Nutritional Support for Neuroscience Patients
Joanne V. Hickey
Elizabeth O. Jacobs
Recognition of the critical role of nutrition in health and disease is reflected in the standards of nutritional assessment and therapeutics based on a large body of scientific evidence and knowledge. The registered dietitian (RD) is the recognized clinical expert prepared in nutritional therapeutics who is a member of the interdisciplinary team. For nurses who provide comprehensive care for neuroscience patients, meeting nutritional needs of the patient is a critical component in the recovery process that requires an appropriate knowledge base and collaboration with an RD. Injury, physiologic dysfunction, and stress often change the basic requirements and utilization of nutrients for energy, cellular function, and repair of injured tissue. In addition, a patient with a neurological condition may have neurological deficits, such as an altered level of consciousness or paresis/paralysis of the muscles for arm movement or swallowing, which further complicates ingestion of nutrients. Consideration of these multiple and complex factors, plus the effect of an illness on both the neurological and other systems, requires the expertise and collaborative efforts of the interdisciplinary team. This chapter briefly addresses nutritional requirements and nutritional therapeutics as applied to a neuroscience patient population. The reader is referred to other standard texts, periodicals, and websites for more detailed information.
BASIC NUTRITIONAL REQUIREMENTS
Recommended dietary reference intakes (DRIs), updated in 2010 to include the new recommendations for calcium and vitamin D, are the recognized guidelines for nutritional sufficiency.1 The DRIs are based on scientific evidence and are approved by the National Academy of Sciences and the Institute of Medicine, Food and Nutrition Board. The DRIs outline target intake levels of essential nutrients for healthy people. Nutrient requirements include macronutrients (energy, protein, lipids) and micronutrients (vitamins, minerals). It is important to keep in mind that the nutritional need can differ greatly in patients who are ill, especially if a malabsorption syndrome is present. Recommended daily allowances (RDAs) cited apply only to oral intake unless otherwise noted; there are some variations for parenteral routes of administration.
Essential Nutrient Requirements in Health and Illness
The essential nutrient requirements of adults in health and illness vary. These requirements will be briefly addressed from the perspective of energy, carbohydrate, fat, protein, fluid, electrolyte, vitamin, and trace elements based on the recommendations of the American Society of Enteral and Parenteral Nutrition (A.S.P.E.N.).2 The Institute of Medicine defines estimated energy requirement (EER) for healthy adults as the dietary energy intake predicted to maintain energy balance in a healthy adult of a defined age, gender, weight, height, and level of physical activity consistent with good health.3
In calculating EER age, physical activity, height, and intensity of activity are included. Energy requirements can also be measured with indirect calorimetry that results in the resting energy expended (REE). This indirect method of estimating REE requires special equipment that measures the ratio of carbon dioxide expired to the amount of oxygen inspired. The values collected are used in a
mathematical equation to determine nutritional needs. Indirect calorimetry is not widely used because it is very expensive and requires both specialized machinery and highly trained staff to complete the estimation of needs.
mathematical equation to determine nutritional needs. Indirect calorimetry is not widely used because it is very expensive and requires both specialized machinery and highly trained staff to complete the estimation of needs.
Other options to estimate REE in the hospitalized patient are the Harris-Benedict equations, and more recently, the Ireton-Jones equations.4 The advantage of the Ireton-Jones calculations is that it is normed to sick populations so that the stress of illness has already been calculated into the equation. The Harris-Benedict equations must be adapted to sick patients. There are also two calculation methods to estimate energy needs for the critically ill patient: the Swinamer and Penn State equations. These equations are more dynamic than the previously mentioned equations and are often used in intensive care units because they consider factors that can change daily such as body temperature and minute ventilation.5 A few basic rules are useful for calculating energy requirements as outlined in the A.S.P.E.N guidelines.2 Energy requirements are based on kilocalories per kilogram of body weight with a range of 20 to 35 total kcal/kg body weight/day. Energy comes from carbohydrates and fats. Carbohydrates should be provided at a rate not to exceed 7 g/kg/day, while fat should not exceed a rate of 2.5 g/kg/day. For critically ill patients fat intake is limited to 1 g/kg/day.
