of fluid, with water being the most abundant component. Approximately 60% of body weight in an adult is water. The amount of total body water as a percentage of body weight varies somewhat among individuals because of differences in the amount of adipose tissue, which contains little water. The total body water also varies for the young and old with a total of 75% to 80% for newborns and 45% for the older adult (Weinstein, 2007).
Body water is distributed between the intracellular, extracellular, and transcellular compartments. Intracellular fluid is the fluid content of all cells, and represents about two thirds of total body weight.
Extracellular fluid constitutes about one third of total body weight. The extracellular fluid compartment is divided into two separate areas. Fluid found in tissue spaces between blood vessels and cells of the body, including lymph fluid, is referred to as interstitial fluid. The other type of extracellular fluid is plasma, which accounts for approximately 5% of total body weight. Extracellular fluid serves two functions in the body. First, it provides a relatively constant environment for the cells. Second, it helps transport substances to and from the cells. Plasma is a highly specialized fluid in the body and contains red blood cells and protein in large amounts. Plasma proteins have a negative charge that increases the binding of sodium and potassium ions (Guyton and Hall, 2006).
Transcellular fluid is considered a component of the extracellular fluid compartment and is the product of cellular metabolism (Weinstein, 2007). The fluid that is contained in transcellular areas is specialized and is composed of cerebrospinal, pericardial, intraocular, peritoneal, or synovial fluid. GI fluid is also classified as transcellular. It is separated from the blood, but unlike the other fluid compartments, it is separated by capillary endothelial cells and epithelial tissue; therefore it is compartmentalized fluid.
Transcellular fluid is not usually considered when talking about fluid and electrolyte balance; however, because of the special attributes of transcellular fluids, physical symptoms are attributed to their loss.
Although intracellular and extracellular fluids contain the same types of anions and cations, the amounts in which they are found vary between the two compartments. The principal cation in intracellular fluid is potassium. Found in lesser amounts are magnesium, sodium, and calcium. The most prevalent anion in intracellular fluid is phosphate, with smaller amounts of bicarbonate and chloride. The fluid cation found in most abundance in extracellular fluid is sodium; present in much smaller amounts are potassium, calcium, and magnesium. The principal anion of extracellular fluid is chloride, with bicarbonate and phosphate found in smaller amounts.
Therefore major electrolytes in extracellular fluid are sodium as a cation and chloride as an anion. Sodium, chloride, and bicarbonate represent more than 90% of the total amount of solutes found in the extracellular space. Potassium is the major cation in cells, and magnesium is also found in high concentration. The anions in the intracellular compartment are phosphate, sulfate, bicarbonate, and proteinate (Figure 11-5).
![]() |
FIGURE 11-5 Anions and cations of intracellular, interstitial, and intravascular fluids. |
MOVEMENT OF FLUIDS AND ELECTROLYTES
One way that water moves in the body is through osmotic pressure. The distribution of water in the body depends on electrolyte balance and on the distribution of electrolytes and fluids within the intracellular and extracellular compartments. Osmotic gradients are established and maintained by solutes. While water is largely unconfined, electrolytes are usually confined to their respective compartments.
Effects of body fluid concentration
If there is no water movement through a membrane because of an osmotic balance, the solutions on either side of the membrane are isotonic. That is, they exert the same amount of osmotic pressure on each side of the membrane, and they contain the same amount of osmotically active solutes. Isotonic osmolality is approximately 300 mOsm/L. Fluid containing a large number of solutes is considered hypertonic when compared with water containing no solute particles. Conversely, water is considered hypotonic when compared with a fluid containing many solute particles. Osmotic pressure refers to how strongly water can be pulled across a membrane; the strength or pressure of that pull depends on the amount of solutes or molecules in the solution.
Colloids
Electrolytes and other low-molecular-weight substances exert a normal osmotic pressure. Colloids, such as protein and albumin, are nondiffusible substances that have a higher molecular weight. Therefore they exert a higher osmotic pressure, the colloid osmotic or oncotic pressure, which causes water to be pulled into the intravascular space.
