html xmlns=”http://www.w3.org/1999/xhtml”>
4
Neonatal lung disease: Apnea of prematurity and bronchopulmonary dysplasia
NEONATAL LUNG DISEASE
Lung development occurs throughout pregnancy; development includes airway branching and formation of alveoli (gas exchanging units) and pulmonary blood vessels. Alveoli continue to develop after birth. The lungs, respiratory muscles, and nervous control of breathing are less developed in infants than in older children or adults; this predisposes them to respiratory failure. The chest wall of infants is very compliant (less stiff); consequently, they breathe less efficiently than their older counterparts. Their diaphragm is also at a mechanical disadvantage and may be more prone to fatigue. Furthermore, the neurological pathways that control breathing are immature and as a result, infants are more susceptible to apnea, or cessation of breathing.
Developmental immaturity is more pronounced in preterm infants, placing them at an even higher risk of respiratory problems. In 2006, there were approximately 63,000 births in the United States; 1.5% of them were infants less than 1,500 g (Martin et al., 2008). These infants commonly experience apnea of prematurity. They develop respiratory distress syndrome (RDS) and require supplemental oxygen and mechanical ventilation. The support they require produces injury and chronic lung disease, known as bronchopulmonary dysplasia (BPD). In this chapter, two common neonatal problems, apnea of prematurity and BPD, will be explored.
Apnea of Prematurity
Epidemiology
Apnea of prematurity, or the cessation of breathing, is seen commonly in infants less than 37 weeks’ postmenstrual age (PMA). The literature clearly defines clinically significant apnea in infants as breathing pauses that last for >20 seconds or for >10 seconds if associated with bradycardia (heart rate less than 80 beats/min) or oxygen desaturation (<80–85% by pulse oximetry) (Committee on Fetus and Newborn, 2003). Apnea of prematurity occurs in over 50% of premature infants and in the majority of infants who are less than 1,000 g at birth (Alden et al., 1972). It has been associated with sepsis, intracranial hemorrhage, vaccine administration (Klein et al., 2008), and severe hyperbilirubinemia (Amin, Charafeddine, & Guillet, 2005). Additionally, a genetic predisposition is likely as there is a higher incidence of apnea of prematurity in infants born to first-degree consanguineous parents compared with other infants (Tamim, Khogali, Beydoun, Melki, & Yunis, 2003).
Apnea of prematurity generally resolves by 36–40 weeks’ PMA; however, the more premature the infant, the longer it lasts. In general, the risk of apnea of prematurity seems to resolve by 43–44 weeks’ PMA (Ramanathan et al., 2001). Although preterm infants make a disproportionate percent (18%) of sudden infant death syndrome (SIDS), there is no relationship between apnea of prematurity and SIDS (Committee on Fetus and Newborn, 2003).
Pathophysiology
Apnea of prematurity is due to the immaturity of the preterm infant’s brain and respiratory muscles (Darnall, Ariagno, & Kinney, 2006). Premature infants have an imbalance in the neural inputs that stimulate breathing and the inhibitory pathways that depress it. Developmental abnormalities in the regulation and production of neurotransmitters such as gaminobutyric acid (GABA), adenosine, serotonin, endorphins, and prostaglandin have been suggested as possible contributing causes to apnea of prematurity (Abu-Shaweesh & Martin, 2008).
Premature infants have blunted respiratory responses to hypoxia (decreased delivery of oxygen to tissues) and hypercapnia (elevated carbon dioxide levels); they do not appropriately increase their breathing efforts in response to either. The impaired response to carbon dioxide is more pronounced in infants with apnea of prematurity (Gerhardt & Bancalari, 1984). Infants, both term and preterm, develop apnea when their larynx is stimulated, which is known as the laryngeal chemoreflex. Preterm infants have decreased tone in their upper airway, which makes them more susceptible to upper airway obstruction. Sleep mechanisms are immature and they have a decrease in their ability to arouse in response to respiratory problems, particularly in the prone position (Bhat et al., 2006).
Apnea of prematurity can be central, obstructive, or mixed. Central apnea is the complete cessation of breathing effort; the brain fails to send the appropriate signals to the respiratory muscles to breathe. Obstructive apnea occurs when the infant’s upper airway collapses and air cannot flow in or out of the infant’s mouth; however, the infant continues to try to breathe. Mixed apneas have components of both during an episode.
In response to the apnea, infants often become hypoxic and bradycardic. Lung volumes, specifically functional residual capacity (FRC), are low, which predisposes them to hypoxemia when they develop apnea (Tourneux et al., 2008). Hypoxia itself can produce apnea in preterm infants, which may increase the severity and duration of the apneic episode. Bradycardia is also seen with apnea. The mechanism is not well understood. It may be directly produced by the stimulus that causes the apnea or may be due to enhanced sensitivity to vagal nerve stimulation. In addition, hypoxia itself can also cause bradycardia. Apneas are worse during active rather than quiet sleep.
Events that further depress an infant’s brain function, such as sepsis or intracranial bleeds, may increase the incidence and severity of apnea. For many years, it was believed that gastroesophageal reflux worsens apnea. However, recent studies do not bear out the association (Di Fiore, Arko, Whitehouse, Kimball, & Martin, 2005). Prone position is associated with an increase in central apneas and a decrease in arousals (Bhat et al., 2006).
Signs and Symptoms
Apnea presents with episodes of hypoxemia and bradycardia. It may also be associated with hypotension. Classically, the infant may turn blue, pale, limp, and mottled and makes little or no respiratory effort. Heart rate by cardiac monitoring decreases to less than 80 beats/min. Oxygen saturations by pulse oximetry decrease to less than 85%. Apnea of prematurity may be temporally associated with feeds. Infants may recover by themselves or may require stimulation in order to terminate the episode.
Diagnosis
The diagnosis is made clinically when a lack of respiratory effort is observed along with bradycardia and hypoxemia. In the neonatal intensive care unit (NICU), cardiorespiratory monitoring will pick up bradycardia and hypoxemia. Blood gases are rarely used for diagnosis since cannulation of the artery or vein often causes enough stimulation to terminate the apnea. Secondary causes of apnea should be ruled out such as sepsis and intracranial hemorrhage.