CASE STUDY: Choosing the correct equation for determining a patient’s estimated energy needs is an imperative part of the dietitian’s plan of care. Mr. RK is a 57-year-old man who is admitted to the intensive care unit of an acute care hospital with an anoxic brain injury; he requires mechanical ventilator support, and has a nasogastric feeding tube in place. Anthropometrics for RK include the following: height 6′0″ (182.88 cm) and weight 285 lbs (129.5 kg), which converts to a BMI of: 38.6 (obese class II). Mr. RK’s current lab values including electrolytes, all within normal limits.
Question: Given the information above, which would be the best equation to determine Mr. RK’s estimated nutritional needs?
Answer: Mr. RK is clearly critically ill as determined by his ICU placement and ventilator dependence, which narrows down the most appropriate equations to use to three, the Swinamer, Penn State and Ireton-Jones equations. By taking into account that we do not have Mr. RK’s respiratory rate, tidal volume, or body temperature, it can be determined that the most accurate equation to use would be the Ireton-Jones equation. This equation is divided further into two formulas depending on if the patient is spontaneously breathing or not. Mr. RK is not breathing spontaneously; he requires mechanical ventilation and is ventilator dependent. Use the Ireton-Jones equation to determine the kilocalories required per day as follows.
IJEE = 1784 − 11(A) + 5(W) + 244(S) + 239(T) + 804(B).
1784 (constant); A = age in years, W = actual body weight (kg), S = sex (male 1, female 0), T = diagnosis of trauma (present 1, absent 0), B = diagnosis of burn (present 1, absent 0). Using the equation above and inputting Mr. RK’s information gives us the following results:
1784 − 11(57) + 5(129.5) + 244(1) + 239(1) + 804(0) = 2287.5 kcal/day.
Carbohydrates are defined as starches and sugars that are used by the body for energy. When metabolized, 1 gram (g) of carbohydrate yields 4 cal. Carbohydrates are classified as monosaccharides, disaccharides, or polysaccharides. For carbohydrates to be used by the body, they must be broken down into glucose (a monosaccharide), the simplest form of sugar. Glucose is oxidized to release energy and is the source of energy for cerebral cell metabolism. Glucose may also be stored as a reserve in the liver (and in muscle tissue to a lesser degree) in the form of glycogen through a process called glycogenesis. Hydrolysis of glycogen to glucose is called glycolysis (the anabolic enzymatic conversion of glucose to lactate or pyruvate, resulting in energy stored in the form of adenosine triphosphate [ATP], such as occurs in muscles). In addition, excess glucose can be converted into fat and stored in the body as adipose tissue.
Fats occurring as organic substances in the body are called lipids and include triglycerides (fats and oils), phospholipids (e.g., lecithin), and sterols (e.g., cholesterol).6 When 1 g of fat is oxidized, 9 cal are generated. The major function of fats is energy production, although they are also important for the manufacture of other fat-related compounds, such as cholesterol, triglycerides, phospholipids, and lecithin. The major sources of fat in the normal diet are butter, margarine, oil, bacon, meat, fats, egg yolks, nuts, and legumes.
Fatty acids are the basic units of structure in lipids; they can be divided into essential fatty acids (EFA) and nonessential fatty acids. An EFA is one that cannot be manufactured in the body. There are two EFAthat are polyunsaturated; they are linoleic acid (omega-6 fatty acid) and α-linoleic acid (omega-3 fatty acid). EFA play a role in maintaining skin and growth in children. As part of phospholipids, EFA are a component of cell membranes and are precursors of eicosanoids, a group of hormone-like substances involved in inflammation and blood clotting. Prostaglandins, thromboxanes, and leukotrienes are types of eicosanoids related to EFA.6 Although the body cannot make EFA, it does store them so that deficiencies are rare under normal conditions. Essential fatty acid deficiency (EFAD) was first documented in animals in the 1920s. This deficiency was first seen in humans when parenteral nutrition (PN) was developed 4 decades later. EFAD is not usually seen in patients with adequate oral intake or in patients requiring enteral nutrition support as most enteral nutrition formulas contain EFA along with the other nutrients (vitamins, minerals) needed to maintain health. In patients requiring total parenteral nutrition (TPN), EFAD can be prevented by proper lipid administration. If EFAD is present, it is usually seen after 4 or more weeks of fat-free (lipid-free) PN administration.5 Under normal circumstances, nonessential fatty acids do not cause specific deficiency disorders if not ingested in sufficient amounts because they can be manufactured in the body.