Crystalloids
Diffusible substances are referred to as crystalloids. They are important in fluid balance because they can pass through capillary walls, which are the barriers between plasma and interstitial fluid. Crystalloids can expand both intravascular and interstitial spaces. Usually, only about 25% of crystalloids administered remain in the intravascular space, with the rest moving to the interstitial space. Examples of crystalloids are dextrose in water, electrolytes in water, and sodium chloride solutions. See the Focus on Evidence box for the use of crystalloids and colloids for fluid management.
Plasma
Because it contains protein, the plasma component of extracellular fluid responds in a special way to fluid balance. Proteins pull water into the intravascular space.
Sodium
Fluid balance is also regulated by the sodium concentration in plasma, and is minimally influenced by glucose and urea levels in plasma. Sodium and associated anions, primarily bicarbonate and chloride, contribute more than 94% of extracellular fluid solutes (Guyton and Hall, 2006). The osmotic pressure produced by sodium determines the state of cellular hydration. Osmosis occurs when the extracellular fluid contains an electrolyte content lower or higher than normal. For example, if plain water with no electrolyte content was injected into the bloodstream, the red blood cells in the plasma would absorb the water. This would cause the cells to swell and burst. If a solution with high sodium content were injected into the body, the red blood cells would lose water to the salt and the cells would shrink.
FOCUS ON EVIDENCE
Use of Crystalloids Versus Colloids for Fluid Management
• A meta-analysis of 63 eligible trials, 55 presenting mortality data, comparing colloids to crystalloids concluded there is no evidence that resuscitation with colloids reduces the risk of death compared with crystalloids in patients experiencing trauma or burns or following surgery (Parel and Roberts, 2007).
• A meta-analysis including 32 trials analyzed resuscitation and volume expansion in critically ill patients using human albumin. The conclusion revealed that for hypovolemic patients there was no evidence that albumin reduces mortality when compared with saline. Also, there was no evidence that albumin reduces mortality in critically ill patients with burns and hypoalbuminemia. There remains a possibility that there is a select group of critically ill patients in which albumin may be indicated (The Albumin Reviewers, 2004).
• A randomized, double-blinded study involving patients (n = 6997) admitted to the ICU compared the use of 4% albumin or normal saline. The conclusion was that the outcomes for both infusions were similar at 28 days (Finfer et al, 2004).
• In a post hoc study of critically ill patients with traumatic brain injury, fluid resuscitation with albumin was associated with a higher death rate than those resuscitated with saline (Myburgh et al, 2007).
Solute concentration
Solute concentration and the associated osmotic force affect body water distribution. As stated earlier, water moves from an area of lower solute concentration to an area of higher solute concentration, or an area of high osmolality. A change in the osmolality of one compartment always alters the osmolality of the other compartment; stated another way, a change in extracellular fluid compartment osmolality dictates a change in the osmolality of intracellular fluids. The body is striving for homeostasis, and there is water movement until the osmolality values of both compartments are relatively equal.
Fluid pressure
To understand the movement of fluids and solutes within the body, fluid pressures and the amounts of solutes and water in the various compartments must be considered. As water moves to achieve a state of equilibrium, so do pressures exerted on the fluids change to reach a state of equilibrium. There are four pressures to consider when studying water exchange between plasma and interstitial fluid. Movement is determined by blood hydrostatic and colloid osmotic pressures on one side of the capillary membrane and by interstitial fluid hydrostatic and colloid osmotic pressures on the other side. Blood hydrostatic pressure forces fluid out of the capillaries into the interstitial fluid on one side; however, blood colloid osmotic pressure draws fluid back into the capillaries. Interstitial fluid hydrostatic pressure forces fluids out of the interstitial space into the capillaries, and interstitial fluid colloid osmotic pressure moves fluid back out of the capillaries. The net effect is that two of these pressures exert a force in one direction, and two exert pressure in the opposite direction. The difference between these two sets of opposing pressures represents the net or effective filtration pressure. An increase in plasma volume causes an increase in hydrostatic pressure of the blood, which then affects the pressure gradient and the movement of fluid. This results in a condition known as edema. The lymphatic system has an impact on fluid pressure because of its removal of excess fluid and debris from the tissue into the blood circulation. This process creates a slight negative pressure in interstitial fluid (Guyton and Hall, 2006).