In cases where the diagnosis is more challenging, polysomnography (sleep study) may be used to better delineate the characteristics of the episodes. It can differentiate between central, obstructive, and mixed apneas. During polysomnography, many physiological parameters are monitored: electroencephalogram (EEG), electrocardiogram (ECG), respirations, oxygen saturation levels, carbon dioxide levels, muscle tone, and eye and extremity movements. It can determine the exact duration of an apneic episode and can assess the resultant physiological consequences such as bradycardia, hypoxemia, arousals, and hypercapnia. Monitoring with a pH probe, during the test, can also determine the presence and relationship of gastroesophageal reflux to apnea.
After discharge from the NICU, respiratory monitoring can detect persistent apnea. The most common type of “apnea monitor” is the transthoracic electrical impedance. The apnea monitor has two parts: a belt or electrodes with sensory wires that a baby wears on the chest and a monitoring unit with an alarm. The sensors measure the baby’s chest movement and breathing rate, while the monitor continuously records these rates. These monitors are generally effective in identifying and alarming with central apneas; however, apneas are sometimes identified even though the infant is breathing (false positive), and true apneas are not detected because of background “noise,” such as cardiac beats (false negative).
Complications
Apnea of prematurity can prolong hospitalization of infants and may require more invasive treatment with positive pressure ventilation and medications. Repeated episodes of hypoxia and bradycardia might cause brain injury. Apnea of prematurity has been associated with impairment in neurological development; infants demonstrate lower scores on Bayley Scales of Infant Development and a higher incidence of cerebral palsy and blindness (Hunt et al., 2004; Janvier et al., 2004). Despite the fact that preterm birth is associated with a higher incidence of SIDS, apnea of prematurity does not seem to be an independent risk factor for SIDS.
Management
Mild episodes of apnea can improve without intervention. More significant episodes may require tactile stimulation—that is, stimulating the infant’s limbs, back, or body. Stimulation is a well-recognized treatment for apnea of prematurity. In the past, oscillatory or “bump beds” were used to stimulate the infants. For severe episodes of apnea, the infant may require manual breaths and supplemental oxygen via bag–mask valve ventilation. If the apnea persists, the infant may require support with intubation and positive pressure ventilation. Alternatively, administration of continuous positive airway pressure (CPAP), intermittent positive pressure ventilation, or high-flow gas via nasal cannula may be effective (Pantalitschka et al., 2009). Transfusions with packed red blood cells to treat anemia has been associated with a transient decrease in apneic episodes (Bell et al., 2005).
The mainstay of medical treatment is the use of methylxanthines, primarily caffeine (Bhatt-Mehta & Schumacher, 2003). Methylxanthines stimulate the respiratory and central nervous systems of the preterm infant. In the past, theophylline was widely used. However, caffeine supplanted it as the drug of choice because it is as effective as theophylline but has a longer half-life and fewer side effects. Doxapram, a respiratory stimulant, has been investigated. It is not available in the United States and there is insufficient information about its effects and adverse reactions.
Caffeine is usually discontinued after a period without apnea and bradycardia or at the time when apnea begins to decrease in frequency at 34–36 weeks’ PMA. After discontinuing the medication, most NICUs require the infant to have a period of 3–7 days without apnea and bradycardia before discharging the infant to home. However, in some infants, particularly those born less than 28-weeks gestation, the episodes persist longer which may delay discharge. In those cases, home apnea monitors may be used (Committee on Fetus and Newborn, 2003). Monitoring continues until the infants are 43 weeks’ PMA or after the cessation of extreme episodes, whichever comes last. The monitors should be equipped with an event recorder, which keeps a record of the events. The information can be downloaded and sent to the primary care provider for review. Of note, home cardiorespiratory monitoring has never been proven to decrease the incidence of unexpected deaths in premature infants.
Nursing Care of the Child and Family
The nursing care of the infant with apnea of prematurity must be systematic and comprehensive. It also requires knowledge of the three different types of apneic episodes described earlier in this chapter—central, obstructive, and mixed.
The first crucial component of care is that the nurse should be able to accurately identify periods of apnea so that the appropriate intervention can be initiated. A monitor (cardiac/apnea/pulse oximeter) should be instituted for all infants diagnosed with apnea of prematurity. The leads must be well placed (in a manner such that they visibly capture the infant’s respiratory pattern on the monitor) and the alarms set appropriately (Calhoun, 1996). The heart rate alarm should be based on the infant’s baseline heart rate. The respiratory alarm should notify the caregiver when the infant has been apneic for greater than 20 seconds. Typical settings include a low heart rate of 80–100 beats/min with a 20-second apnea delay (Theobald, Botwinski, Albanna, & McWilliam, 2000). Alarm limits should be designed to provide the nurse time to arrive to the bedside and assess the infant.
Assessment of an apneic episode includes color, respiratory rate and effort, oxygen saturation level, heart rate, and position (Theobald et al., 2000). A thorough assessment will help to determine if the apnea is central, obstructive, or mixed. If the infant is having central apnea, the apnea alarm will probably be the first to sound. Heart rate and oxygen saturation alarms may follow if the apneic episode is prolonged and leads to hypoxia and/or bradycardia. If the infant is experiencing obstructive apnea, the saturation or possibly the heart rate would alarm first as the infant continues to try to breathe. If unsuccessful, the infant will eventually become apneic. In this case, the apnea alarm is a late indicator that the infant is in trouble.
Common triggers for apnea include feeding, suctioning, temperature changes, and immunizations (Calhoun, 1996; Stokowski, 2005; Theobald et al., 2000). The primary intervention for an apneic episode is tactile stimulation, such as foot rubbing (Stokowski, 2005; Theobald et al., 2000). If the infant is not bradycardic or hypoxic, the nurse should observe the infant for a short period of time (approximately 15 seconds) before stimulating the infant to see if he/she will trigger his/her own respirations. If the infant does not spontaneously begin to breathe or if the heart rate or oxygen saturation level drop, the nurse must intervene. If tactile stimulation is insufficient, a more vigorous stimulation, supplemental oxygen, or bag–mask ventilation should be instituted (Stokowski, 2005; Theobald et al., 2000). With obstructive apnea, the infant may require suctioning or repositioning to open his/her airway. Preventive nursing interventions include maintaining a neutral temperature, proper positioning and avoidance of neck flexion, appropriate placement of the nasogastric tube if applicable, slow feedings, avoidance of deep suctioning, and providing periods of undisturbed sleep (Stokowski, 2005; Theobald et al., 2000).