All food contains a mixture of saturated, monounsaturated, and polyunsaturated fatty acids. The saturation characteristic is important because it influences the fat’s physical traits and its impact on health. Discussion about the role of omega-3 and omega-6 fatty acids in health is of interest. Omega-3 polyunsaturated oils are found in fish such as salmon, herring, trout, mackerel, and swordfish and also in some plant oils such as canola oil, flaxseeds, walnuts, and hazelnuts whereas omega-6 polyunsaturated oils are found in plant oils such as sunflower, corn, soybean, and cottonseed oils. Both omega-6 and omega-3 fatty acids have immunemodulating properties and both have an effect on inflammation, blood clotting, and lipid levels. However, these are often opposing functions; therefore, it is important that both omega-6 and omega-3 fatty acids are present in the diet in a balanced ratio.
Protein is a class of energy-yielding nutrients composed of carbon, hydrogen, and oxygen plus nitrogen, which is unique to protein. When metabolized, 1 g of protein yields 4 cal. The building blocks of protein are amino acids, the primary function of which is to build and repair body tissue. Almost all nitrogen ingested comes from protein, and most of the nitrogen lost from the body is in the form of nitrogenous end-products found in the urine as urea, creatinine, uric acid, and ammonium salts. A small amount of nitrogen loss occurs through the stool and skin. Nitrogen balance is defined as when protein synthesis and protein breakdown occur at the same rate. Positive nitrogen balance is present when protein synthesis exceeds protein breakdown whereas negative nitrogen balance occurs when protein breakdown exceeds protein synthesis. In the clinical setting, nitrogen balance is determined by calculating protein intake and urine urea nitrogen (UUN) excretion for the same 24-hour period and comparing findings.
Proteins are composed of amino acids. There are 20 common amino acids that are classified as either essential or nonessential. Essential amino acids are necessary for normal growth and development and cannot be manufactured by the body. Nonessential amino acids are defined as amino acids that are not necessary for normal growth and development and can be manufactured by the body. Protein can also be classified as either complete or incomplete. A complete protein is one that contains all essential amino acids in sufficient quantity and appropriate proportions to supply the body’s needs. Proteins of animal origin, such as milk, meat, cheese, and eggs, are examples of complete proteins. An incomplete protein is defined as one that is deficient in one or more essential amino acids. Incomplete proteins are of plant origin and include grains, legumes, and nuts.
The RDA of protein for healthy adults is 0.8 g/kg regardless of gender or age.3 For a patient who is in a catabolic state, the requirement is higher, ranging from 1.2 to 2 g/kg/day.2 In addition, adequate kilocalories must be provided with the protein to ensure proper protein utilization. Note that patients with certain diseases, such as renal failure and end-stage liver disease, may require a protein-restricted diet.
Fluid, electrolytes, vitamins, and trace elements are all essential to health. The typical fluid requirement for adults is 20 to 40 mL/kg/day or 1 to 1.5 mL/kcal of energy expended.7 In the clinical setting, there are great variations dependent on disease (e.g., heart failure, ascites), which may require fluid restriction, and hypermetabolic states (e.g., perspiration, diarrhea), which may require an increased fluid intake. Fluid supplement is individualized based on intake and output measurements and clinical condition. Relying on the thirst mechanism to trigger drinking is unreliable, especially in elderly persons, because there tends to be impairment of thirst, which leads to underhydration. When adequate fluid intake cannot be achieved by the oral route, the enteral or parenteral routes are alternative options that will be discussed later in the chapter. See Chapter 10 for further discussion of fluid imbalance. The major electrolytes in the body are sodium, potassium, chloride, calcium, magnesium, and phosphorus. Electrolytes are involved in metabolic activities and are essential to the normal cellular function. Electrolyte imbalances are common in disease states and require careful physical and laboratory monitoring and correction as part of therapeutic intervention.