Hydrostatic pressure is comparable to the principle of filtration, which is the transfer of water-soluble substances from an area of high pressure to an area of low pressure. Fluid and water-soluble substances are moved by hydrostatic pressure in the vessels. Hydrostatic pressure can be exerted by the pumping action of the heart. The difference in arterial and venous pressures also plays a role in the movement of fluids. Hydrostatic pressure is greater than colloid osmotic pressure at the arterial end of a capillary, which causes fluids to move out of the vessel. Conversely, the osmotic force is greater than the hydrostatic pressure on the venous end of a capillary, enabling fluid to reenter a capillary on the venous end (Guyton and Hall, 2006).
Vascular effects
For the body to function correctly, there must be enough circulating fluid to support osmosis, diffusion, and filtration. Baroreceptors, or stretch receptors, located in the carotid sinuses and the aortic arch, respond to the amount of stretch in the vessel wall. The stretch depends on the volume of blood flowing through the vessel. If there is a drop in arterial pressure, these baroreceptors generate fewer impulses, which in turn causes an accelerated heart rate and an increase in blood pressure. The mechanism controlling water movement between fluid compartments is a rapid-response system. Its primary action is to maintain a normal blood volume, even at the expense of interstitial fluid volume. The interstitial space may expand by several liters over a long period without major changes in the intravascular or intracellular compartments.
Body fluid volume and composition
Age, gender, and the amount of adipose tissue all affect the amount of fluids in the human body. Women have less body fluid than men because men have less body fat. This gender difference in fluid amount is not seen until adolescence but remains throughout life. A newborn’s fluid content is 70% to 80% of body weight. Premature infants have an even higher percentage of fluid, approximately 90% of their body weight. Infants are more susceptible to fluid volume deficit because their bodies have a higher fluid percentage and they have more extracellular fluid. More than half of the newborn’s body fluid is extracellular fluid. In adults, extracellular fluid accounts for only one third of body fluid. Extracellular fluid is more readily lost from the body. By the end of the second year of life, the infant’s total body fluid approaches that of the adult. The adult body composition of 40% cellular and 20% extracellular is reached by puberty. After the age of 40 years, the total fluid percentage of body weight begins to decrease for both men and women (Weinstein, 2007). After 60 years of age, the percentages decrease even more, because with aging there is a decrease of lean body mass and an increase in fat content. Therefore the body holds less water. Changes in fluid volume and composition throughout life are shown in Table 11-1.
Age | Approximate percentage |
---|---|
Infant (birth) | 75-80 |
1 year | 67 |
Adult | 50-60 |
Older adult | 45 |
Water regulation
Intake
The goal is to maintain a state of equilibrium between fluid compartments and between the body’s daily fluid intake and output. The average healthy adult requires from 2000 to 2800 mL of fluid a day. Usually, 1000 to 1500 mL of this total is taken into the body in liquid form. Another 800 to 1000 mL comes from food eaten during the day. Oxidation in body tissues accounts for another 350 mL. Fluid loss amounts to approximately 2500 mL/day. When the body is functioning correctly, the intake is balanced by the output.
Mechanisms of intake
There are various ways to achieve intake and output of fluids and electrolytes. Water is taken into the body by food or drink. The liquid that is ingested is measured as part of intake. Liquid is also taken into the body through food. Water is formed by oxidation when food is broken down into energy by the body. Oxidation releases water for use in metabolism; approximately 350 mL of water comes from the oxidation process daily. Thirst is controlled by osmoreceptors found in the hypothalamus and by intravascular volume. Thirst is an even more important mechanism in supplying endogenous water to the body and is activated when the total body water content is decreased by about 2%. ADH plays an important role in preventing dehydration and therefore hypertonicity of body fluids.
Thirst mechanism
The thirst mechanism is activated when stimulated by osmoreceptors in the anterior hypothalamus, where antidiuretic hormone (ADH) is synthesized, and in the posterior pituitary gland, where ADH is released (Guyton and Hall, 2006). Plasma osmolality and sodium concentrations are usually kept within a narrow range. The upper limit of this range is determined by the osmotic threshold for thirst, referred to as the threshold for drinking (Guyton and Hall, 2006). When the sodium concentration increases by about 2 mEq/L above the normal level, the physical desire for water increases. The drinking mechanism is stimulated, creating a desire for water so that the extracellular fluid level returns toward normal as water is ingested. Through several processes, thirst is relieved almost immediately after drinking fluid. However, it may take 30 to 60 minutes before the fluid is distributed throughout the body (Guyton and Hall, 2006).