All apnea episodes must be reported and documented. Documentation of apneic episodes is a vital aspect of care. Documentation should include the date and time of the event, the duration of apnea, associated episodes of bradycardia, oxygen saturation level, any precipitating events, and intervention required (Calhoun, 1996).
Apnea of prematurity is also treated pharmacologically with the use of methylxanthines (Calhoun, 1996; Theobald et al., 2000). Therefore, the nurse must monitor the therapeutic and adverse effects of pharmacological therapy. Side effects of methylxanthines include tachycardia, tachypnea, glucose instability, jitteriness, restlessness, tremors, irritability, vomiting, and feeding intolerance (Theobald et al., 2000). Methylxanthines can lead to mild diuresis. Therefore, intake and output should be monitored closely, especially if the infant is on a diuretic (Calhoun, 1996; Gannon, 2000; Theobald et al., 2000).
Theophylline and caffeine are two methylxanthines that have been used in the management of apnea of prematurity. Methylxanthines are thought to stimulate breathing efforts; however, their mechanism of action is not certain (Henderson-Smart & Steer, 2010). A recent review revealed that caffeine and theophylline have similar short-term effects on apnea and bradycardia; however, caffeine is associated with less toxicity, a higher therapeutic ratio, more reliable enteral abosorption, and a longer half-life (Henderson-Smart & Steer, 2010).
Caffeine can be given once a day. It provides stable, predictable plasma concentrations and a wide therapeutic range. Most institutions monitor caffeine levels every 1–2 weeks, while some do not feel monitoring is necessary (Theobald et al., 2000). A therapeutic trough caffeine serum level is 5–25 µg/mL (Gannon, 2000). As previously mentioned, theophylline is not used often because caffeine has increased efficacy and a better safety profile. However, when it is used, it is dosed every 8–12 hours. Serum levels between 7 and 12 µg/mL are generally acceptable (Gannon, 2000). Because theophylline toxicity can occur if levels are slightly higher than the acceptable range, serum levels are checked frequently (Gannon, 2000). Theophylline can affect sleep/wake patterns and can cause gastric irritation. Therefore, it should not be given in the afternoon and stools should be tested for occult blood (Calhoun, 1996; Gannon, 2000; Theobald et al., 2000).
When caring for the infant with apnea of prematurity, it is important to keep the parents informed and well educated. Parents should be updated on their infant’s status as well as on changes in therapy. Information regarding apnea of prematurity and its progression is usually welcomed by parents. Knowing that the apnea is likely related to the infant’s immaturity and will improve over time can help decrease the parents’ level of anxiety. Other educational messages should include positioning, proper feeding techniques, sleep safety measures, avoidance of secondhand smoke, and avoidance of large crowds or exposure to sick contacts. If the infant is experiencing desaturations and/or bradycardia related to positioning, parents should be instructed on the proper positioning that will maintain the infant’s airway. Parents should also be instructed to hold their infant semi-upright while feeding and to observe the infant’s sucking and breathing patterns during the feed. Premature infants may need to have the bottle removed from their mouth to allow them time to rest between long sucking bursts. At home, parents should also be instructed to feed their infants in an area that is well lit so that they can readily assess the infant’s color if an apneic episode occurs (Stokowski, 2005). Parents must be informed that the infant should be maintained in a supine position during sleep and they should avoid having the infant sleep with them in their bed.
Typically, infants are discharged once they are free from apneic episodes for 3–7 days. However, home monitoring can be arranged. The decision to discharge an infant with an apnea monitor is usually institution specific and provider related (Stokowski, 2005). If the infant is discharged home on a monitor, it is important that the parents be informed that the purpose of the monitor is not to prevent SIDS but to provide early recognition of an apneic episode (Stokowski, 2005).
BPD
Epidemiology
BPD is the most common chronic lung disease of infancy (CLDI). It was first described in 1967 by Northway et al. in premature infants who had been exposed to high levels of supplemental oxygen and prolonged mechanical ventilation (Northway, Rosan, & Porter, 1967). Very premature infants are at risk of neonatal RDS and often require support with supplemental oxygen and positive pressure ventilation. BPD occurs primarily in infants who are delivered at a gestational age of less than 30 weeks with a birth weight of less than 1,500 g. BPD occurs in approximately 20% of infants less than 1,500 g (Fanaroff et al., 2007) and one-third of those less than 1,000 g (Walsh et al., 2006). Despite the introduction of antenatal corticosteroids, postnatal surfactant replacement, and more gentle modes of positive pressure ventilation, the incidence of BPD has remained stable throughout the last two decades. Though these interventions have decreased the risk of BPD in older, preterm infants, the younger, more preterm infants are now surviving with an increased incidence of BPD.
The development of BPD has been associated with maternal preeclampsia (Hansen, Barnes, Folkman, & McElrath, 2010), chorioamnionitis (Watterberg, Demers, Scott, & Murphy, 1996), high fluid administration in the early newborn period (Van Marter, Leviton, Allred, Pagano, & Kuban, 1990), patent ductus ateriosus (Brown, 1979), adrenal insufficiency (Watterberg, Gerdes, Gifford, & Lin, 1999), neonatal infections, such as ureaplasma urealyticum (Colaizy, Morris, Lapidus, Sklar, & Pillers, 2007) and cytomegalovirus (Sawyer, Edwards, & Spector, 1987), vitamin A deficiency (Inder, Graham, Winterbourn, Austin, & Darlow, 1998), and intrauterine growth restriction (Bose et al., 2009).
There may be a genetic predisposition to RDS and a subsequent development of BPD. Analysis of twin pairs demonstrates an increased association of BPD in identical versus fraternal twins (Lavoie, Pham, & Jang, 2008). The development of BPD has been correlated with certain genetic polymorphisms or variants (Kwinta et al., 2008; Levit et al., 2009). A family history of asthma has been associated with BPD; however, recent data are not supportive (Evans, Palta, Sadek, Weinstein, & Peters, 1998).
Pathophysiology
Preterm infants have immature lung development. They have a decreased number of branching airways and alveoli. They are deficient in surfactant, which is important for the stability of the alveoli. With insufficient surfactant, the alveoli collapse and develop areas of atelectasis, further impairing gas exchange. As a result, the infants develop respiratory distress and hypoxemia. Prior to the development of surfactant replacement therapy, chest radiographs typically showed a diffuse reticulogranular infiltrate with air bronchograms, and pathology revealed evidence of “hyaline membrane disease.”