Certain vitamins cannot be stored in the body, so that deficiencies can develop if an adequate diet is not consumed daily. Other vitamins can be stored in the body so that deficiencies are not apparent for weeks to months of inadequate vitamin intake. Vitamins are classified as either water soluble or fat soluble. Water-soluble vitamins are vitamin C and the B-complex vitamins (i.e., thiamine, riboflavin, niacin [nicotinic acid], pyridoxine, pantothenic acid, biotin, folic acid, and cobalamin). The fat-soluble vitamins are vitamins A, D, E, and K. Vitamins are needed for recovery and maintenance of cellular and system functions. Although there are recommendations for vitamin and trace mineral requirements for a healthy person on an oral diet, the recommendations can be extrapolated with caution for patients who are ill.2
In summary, this brief review provides a backdrop to consider the nutritional requirements and the impact of neurological conditions and nutritional modifications needed for recovery and maintenance.
METABOLIC CHANGES AND CHARACTERISTICS OF ACUTE STRESS
Profound metabolic changes result in response to acute illness such as neurotrauma, sepsis, and major surgery. Patients have increased energy and nutrient needs and require nutritional support. Because of the complexity of the stress response to injury and subsequent changes in nutrient metabolism, the nutrition prescription and implementation are challenging.8
After acute episodic events such as neurotrauma, sepsis, and major surgery, a shock phase occurs lasting about 24 to 48 hours and is characterized by hypoxia, acidosis, and hypoglycemia, which result in hypovolemia, hypotension, and tissue hypoxia. The sympathetic nervous system (SNS) and the hypothalamic-pituitary-adrenal axis (HPAA) are stimulated and precipitate a number of complex humoral responses. The focus in the shock phase is physiologic stabilization as evidenced by hemodynamic stability and restoration of oxygen delivery and cellular perfusion. The metabolic response follows the physiologic stabilization and has two components, an acute-phase response and the adaptive response. The acute-phase response is a hypermetabolism catabolic state, which peaks 3 to 6 days after initial insult and then gradually subsides to an adaptive phase in 2 to 3 weeks.8, 9 The HPAA and SNS are further activated by the hypermetabolic state triggering the release of cortisol, glucagon, insulin, growth hormone, and catecholamines.10 Concurrently, the immune system is activated through proinflammatory mediators such as prostaglandins and cytokines (interleukin [IL]-1 to -16 and tumor necrosis factor-α [TNF-α]).11 If the patient is well stabilized, he or she transitions into the adaptive phase. The adaptive phase is characterized by anabolism and recovery. However, the transition to the adaptive phase may be delayed by a second insult, such as sepsis or surgery, that could lead to accelerated catabolism and loss of lean body mass; chronic dysfunction of carbohydrate, protein, and fat utilization; profound weight loss; negative nitrogen balance; lactic acidosis; recurring hyperglycemia; malnutrition; and stress starvation.8, 11, 12
Approximately 50% of hospitalized patients exhibit either hypoalbuminemic malnutrition or marasmic-type malnutrition. Hypoalbuminemic (protein) malnutrition, the most common in hospitalized patients, is diagnosed by laboratory findings of reduced serum albumin, transferrin, prealbumin, or retinol-binding protein levels, with albumin being the most commonly used diagnostic. Marasmic malnutrition is characterized by loss of 20% or more of usual body weight over the preceding 3 to 6 months or less than 90% of ideal body weight.10 Malnutrition is associated with increased mortality, nosocomial infections, sepsis, pressure ulcers, and pneumonia. Therefore, attention to nutritional needs is critical for achieving best outcomes.