Output
The kidneys, in addition to excreting urine, can adjust the amount of water and electrolytes that leave the body so that it equals the amount of water and electrolytes that enter the body. They have a vital role in fluid and electrolyte balance and in acid-base balance. On average, the kidneys filter approximately 180 L of water in a 24-hour period (Guyton and Hall, 2006). This amount varies according to the fluid intake. The usual amount of fluid output through the kidney is approximately 60 mL/hour (1.5 L/day) (Elkin et al, 2007).
Perspiration
Water and electrolytes can be lost through the skin; these are referred to as sensible losses. Sensible losses, or perspiration, can account for up to 1 to 2 L/hour under hot, dry conditions or with heavy exercise, whereas average general loss is approximately 100 mL/day (Guyton and Hall, 2006). Perspiration is considered a hypotonic fluid; it contains chiefly sodium and potassium. Losses by perspiration vary according to environmental temperature. Body temperature and ambient room temperature also affect the amount of perspiration. The skin also loses water by evaporation, which can be up to 600 mL/day. Evaporation is considered an insensible loss.
Respiration
Approximately 300 mL of water is lost through the lungs in any 24-hour period. This is considered insensible water loss, and the amount lost varies according to the rate and depth of respiration. In addition, the lungs play a role in homeostasis because of their ability to eliminate about 13,000 mEq of hydrogen ions in 24 hours, which is significantly more than the kidneys excrete.
Gastrointestinal tract
Although the GI tract is responsible for 100 to 200 mL of fluid loss daily, it can actually filter up to 8 L of fluid in 24 hours. Much of this fluid, however, is reabsorbed through the small intestine. Greater losses can occur from adverse conditions such as diarrhea, vomiting, or fistula development.
Other mechanisms
Water and electrolytes can be lost through other mechanisms, such as from tears and through feces. Abnormal losses can occur from the use of strong diuretics, which deplete body fluids and electrolytes, or through wound drainage, fever, hyperventilation, mechanical ventilation, and GI tubes.
Antidiuretic hormone
The release of ADH in response to osmotic dehydration is affected by plasma osmolality. Osmoreceptors can detect very small changes in the concentrations of sodium and other solutes in plasma. Sodium concentration is the driving force in ADH secretion (Guyton and Hall, 2006). With a normal plasma osmolality, the secretion of ADH is low enough to permit maximum urinary output. If plasma becomes hyperosmolar, ADH is secreted to maintain maximum water retention in the kidneys, and solutes continue to be excreted in an effort to bring osmolality back to normal. The reverse is also true—in a hypoosmolar state, ADH secretion is diminished, allowing the excretion of water while solutes are retained by the kidney. This process returns the plasma to a more normal osmolality.
Effects of age on intake and output
When considering the intake of fluid into the body and the output of that fluid and associated electrolytes, the effect of age on homeostatic mechanisms should be considered. The elderly may experience up to a 50% reduction in kidney function, which results from a decrease in blood flow to the kidneys. There is also an inability to concentrate urine when the fluid intake is reduced, so the glomerular filtration rate is also decreased. This indicates that the elderly are more susceptible to drug toxicities because of decreased renal function. Cardiac output and stroke volume of the heart are lowered in the elderly. Glands may atrophy, which reduces the ability to eliminate fluid through perspiration and causes some control of body temperature to be lost. There is sometimes a loss of muscle tone of the intestinal tract. Thirst mechanisms may be diminished in the aging person, so the attempt to reach homeostasis based on thirst is then compromised.