BPD results from injury induced by the supplemental oxygen and positive pressure ventilation that the preterm infant requires for survival. In addition, there is evidence that immature lungs of the extremely low birth weight infants do not develop normally after birth, which may further limit pulmonary function.
Both supplemental oxygen and mechanical ventilation can cause injury independently; the combination can cause injury and inflammation within minutes (Pierce & Bancalari, 1995). Oxygen can cause damage by the production of reactive oxygen species and free radicals. Mechanical ventilation causes injury because of the swings in both pressure (barotrauma) and volume (volutrauma). The premature infant is at increased risk because it has a poorly developed antioxidant system to protect against the injury (Frank, 1992). In addition, infections may aggravate the underlying inflammation. The inflammation and injury may hinder the postnatal development of the immature lung.
Classic BPD was associated with typical pathological findings, which included inflammation, necrotizing bronchiolitis, parenchymal fibrosis, smooth muscle hypertrophy, squamous metaplasia, loss of ciliated epithelium, and mucus gland and vascular smooth muscle hypertrophy (Northway et al., 1967). Chest radiographs reflected the heterogeneity of disease with areas of patchy consolidation and fibrosis alternating with hyperinflation.
However, a “new” BPD has recently emerged. Infants are now being delivered at earlier gestational ages, such as 23–26 weeks. At this point in development, the lungs and alveoli are poorly developed; they are little more than conducting tubes with only a small amount of functional alveolar tissue available for gas exchange. The pathology of the new BPD reflects less fibrosis and cellular proliferation; there is more evidence of arrested alveolarization and decreased pulmonary blood vessel development (Kinsella, Greenough, & Abman, 2006).
As a result of the pathological changes, infants have a decrease in their lung compliance and an increase in their airway resistance, both of which increase their work of breathing. The scarring and decreased alveolarization produces hypoxemia. Hypercapnia, or an increase in arterial carbon dioxide levels, may result from both the scarring and respiratory muscle fatigue from the increased demands of breathing. Inflammation also leads to increased airways hyperreactivity—airways smooth muscle constricting in response to a variety of stimuli.
Signs and Symptoms
Infants with RDS present with tachypnea, retractions, cyanosis, and apnea after birth. Infants in respiratory distress are often put on a trial of high-flow oxygen, CPAP, or intermittent positive pressure ventilation via nasal cannula. If they fail this support or develop apnea, they are often intubated and placed on positive pressure ventilation. Some of the infants may improve quickly and may be weaned off positive pressure and oxygen. However, others demonstrate a persistent need for both. As an infant develops chronic lung disease, chest radiographs often develop a bubbly appearance and irregularly dense areas.
Infants with BPD have evidence of impaired oxygenation and increased work of breathing. Without supplemental oxygen, the infant may experience cyanosis, particularly around his/her mouth and mucus membranes. The increased work of breathing may be reflected in tachypnea, nasal flaring, grunting and retractions (subcostal, intercostal, and/or suprasternal), and tachycardia. In the older infant with BPD, respiratory distress may be accompanied by agitation, irritability, and diaphoresis. In overt respiratory failure, infants may demonstrate apnea and a decreased level of consciousness. Older infants with BPD often have crackles because of pulmonary edema or wheezes because of narrowed, hyperreactive airways. Some have upper airway obstruction because of narrowing in the trachea or larynx; this obstruction may be evidenced by stridor on inspiration.
In the NICU, most young infants with BPD receive nutrition with feeding tubes. However, when oral feeds are instituted, infants with BPD can have difficulty feeding and may experience apnea or dyspnea with feeds. They can also have discoordinate swallowing and often have difficulty gaining weight.
Infants with BPD often have problems with oxygenation and ventilation. Pulse oximetry is widely utilized in NICUs. Blood gases may demonstrate hypoxemia and inadequate ventilation with carbon dioxide retention. An arterial blood gas is the gold standard for determining the arterial oxygen level (PaO2) and carbon dioxide (PaCO2). Because an arterial blood gas is technically difficult to obtain, a capillary blood gas is often used. An extremity, such as a finger or toe, is pierced with a lancet; the blood is collected into a thin, small capillary tube. Since it reflects a mixture of venous and arterial blood, the capillary blood sample, it is not good for assessing arterial oxygenation. Venous carbon dioxide levels are higher than those in the arterial blood; thus, it may reflect the “worst-case scenario.”
A chest radiograph usually demonstrates heterogenous lung disease. There are areas of irregularly dense consolidation and hyperinflation. There may be formation of cysts. If the infant is fluid overloaded, then pulmonary edema and cardiomegaly may be seen. Areas of consolidation may represent superimposed pneumonia or atelectasis.
Diagnosis
The diagnosis of BPD in a child who was born premature and received mechanical ventilation was originally made on the basis of three criteria: supplemental oxygen requirement at 28 days of age, persistent abnormalities on chest radiograph, and tachypnea with crackles or rhonchi (Bancalari, Abdenour, Feller, & Gannon, 1979). However, not all infants met the criteria and, subsequently, the definition was refined to correlate better with pulmonary outcomes. BPD is presently diagnosed in infants less than 32 weeks’ gestational age and <1,500 g if they have been on supplemental oxygen for at least 28 days. The severity of disease is stratified by the amount of oxygen that these infants require at 36 weeks’ PMA (Jobe & Bancalari, 2001). It is considered mild if no oxygen is required, moderate if less than 30% is required, and severe if greater than or equal to 30% is required (including the need for positive pressure ventilation).
Complications
There are many complications in infants with BPD both related to their prematurity and their lung disease. The complications are greater in those with severe disease. Approximately half of infants with BPD have mild disease and outgrow their need for oxygen by 36 weeks’ PMA (Ehrenkranz et al., 2005). In contrast, some with severe disease may require long-term positive pressure ventilation administered via a tracheostomy tube. Infants with BPD demonstrate long-term pulmonary impairment (Bhandari & Panitch, 2006). They have frequent respiratory symptoms, airflow obstruction, and airways hyperreactivity (Kennedy et al., 2000; Northway et al., 1990). However, most long-term studies were done on survivors from the 1980s and 1990s who were born at a mean gestational age of 28–29 weeks. The prognosis of those who were born more premature is unknown. The concern is that the decrease in alveolarization may be irreversible and that these infants may be at risk of chronic obstructive pulmonary disease and pulmonary hypertension in the future.