NUTRITIONAL ASSESSMENT
The nutrition care process is a series of steps that includes nutrition screening, nutrition assessment, and nutrition support.13 All patients should have a nutrition screening by a registered nurse to determine presence of nutritional risk. The Joint Commission (TJC) notes that “a nutritional screening, when warranted by the patients’ needs or condition, is completed within no more than 24 hours of inpatient admission.”14 The nutrition screen is usually part of the admission history and assessment conducted by the nurse within 24 hours of admission. An example of a simple screening tool with established validity and reliability is found in Figure 9-1.15 Patients identified as “at nutritional risk” usually have altered nutritional intake, malabsorption, or altered metabolism problems and require a more comprehensive nutrition assessment.16, 17 Identification of a patient at risk usually triggers the need for an in-depth assessment by an RD. A nutritional assessment is a systematic process of collecting, verifying, and interpreting data to make decisions about the nature and cause of nutrition-related problems. The data set collected depends on the practice setting and patient acuity, but usually includes a thorough history, physical examination, and laboratory studies.2, 13 The RD assumes responsibility for conducting the nutritional assessment, estimating nutritional requirements, and recommending a nutritional support plan. The nurse implements the nutritional plan, provides education for the patient and family, and monitors both response to therapy and complications. Implementation includes safe administration of nutrients by the oral, enteral, or, on occasion, parenteral route.
The prevalence of malnutrition in hospitalized patients is reported at a rate of 30% to 55%.18 Therefore, malnutrition has an impact on patient outcomes and must be carefully addressed. Malnutrition is a common comorbidity that places patients at risk for complications such as pressure ulcers, pneumonia, and other infections; delayed wound healing; failure to wean from a ventilator; and difficulty refeeding. In addition, documented increased length of stay, higher costs, and increased mortality make nutritional support an important therapeutic intervention for improved outcomes.19 Nutritional assessment is the cornerstone for addressing nutritional needs.
Components of the Nutritional Assessment
History
The history includes acute and chronic diseases, medications (including supplements), diagnostic procedures, surgeries, and other therapies (e.g., immunosuppressants).15 It includes a review of systems to collect information about recent weight change, anorexia, nausea, vomiting, diarrhea, and other positive findings. Determine whether there has been unexplained weight loss or weight gain. An involuntary weight loss of 10% or more in 1 year or 5% in 6 months is significant. Reference data for body weight is available from the National Health and Nutrition Examination Surveys (NHANES).20 Information on recent dietary changes and what constitutes normal daily dietary intake is noted. This information can be collected from the medical record, a family member, or the patient.
Physical Examination
Physical inspection includes a comprehensive assessment with attention to special components such as skin (turgor, dryness, edema, bruising, scaling, dermatitis, seborrhea); mucous membranes (dryness, color, bruising, bleeding, especially gums); tongue (swelling, papillary atrophy); eyes (pale or dry conjunctiva, sunken eyeballs); dry, dull-looking hair or hair loss; and muscles (atrophy, wasting). Note the patient’s weight and how it compares with usual weight. This is more useful than actual weight because many critically ill patients retain fluid, so that their current weight may not correlate with current nutritional status.21
Anthropometric measurements such as skinfold thickness and midarm muscle circumference are not useful in critically ill patients because of frequent clinical conditions such as fluid retention and edema. Anthropometric measurements are more useful in less
severely ill patients. When used, triceps or subscapular skinfold thickness (SFT) estimate body fat, and midarm muscle circumference estimates lean body muscle (protein stores). These data are not helpful in patients who are obese or edematous.22
severely ill patients. When used, triceps or subscapular skinfold thickness (SFT) estimate body fat, and midarm muscle circumference estimates lean body muscle (protein stores). These data are not helpful in patients who are obese or edematous.22
Clinical Pearl: Pain assessment is another portion of the physical examination that must not be overlooked. Each patient must be assessed for pain. The most common pain assessments completed by dietitians include checking for pain with chewing or swallowing, any gastrointestinal (GI) pain, or any pain with upper extremities that may inhibit feeding. It may be helpful to visit the patient during meal times. Assessing patients during meal times may bring problems to the clinician’s attention that he or she may have otherwise overlooked. Mealtime observation can provide important information such as if patient is edentulous (with or without dentures) or if he or she needs feeding assistance. This is crucial in determining patient’s overall nutritional risk.