Likewise, infants also require special consideration. There is proportionately more water in the extracellular compartment of an infant than in that of an adult. Therefore the infant is more vulnerable to fluid volume deficit. The infant may turn over half of its extracellular fluid daily, whereas adults may change only one sixth of their extracellular volume in the same 24-hour period. This means that the infant has less body fluid in reserve. Infants have a large amount of metabolic waste to excrete because their daily fluid exchange is up to two times greater per unit of body weight than that of an adult. Large volumes of urine are formed each day to excrete all the waste products. Infants have a proportionally higher body surface area than adults, so they have a greater fluid loss potential through their skin. Infants can also suffer greater losses from the GI tract in a relatively shorter period than adults.
FLUID DISORDERS
Homeostatic mechanisms of the body are complex and delicate. Generally, this system has the ability to maintain equilibrium, but sometimes these mechanisms fail and the body can be in a state of fluid deficit or excess.
Fluid volume excess
An increase in extracellular fluid volume is known as fluid volume excess or hypervolemia. The increased volume may occur in the extracellular and the intracellular compartments while the extracellular overload can be with the intravascular or interstitial fluids. Hypervolemia is generally the result of an increase in body sodium concentration, which in turn causes water retention. Hypervolemia may be classified as isotonic, hypotonic, or hypertonic. When both sodium and water are retained, the relative serum sodium concentration remains essentially normal (Pagana and Pagana, 2007). Once an imbalance develops, the body attempts to compensate. This occurs through the release of atrial natriuretic factor, which causes the kidneys to increase the rate of filtration and excretion of sodium and water. There is also a decrease in the aldosterone and ADH levels.
Cause
Fluid volume excess is related to an increase in sodium, water, or a combination of the two. Extracellular fluid overload may be due to an increased intake of sodium through diet or hypertonic fluid administration. It can also be caused by regulatory mechanisms. The kidneys, which help regulate sodium and water levels, may be diseased, leading to sodium and water retention. This is particularly true in the presence of a decreased output. Increased secretion of ADH and aldosterone results in fluid retention.
Another major organ that is a part of the normal regulatory system is the heart. In conditions such as congestive heart failure, the diseased heart cannot circulate the intravascular fluids adequately. This pseudointravascular deficit signals the kidneys to conserve sodium and water, leading to fluid volume excess.
A malfunctioning liver could lead to excessive fluid retention. In cirrhosis, for example, the retention is related to a decreased serum albumin level, which facilitates the loss of intravascular fluids into the interstitial space. Additional fluid may be lost into the peritoneal cavity because of hepatic venous obstruction. Again, this decreased intravascular volume signals the kidney to release more renin, which leads to an increase in the aldosterone level and results in sodium and water retention.
Hypervolemia may occur as a result of an excessive sodium and fluid intake. This is generally caused by the excessive administration of IV solutions, particularly those that contain sodium. Also, the excessive ingestion of sodium contained in food or medications may lead to fluid volume excess; this is especially true in those with a heart or kidney abnormality.
Other possible causes of hypervolemia are the administration of excessive doses of steroids and fluid volume shifts within the body. In the case of steroids, the increased fluid volume is related to sodium and water retention. A shift of interstitial fluid to plasma may occur with the treatment of burns. Often, initial burn treatment includes the administration of large amounts of IV solutions because of a fluid volume deficit. Several days later, there is a shift of fluid from the interstitial space back into the intravascular space, which could lead to hypervolemia.
Intracellular fluid overload may be related to an increased administration of hypotonic IV solutions including 0.45% sodium chloride. An isotonic solution, 5% dextrose in water, can also become hypotonic as the body rapidly absorbs the glucose. The overload may also be due to excessive use of free water from irrigation of enteral or nasogastric tubes. Other causes include the syndrome of inappropriate antidiuretic hormone (SIADH), with increased reabsorption of water, and psychogenic polydipsia, from excessive ingestion of water (Baumberger-Henry, 2005).
Assessment
The signs and symptoms of hypervolemia are related to the location and degree of fluid volume excess and to the rate of onset. A sudden, rapid onset results in more pronounced problems.
Probably the most visible characteristic is edema, which is increased fluid volume in the interstitial space. When edema is present, it is usually most visible in dependent areas, as well as around the eyes. The degree of edema may be determined by applying finger pressure around the ankle and sacral areas. Removing the finger leaves a small indention or pit as the fluid excess becomes more severe.