Infants with BPD are at increased risk for rehospitalization because of respiratory tract infections during their first year of life (Lamarche-Vadel et al., 2004). They have hyperreactive airways and are prone to flare-ups of their BPD characterized by labored breathing, hypoxemia, and wheezing. The exacerbations are usually caused by viral respiratory infections such as respiratory syncytial virus (RSV), rhinovirus, influenza, parainfluenza, and human metapneumovirus. While they may have decreased levels of immunoglobulin because of their prematurity and impaired maternal delivery, they do not seem to have an increase in bacterial pulmonary infections.
Infants with BPD have a higher incidence of tracheal abnormalities, such as subglottic stenosis or edema (from prolonged intubation with endotracheal [ET] tubes) and tracheobronchomalacia, or collapsible airways. The stenosis may be so severe that it may require surgical intervention and tracheal reconstruction.
Infants with BPD are at increased risk for both systemic and pulmonary hypertension. Infants with chronic lung disease and pulmonary hypertension have a high risk of mortality. Many infants with BPD have gastroesophageal reflux. It may be easily diagnosed by recurrent vomiting, or it may be subtle and indicated by failure to thrive, aversion to oral feeds, and arching. Infants with BPD may have hematuria from kidney stones, which are caused by chronic diuretic use. They also demonstrate “soft” neurological signs and irritability. BPD is associated with impairment in IQ, cognitive tests and motor skills, lower academic achievement, and an increased need for special education services (Short et al., 2003).
Management
The goal of management is to support the infant’s impaired respiratory status and associated complications, and to provide nutrition in order to allow the infant’s lungs to maximally develop and heal. Supplemental oxygen is given in order to prevent hypoxemia. It is important to keep oxygen saturations greater than 91% in order to allow optimal growth and to prevent pulmonary hypertension. However, there is no improved benefit to “hyperoxia,” that is, keeping the saturations 95–98% instead of 91–94% (Askie, Henderson-Smart, Irwig, & Simpson, 2003). Hypoxemia may develop during oral feeding, increased activity, sleep, or acute illnesses (Wang et al., 2010); if this occurs, an increased concentration of supplemental oxygen during those periods may be required.
For infants with severe BPD, CPAP or high flow via nasal cannula may be required for a prolonged period. Some of these infants may require a tracheostomy in order to administer either CPAP or positive pressure ventilation. As the infants slowly improve, they are gradually weaned off the positive pressure and supplemental oxygen.
Systemic corticosteroids are often administered to wean infants off the ventilator in the NICU. Corticosteroids, like dexamethasone, improve lung compliance, airways resistance, and gas exchange. However, they must be used judiciously because they have been associated with significant side effects and adverse neurological outcomes. In older infants with BPD, corticosteroids may be used for acute exacerbations of BPD associated with wheezing. Inhaled corticosteroids are of uncertain benefit in early BPD and are not routinely used unless reversible lower airways obstruction, wheezing, or bronchodilator-responsive symptoms are documented.
Inhaled bronchodilators have inconsistent effects in infants with early BPD (Pantalitschka & Poets, 2006). In older patients with BPD, they can be used to treat the bronchoconstriction associated with flare-ups of their reactive airway disease.
Diuretics are widely used for infants with BPD in the NICU. In short-term studies, they improve lung function and compliance. However, they have not been proven to decrease the length of hospitalization, the need for ventilatory support, or long-term outcome (Brion, Primhak, & Ambrosio-Perez, 2000). In general, diuretics are continued until the infants are weaned off supplemental oxygen.
Pulmonary hypertension is an often underappreciated entity in infants with BPD. It can be detected by cardiac echocardiography. However, cardiac catheterization may be required to more accurately assess intracardiac pressures. It is important in patients with pulmonary hypertension to prevent hypoxemia and acidosis, which would increase pulmonary vascular resistance and worsen pulmonary hypertension. Sometimes, the use of pulmonary vasodilators, such as sildenafil or bosentran, are necessary (Krishnan, Krishnan, & Gewitz, 2008).
Systemic hypertension can be diagnosed when the infant’s blood pressure is documented to be over the 95 percentile for age. It is important to use the appropriate size cuff and to take the pressure when the infant is quiet. If systemic hypertension is documented, then the cause should be analyzed. In older infants with BPD, it is important to rule out renal artery stenosis, which may have been caused by the presence of an umbilical artery catheter in the early neonatal period. Occasionally, treatment with systemic antihypertensives is necessary.
Nutrition is of paramount importance. Caloric needs are increased because of the infant’s increased work of breathing; energy expenditure may be increased by 30% (Gamarra, 1992). Intake may be limited by inadequate oral intake, vomiting from gastroesophageal reflux, and the need to fluid-restrict the infant. Often nasogastric feeds are necessary to ensure adequate calories. In addition, concentrated formulas are often used to minimize the amount of fluid.
Gastroesophageal reflux is very common in infants with chronic pulmonary disease. Small, frequent, or continuous feeds are often necessary. In some cases, gastroesophageal reflux may be aggravated by milk protein intolerance. Elemental hydrolyzed formulas may be used. In addition, use of acid-blocking medications and promotility agents may be necessary. Some infants require surgical placement of gastric feeding tubes and gastrofundoplication.
Infants with BPD are susceptible to severe bronchiolitis with viral illnesses. RSV and influenza are epidemic in the winter months in the northern hemisphere. Palivizumab (Synagis) is a monoclonal antibody to RSV, which has been shown to decrease hospitalizations and moderate/severe exacerbations. Monthly injections are recommended for all infants with BPD who are <2 years of age at the start of RSV season and have received medical therapy (oxygen, diuretics, bronchodilators, and corticosteroids) in the previous 6 months (American Academy of Pediatrics, 2009). For infants who are at least 6 months of age, influenza vaccine is also recommended.
Nursing Care of the Child and Family
The nursing care of the infant with BPD is multifactorial because of the numerous conditions and complications associated with BPD. Moreover, the nursing care is dependent upon the stage (evolving vs. established) and severity of the disease process. However, whether caring for an infant with BPD during the evolving or established phase, the foundation of care should be grounded in the recognition that the infant has all the developmental issues and needs of a well child in addition to those related to his or her chronic lung disease. Furthermore, he or she is a member of a family, and education and support of that family is crucial to the long-term care of that infant at home. Parents should be considered partners in the care of their infant, and parental involvement should be encouraged (Jackson, 1986; Thomas & Speer, 2008).