Laboratory Studies
Blood and Urine Studies. A few blood and urine laboratory tests are used to indirectly estimate nutritional status. However, caution needs to be exercised because these studies are greatly affected by organ function (especially liver and renal), infection, inflammation, administration of intravenous fluids, medications such as steroids, and the acute stress response. Serum hepatic transport proteins are considered markers for malnutrition. Albumin, transferrin, and prealbumin, all synthesized in the liver, are used to assess for malnutrition.2 These proteins are referred to as negative acute-phase proteins because they decrease in serum concentration at least 25% due to decreased hepatic synthesis in response to chronic or acute inflammation. By comparison, proteins that increase in concentration at least 25% associated with inflammation and increased hepatic synthesis are called positive acute-phase proteins (C-reactive proteins).23 Serum albumin is a good marker of malnutrition in patients who are not in a stressed state because it is a measure of visceral protein stores.10 It has a long half-life of approximately 21 days and thus is a poor marker of the effects of short-term feeding in hospitalized patients. Transferrin has a half-life of 8 to 10 days and is helpful to monitor response to nutritional support. Prealbumin (half-life, 2 to 3 days) can be useful for monitoring rapid changes in nutritional status. Table 9-1 compares markers of malnutrition.24 Note that these values are unreliable in acute renal or liver failure.
Nitrogen represents the end-product of protein metabolism. Nitrogen balance studies, such as 24-hour UUN collection, compare adequacy of protein intake in relation to protein need with a goal of nitrogen balance (zero).
TABLE 9-1 MARKERS OF MALNUTRITION | ||||||||||||||||||||||||||||||
---|---|---|---|---|---|---|---|---|---|---|---|---|---|---|---|---|---|---|---|---|---|---|---|---|---|---|---|---|---|---|
|
Nitrogen balance = g protein intake/6.25—urinary nitrogen (mostly urea) + fecal losses + obligatory losses (usually 2 to 4 g; corrects for obligatory and insensible protein losses through the skin, lungs, and stool)
The study may be unreliable in patients with severe nitrogen retention conditions (renal or hepatic failure) or if protein intake is incomplete or urine samples for the collection period have been spilled or inadvertently discarded.
The Immune System. Malnutrition is closely correlated with alternations in immune response as measured by total lymphocyte count (TLC) and delayed hypersensitivity reaction (DHR) skin-test antigens.25 The TLC is calculated by multiplying the white blood cell count by the percentage of lymphocytes.26 Infections and immunosuppressant drugs alter the TLC and thus are not helpful in patients with advanced age, immunodeficiency syndromes, radiotherapy, hypoalbuminemia, metabolic stress, infection, cancer, or chronic illness.2 The second test mentioned is DHR; in malnutrition, the reaction is delayed or fails to react to several skin-test antigens. The DHR may not be helpful in the critically ill because of a decreased cellular immunity response (a decrease in the synthesis of antibodies and the antibody response). The C-reactive protein (CRP) is the most commonly identified indicator of inflammation and an elevated CRP is a marker of ongoing acute stress response.