Weight gain usually accompanies the increased fluid volume. This would not occur, however, if the increase were a shift from another compartment. Weight gain is approximately 1 kg/1000 mL fluid increase (Chernecky et al, 2002).
Fluid may shift to another cavity in the body, primarily the abdominal cavity. Accumulation in the abdominal cavity, known as ascites, is often seen in patients with advanced renal or hepatic disease. It is noted by shortness of breath or decreased cardiac output caused by the increased pressure of the excessive fluid volume.
Another characteristic of hypervolemia is pulmonary edema, which can lead to moist rales, shortness of breath, and wheezing. There may be an increase in blood pressure, distention of the neck veins, slower emptying of the peripheral veins, and a more rapid and bounding pulse rate. Polyuria is present if the kidneys are functioning normally.
Laboratory findings reveal a decreased hematocrit and decreased blood urea nitrogen (BUN) value resulting from hemodilution. If the excessive volume is caused by water retention, the serum sodium level and osmolality decrease. In most cases, the urine specific gravity also decreases. Pulmonary congestion may be shown on chest x-ray examination. Because of a decrease in oxygen transport capabilities with pulmonary edema, the arterial blood gases may show a decreased Pa o2 and Pa co2 and an increased pH.
Treatment
Treatment of fluid volume excess depends on its cause. When the cause cannot be determined, it is necessary to treat the disorder symptomatically. This generally includes restriction of sodium and fluid intake, bed rest, and/or diuretic administration. The diuretic will depend on kidney function, degree of hypovolemia, and potency of the diuretic, and may need to be given by the IV route. There may be special requirements for some patients, such as paracentesis in the case of ascites. Dialysis may also be indicated in the presence of renal disease.
Sodium restriction may extend to the diet and to medications, particularly those containing a sodium salt. There are also a variety of over-the-counter preparations that contain sodium.
Nursing interventions should include monitoring vital signs, shortness of breath, cough, and body weight. Any continued presence of edema should be noted. Intake and output records and electrolyte levels should be monitored, particularly after diuretic administration.
Observations should be clearly documented, including the response to diuretics. All abnormal observations should be communicated to the physician.
Fluid volume deficit
Fluid volume deficit, or hypovolemia, occurs as a result of excessive but relatively equal fluid and electrolyte depletion in the extracellular compartment. The body attempts to compensate for the losses by stimulating thirst, increasing the heart rate, and releasing ADH and aldosterone. If the deficit is severe and not corrected in a timely manner, it could lead to renal failure and death. As with fluid volume excess, a fluid volume deficit can be classified as isotonic, hypotonic, or hypertonic (or osmolar). With isotonic deficit, the loss of water and solutes is equal. In a hypertonic deficit, the loss of fluid is greater than solute loss. Hypotonic deficits, which occur less often than the other types, exhibit a decreased concentration of solute (usually sodium and potassium) in the extracellular fluid (Guyton and Hall, 2006).
Cause
Hypovolemia may result from an abnormal loss of body fluids or inadequate fluid intake, which affects the fluid and electrolyte content. Fluid deficit may be caused by the loss of GI fluids. This may occur through vomiting, diarrhea, suctioning, and fistulas.
The skin is another mechanism for fluid loss. Under normal conditions, fluid is lost through the skin as a means of regulating body temperature. In the presence of a fever, however, there are abnormal, insensible fluid losses. Any type of trauma related to the skin, such as burns and cuts, also facilitates the abnormal loss of fluids. In the case of burns, fluid moves from the vascular to the interstitial space where it surrounds the burned area.
Excessive loss takes place through the renal system. This may be caused by polyuria related to administering osmotic diuretics or concentrated IV solutions and tube feedings. Polyuria may also occur with hyperglycemia and some renal disorders.
Trauma, surgery, and bleeding disorders may result in hemorrhage, which rapidly decreases the intravascular fluid volume. There may also be a decrease in the circulating volume because of third spacing. With this phenomenon, there is a shift of fluid from the circulating volume into a space where it cannot easily be exchanged with fluid in the extracellular space. Because third spacing is only a shifting of fluid, there is no actual fluid loss. The fluid deficit in this case is the result of the decreased circulating volume.