Evolving Phase
During the evolving phase when the infant is experiencing respiratory distress, nursing care of the infant is mainly supportive (Charsha, 2009) and focused on minimizing the potential side effects of therapies required to provide adequate gas exchange. Supportive care includes the administration and monitoring of surfactant, corticosteroids, diuretics, bronchodilators, supplemental oxygen, and positive pressure ventilation therapies that are prescribed (Charsha, 2009).
Surfactant is generally administered within minutes after birth into the ET tube of the infant. The ET tube should be suctioned prior to administration. Furthermore, the infant will require position changes to allow gravity to distribute the product to the lungs (Kee, Hayes, & McCuistion, 2009). Due to a limited ability to regulate cerebral blood flow and the risk of precipitating an intraventricular hemorrhage, very low birth weight (VLBW) infants should not be placed in a head-down position to administer the surfactant but should be rotated side to side during the administration. The nurse or respiratory practitioner must assure that the medication is warmed to room temperature before administration. It should not be artificially warmed and it should not be shaken. Depending on the product being used, surfactant is administered in premeasured aliquots. Crackles may be noted after administration of a synthetic surfactant; however, the infant should not be suctioned for at least 2 hours after administration unless prolonged hypoxemia and/or airway obstruction is present. During and after administration, the infant’s respiratory status including inspiratory pressure readings, chest expansion, color, oxygen saturation levels, and arterial blood gases should be monitored closely. Additionally, heart rate, blood pressure, and ET tube patency should be assessed (Kee et al., 2009).
The prolonged use of corticosteroids, especially systemic corticosteroids, are associated with delayed growth, increased blood pressure, osteoporosis, adrenal suppression, and cataracts (Kee et al., 2009). Therefore, these side effects should be monitored for. When given via inhalation, corticosteroids can result in candidiasis. Therefore, if given by a metered-dose inhaler, they should always be used with a holding chamber. Additionally, the infant’s mouth should be rinsed after administration.
Diuretic use requires the monitoring of breath sounds, work of breathing, and urinary output, in addition to the monitoring of potential side effects associated with this class of medications. Side effects of chlorothiazide include hypokalemia, hypercalcemia, and hypomagnesemia (Allen et al., 2003; Kee et al., 2009). Furosemide can result in electrolyte imbalances, such as hypokalemia, hyponatremia, hypocalcemia, hypomagnesemia, and hypochloremia (Kee et al., 2009). Metabolic alkalosis can also occur (Allen et al., 2003; Kee et al., 2009). Because of the effect chlorothiazide and furosemide have on potassium, potassium supplements are often prescribed (Allen et al., 2003; Kee et al., 2009).
Spironolactone is a potassium-sparing diuretic. The main side effect of spironolactone is hyperkalemia (Allen et al., 2003; Kee et al., 2009). Infants who are prescribed spironolactone and a potassium supplement should be monitored closely for hyperkalemia. Furthermore, because of the potential electrolyte imbalances associated with the diuretics commonly used in the management of BPD, all serum electrolytes should be monitored on a regular basis (Allen et al., 2003).
If the infant is receiving bronchodilator therapy, the nurse should evaluate the infant’s therapeutic response by monitoring the infant’s breath sounds, respiratory rate and effort, and oxygen saturation level. The infant receiving bronchodilator therapy should also be monitored for side effects of the medication, including tremors, tachycardia, hyper- or hypotension, dysrhythmias, vomiting, hypokalemia, coughing, wheezing, and bronchospasm (Allen et al., 2003 Kee et al., 2009). In the nonventilated infant, inhaled bronchodilators should always be administered via a face mask, whether given by a metered-dose inhaler or a nebulizer, to help ensure adequate delivery to the airways (Allen et al., 2003).
Infants receiving supplemental oxygen therapy should be monitored with a pulse oximeter. It is important to note that oxygen saturation levels can vary depending on activity level. Therefore, oxygen saturations should be monitored awake, asleep, and during feedings. Saturations consistently above or below the infant’s prescribed range should be documented and discussed with the infant’s provider.
The goals of oxygen therapy are to promote growth and repair of the immature lungs, to provide adequate exercise tolerance, and to decrease the incidence of pulmonary artery hypertension and right ventricular work load (Allen et al., 2003). The range of optimal oxygen saturation in preterm infants is controversial and is still under study; ranges of 85–95% measured by pulse oximetry have been used. Lower target ranges for oxygen saturation are often advocated to prevent retinopathy of prematurity (ROP) (Carlo et al., 2010).
The target oxygen saturation recommendation for those who are very preterm, with early, immature eyes, and without ROP is 90–94%. For those with ROP or those whose retinal vascularization is complete, target oxygen saturation is 95–99% (Allen et al., 2003). Recent findings have not demonstrated significant differences in rates of severe retinopathy or death among infants born between 24- and 27-weeks’ gestation in two oxygen saturation target groups (85–91% and 91–95%). However, death before discharge occurred more frequently and severe retinopathy occurred less often in the lower oxygen saturation group (Carlo et al., 2010). Furthermore, in extremely preterm infants, oxygen saturation levels higher (95–98%) than the standard range (91–94%) have not resulted in significant differences in regard to weight, length, head circumference, or major developmental abnormalities (Askie et al., 2003). Despite the debate, oxygen saturation targets should be monitored closely and individualized for each infant based on his/her condition.
The most common route of supplemental oxygen administration in the nonventilated infant is a nasal cannula. Because the skin of newborns is sensitive, it is important that the nurse or respiratory practitioner monitor for skin breakdown around the cannula site, particularly at the nasal septum and on the infant’s face under the cannula tubing. Protective barriers are often used on the face to prevent breakdown. If the infant has a tracheostomy tube in place and is not ventilated, supplemental oxygen is administered via a tracheostomy collar. It is important for the nurse or respiratory practitioner to assure that the infant is receiving proper humidification via the tracheostomy collar to avoid a decrease in ciliary action, injury to the airway epithelium, and retained mucous secretions (Allen et al., 2003; Sherman et al., 2000).