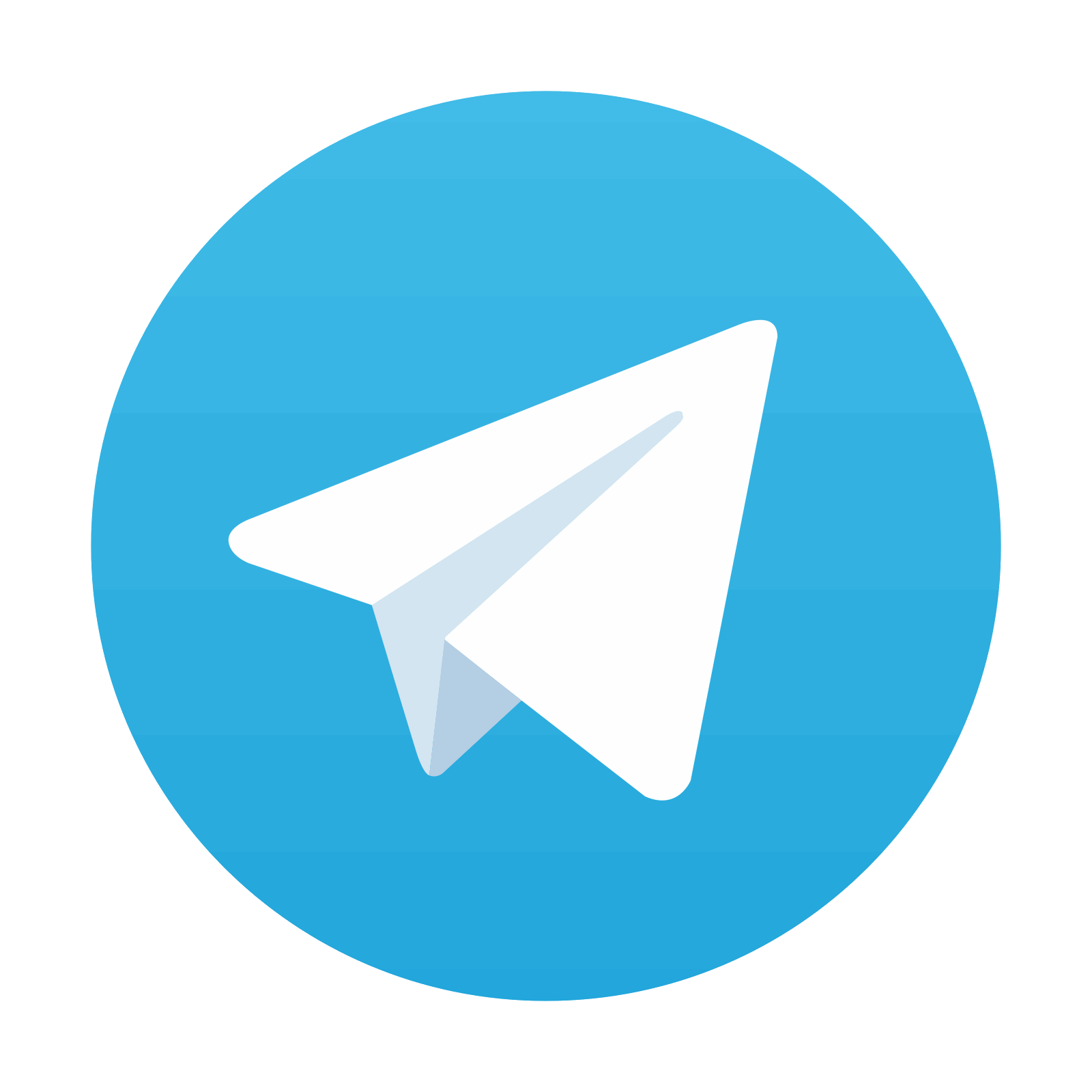
Stay updated, free articles. Join our Telegram channel

Full access? Get Clinical Tree
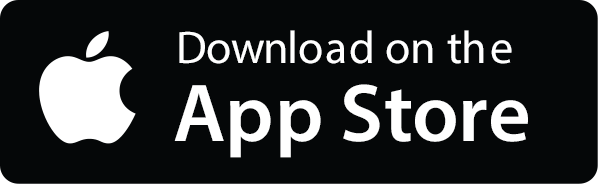
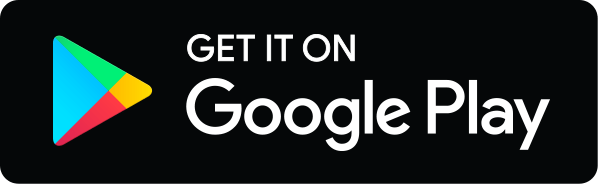