Finally, hypovolemia may occur because of a decreased fluid intake, particularly in the infant and older adult populations. Infants have a larger body surface area and tend to lose more fluid than adults. They depend on others to provide oral fluids. Older adults have a decreased sense of thirst and therefore are less likely to seek fluid replacement. The ability to replace fluid may be further complicated by decreased mobility. Patients who cannot respond to thirst (because they are confused or comatose, for example) are subject to fluid volume depletion.
Assessment
As with fluid volume excess, the signs and symptoms of hypovolemia are related to the degree of the deficit and how fast it occurs. There is a loss of weight as the fluid volume decreases, except in the case of third spacing.
Assessment reveals a decreased central venous pressure, flattened jugular vein while in the supine position, capillary filling time longer than 3 seconds, and slow filling of the hand veins (longer than 3 to 4 seconds) (Elkin et al, 2007). The lower circulating volume leads to decreased blood pressure and possibly postural hypotension. With less volume, there is decreased tissue perfusion, which creates a variety of problems, including muscle weakness, dizziness, lethargy, and confusion. As the body tries to maintain an adequate intravascular volume, the pulse rate increases and becomes weaker. The kidneys try to conserve fluid, so there is a decreased urine output.
Skin turgor should be checked by pinching the skin, which slowly returns to the normal position in the presence of hypovolemia. The tongue, which normally has one furrow, has several small furrows. The eyes appear sunken and the face has a pinched expression.
As fluid loss becomes more severe, the patient may go into shock. The extremities become cool and clammy, diaphoresis occurs, urinary output drops sharply, and the patient may become comatose.
Laboratory findings show an increased BUN level elevated out of proportion to the serum creatinine level (ratio greater than 20:1) (Smeltzer et al, 2008). This is the result of the kidneys conserving water and urea, which follows the water. The hematocrit also increases except when the deficit is caused by hemorrhage. With blood loss, red blood cells and serum are lost in equal amounts. However, as the body attempts to compensate for the fluid deficit, interstitial fluid shifts into the intravascular space and the hematocrit decreases. There is an increase in the urine specific gravity and osmolality. The electrolyte levels, serum osmolality, and acid-base balance vary according to the type of fluid lost and the causative factor.
Treatment
The treatment of hypovolemia includes correcting the cause of the deficit and returning the extracellular fluid to a normal level. If oral fluids cannot replace the deficit, IV therapy should be initiated based on physician orders, patient assessment, and established procedures. An isotonic electrolyte solution such as lactated Ringer’s is generally used to initiate therapy for hypovolemia. The severity of the deficit generally dictates the administration rate. As the fluid is replaced, the IV solution may be changed to one that provides free water. This helps the kidneys excrete wastes.
If the deficit is severe enough, oliguria may be present. In this case, it is important to determine whether the cause is fluid volume deficit or renal disease. This may be accomplished through a fluid challenge test, in which the patient is monitored closely as IV solutions are administered. If the kidneys respond by producing urine, then oliguria is probably the result of fluid volume deficit. When there is no increase in urinary output, the cause of the oliguria is most likely related to renal failure or decreased cardiac function.
During treatment for fluid volume deficit, the patient must be monitored closely. This includes monitoring the urinary output, vital signs, hemodynamic pressures, and body weight. Monitoring laboratory test results can help maintain normal fluid and electrolyte levels. The rate of administration for IV solutions should be monitored to prevent fluid overload. Assessment findings need to be clearly documented and the physician notified of abnormal results.