Infants with BPD who require long-term positive pressure ventilation will frequently undergo a tracheotomy procedure. The severity of lung disease is the most significant factor associated with tracheostomy tube placement in preterm infants (Pereira, MacGregor, McDuffie, & Mitchell, 2003). Although the care of an infant with a tracheostomy tube is beyond the scope of this text, a summary of the nursing care will be provided. Tracheostomy tubes should be changed regularly. Changing the tube on a regular basis facilitates parental education and helps to maintain a patent airway. Tracheostomy tubes are usually changed once a week (Sherman et al., 2000). Tracheostomy tube ties should also be changed on a regular basis. Although there is no consensus regarding the frequency for changing tracheostomy tube ties, they are usually changed at least three times a week and whenever soiled. It is imperative that the ties are secured properly to avoid accidental decannulation and skin breakdown. In general, one should be able to fit one finger between the infant’s neck and the tracheostomy ties (Sherman et al., 2000). The infant with a tracheostomy tube should be suctioned based on clinical need (presence of secretions, increased work of breathing, a drop in oxygen saturation levels, etc.). If there is no evidence of secretions, the infant should be suctioned at least twice a day to assess for patency of the tracheostomy tube. When suctioning, a premeasured technique is recommended to avoid damage to the airway epithelium (Sherman et al., 2000).
The nursing care of an infant with BPD should include monitoring for complications associated with preterm birth and BPD as well as implementing practices that limit or prevent the occurrence of such complications. Common complications include subglottic stenosis, tracheobronchomalacia, systemic hypertension, pulmonary hypertension and cor pulmonale, growth failure, gastroesophageal reflux, and developmental delay.
Subglottic stenosis can occur after an ET intubation. Symptoms include stridor, hoarseness, apnea and bradycaria, cyanosis, and failure to tolerate extubation (Allen et al., 2003). Thus, making sure the ET tube is properly positioned and taking measures to decrease the infant’s level of agitation while intubated are of paramount importance. Tracheobronchomalacia can be acquired or congenital. Symptoms include wheezing that is often unresponsive to bronchodilators (Allen et al., 2003).
The cardiac complications can occur during the evolving phase or the established phase. Indicators include an increase in blood pressure and a decrease in oxygen saturation levels.
The infant with BPD should have an order for daily weight measurement in addition to a weekly length measurement and head circumference. Length can be a predictor of lung growth, which is very important in this patient population. Weight is an important nutritional parameter to monitor; a decrease in weight or poor weight gain can be indicative of other issues, such as increased work of breathing or gastroesophageal reflux. Gastroesophageal reflux can lead to aspiration and bronchospasm (Allen et al., 2003); therefore, the infant should be monitored closely for signs of gastroesophageal reflux and aspiration so that appropriate management can be initiated if required.
Facilitating oral feedings in premature infants is another important nutritional intervention. The nurse should work closely with the occupational therapist to assure this developmental milestone is being addressed. By 28 weeks’ gestation, the infant may demonstrate the simple elements of sucking and swallowing. Bursts of sucking may be evident by 32 weeks and a rhythmic suck, swallow, and breathing pattern by 34 weeks. To maximize oral skills and to facilitate transition to oral feedings, it is recommended that perioral stimulation (gentle touch, i.e., hand grasping and facial stimulation) be provided as tolerated at 23 weeks and that nipple readiness is assessed with nonnutritive sucking at 32–34 weeks followed by a transition to nutritive sucking (Liu et al., 2007). Nonnutritive sucking does not involve the flow of nutrients; it occurs at a rate of two sucks per second and is used to satisfy the basic sucking urge. Nutritive sucking involves the intake of nutrition and occurs at a rate of one suck per second that is constant over the course of the feeding (Harding, 2009). Infants with more mature nonnutritive sucking abilities have demonstrated more advanced oral feeding skills (Bingham, Ashikaga, & Abbasi, 2009). Nonnutritive sucking has also been associated with improved weight gain and improved response to pain (Liu et al., 2007).
From a neurodevelopmental perspective, strategies that promote newborn sleep should be implemented. This includes limiting the amount of narcotics and other medications, such as theophylline, which interrupts normal sleep cycles (Liu et al., 2007).
Established Phase
Once the infant is stable, continuing care is based on the severity of the disease. Some infants will not require any medications or supplemental oxygen, while others may require numerous medications, including diuretics, bronchodilators, and inhaled steroids, in addition to supplemental oxygen and ventilatory support. During this phase, the nurse should continue to administer the necessary therapies and monitor for therapeutic and adverse effects of the medications/therapies as well as the complications associated with BPD.
Optimal growth and prevention of respiratory infections are critical components of care for infants with BPD. Infants with BPD require calorically dense formulas. However, such formulas can contribute to gastroesophageal reflux and loose stools (Allen et al., 2003). Therefore, the nurse should monitor for symptoms of gastroesophageal reflux and loose stools and report them if they occur.
Early on, infants with BPD usually require continuous enteral feedings in order to decrease their energy expenditure. As the infant’s respiratory status improves, bolus feeds can be attempted. Infants receiving enteral feeds should receive oral–motor stimulation in preparation for oral feedings. Once it has been established that the infant’s swallow function has matured, the infant can be fed by mouth. When feeding the infant by mouth, the nurse should monitor for oral–motor dysfunction and report it if it occurs. Oxygen saturation levels should also be monitored during feeds as the infant may require supplemental oxygen.
In addition to teaching parents the importance of keeping the infant away from sick contacts and practicing good hand washing, infants with BPD should receive an annual influenza vaccine if they are 6 months of age or older and palivizumab (Synagis) if they are <24 months of age and have received medical therapy within 6 months of the start of RSV season (American Academy of Pediatrics, 2009). The recommended dose of palivizumab (Synagis) is 15 mg/kg once a month, intramuscularly. The first dose should be administered before the start of RSV season and the treatment should continue for a maximum of 5 months. Palivizumab (Synagis) should not be diluted, shaken, or vigorously agitated. Palivizumab (Synagis) is available as single-dose vials (50 mg/0.5 mL and 100 mg/1 mL) and is preservative free (MedImmune, 2009). Therefore, any unused medication should be discarded.