< div class='tao-gold-member'>
Only gold members can continue reading. Log In or Register a > to continue
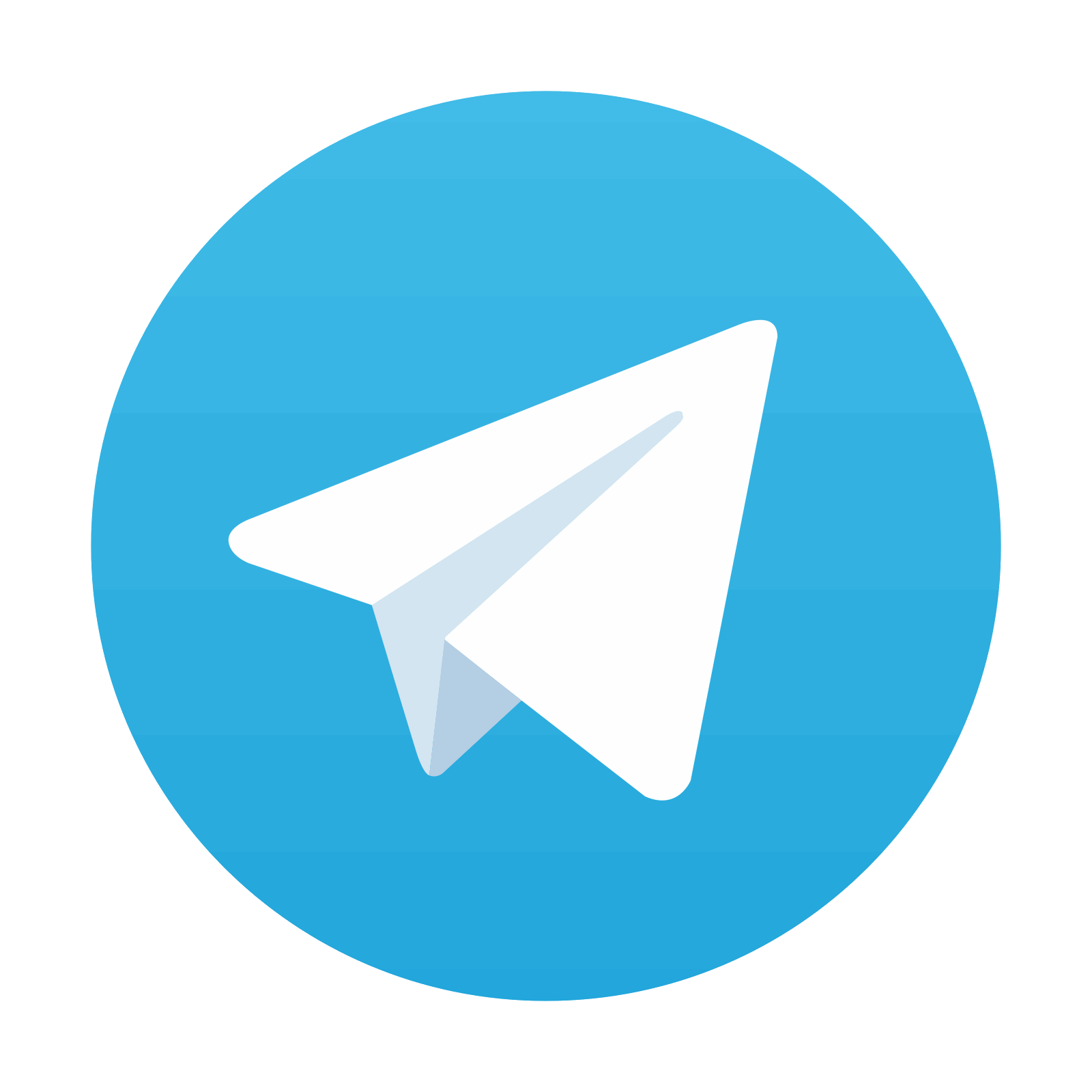
Stay updated, free articles. Join our Telegram channel

Full access? Get Clinical Tree
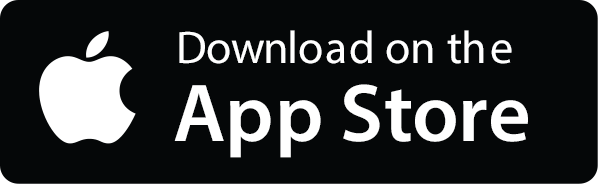
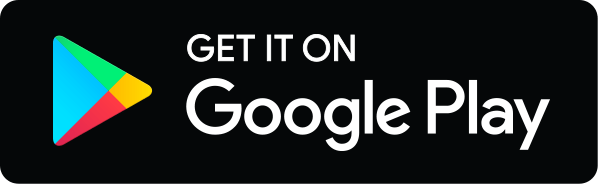
Get Clinical Tree app for offline access