Preparing for Discharge
Prior to discharge, it is important to determine the parents’ ability and willingness to care for their infant at home as well as the safety and suitability of the home environment (Jackson, 1986). As part of the multidisciplinary team, the nurse is instrumental in identifying family stressors and in advocating for support services such as counseling, sibling support groups, and financial assistance (Jackson, 1986). The nurse also works closely with the care coordinator and medical team to determine the level of services required in the home setting. If skilled nursing services will be involved in the home care of the infant, the family should be provided with anticipatory guidance regarding what the role of the nurse is in the home. If special equipment will be required in the home setting, the home must first be evaluated to determine whether there is enough space for the equipment as well as the appropriate number of electrical outlets to accommodate the necessary equipment. All equipment, supplies, and medications should be secured and in the home prior to discharge (Allen et al., 2003).
Parents learning their infant’s care in preparation for discharge should be encouraged to spend as much time at the hospital as possible. This allows them to observe, learn, and participate in all facets of their infant’s care. During this time, the role of the nurse should include that of an educator. Nurses must provide thorough and focused education regarding all aspects of the infant’s care. Written instructions and materials are also useful for the parents. Documenting the education as well as the parents’ level of understanding and ability are crucial in providing continuity of care. Key educational points for the parents are presented in Table 4.1 (Allen et al., 2003; Bakewell-Sachs, 2002; Jackson, 1986). If the infant has a tracheostomy tube in place, it is recommended that parents participate in an “overnight stay” (this is usually divided into 2- to 12-hour or 3- to 8-hour shifts), after having completed all other components of their training, in order to demonstrate their ability to care for their infant around the clock (Sherman et al., 2000).
Table 4.1 Key educational points for parents going home with an infant who has BPD.
Respiratory assessment—that is, respiratory rate, color, and breathing patterns |
Signs and symptoms of infection—that is, temperature, increased work of breathing, change in appetite or behavior, change in color, odor, or quantity of secretions |
Fluid balance—that is, assessing intake, monitoring and reporting emesis, monitoring wet diapers and reporting decreases in urinary output, assessing for and reporting edema |
Treatments and procedures—that is, airway clearance technique, suctioning, tracheostomy care (cleaning, tracheostomy ties, and tracheostomy changes), preparation of formula, administration of enteral feedings, feeding tube care, care and cleaning of equipment (ventilator, feeding pump, pulse oximeter, etc.), purpose of supplemental oxygen, administration of supplemental oxygen, monitoring of supplemental oxygen |
Medication administration—that is, purpose, dose and schedule, how to draw up medication, how to administer medication (oral, inhaled, via nasogastric or gastrostomy tube), side effects, storage, and identifying need for refill |
Safety issues—that is, keeping infant away from large crowds, keeping infant away from people who are sick, care of supplemental oxygen (no smoking in the home, store tanks upright, etc.), notifying rescue squad and utility and telephone companies that infant has chronic lung disease and requires special equipment, list of emergency numbers to keep posted by the telephone, CPR training |
Anticipatory guidance—that is, developmental issues, potential for rehospitalization |
Follow-up—that is, scheduling appointments (primary care provider, pulmonologist, opthomalogist, gastroenterologist, occupational/physical therapy, etc.), obtaining influenza vaccines and palimizumab |
Travel—that is, travel bag with necessary supplies (feeding supplies, tracheostomy supplies, suction machine, etc.) |
Because gastroesphageal reflux is common in infants with BPD, parents should be instructed on signs and symptoms of gastroesophageal reflux, such as vomiting, irritability and arching after feeding, and failure to thrive. Furthermore, because preterm infants have immature sleep patterns, parents should be provided with anticipatory guidance regarding the fact that these infants have more frequent awakenings over the first few months of life and have less predictable patterns of wakefulness and alertness (Bakewell-Sachs, 2002). Furthermore, infants with BPD have an increased risk for rehospitalization during the first year of life. In a study conducted to describe the rates for rehospitalization during the first year of life among infants with BPD, it was found that 49% of infants were rehospitalized in the first year of life. Those without BPD were hospitalized at a rate of 23% (Smith et al., 2004). Parents should be informed of this fact so that they do not feel they have failed their infant if a readmission becomes necessary. Finally, infants with BPD are at risk of developmental delay. Parents should be provided with the opportunity to learn about premature infant development and behaviors and should be informed of the risk for developmental delay. In a study conducted to examine the effects of BPD and VLBW on the cognitive and academic achievement of a large sample of 8-year-old children, it was found that BPD and duration of supplemental oxygen have long-term adverse consequences on cognitive and academic achievement above and beyond those of being born VLBW (Short et al., 2003).
In addition to the special needs required by infants with BPD, parents should be educated on well child care needs such as feeding, bathing, diaper changes, sleep patterns, development, and immunization schedules (Allen et al., 2003; Bakewell-Sachs, 2002). Developing a daily schedule with the parents for their infant prior to discharge will be helpful as they learn to juggle caring for their infant with their other responsibilities such as work and home life (Allen et al., 2003). Finally, if they have not done so already, parents should identify a primary care provider and preferably meet with that provider before the infant is discharged from the hospital (Bakewell-Sachs, 2002).
REFERENCES
Abu-Shaweesh, J. M., & Martin, R. J. (2008). Neonatal apnea: What’s new? Pediatric Pulmonology, 43, 937–944.
Alden, E. R., Mandelkorn, T., Woodrum, D. E., Wennberg, R. P., Parks, C. R., & Hodson, W. A. (1972). Morbidity and mortality of infants weighing less than 1,000 grams in an intensive care nursery. Pediatrics, 50, 40–49.
Allen, J., Zwerdling, R., Ehrenkranz, R., Gaultier, C., Geggel, R., Greenough, A., et al. (2003). Statement on the care of the child with chronic lung disease of infancy and childhood. American Journal of Respiratory & Critical Care Medicine, 168, 356–396.
American Academy of Pediatrics (2009). Policy statement: Modified recommendations for use of palivizumab for prevention of respiratory syncytial virus infections. Pediatrics, 124, 1694–1701.
Amin, S. B., Charafeddine, L., & Guillet, R. (2005). Transient bilirubin encephalopathy and apnea of prematurity in 28 to 32 weeks gestational age infants. Journal of Perinatology, 25, 386–390.
Askie, L. M., Henderson-Smart, D. J., Irwig, L., & Simpson, J. M. (2003). Oxygen-saturation targets and outcomes in extremely preterm infants. New England Journal of Medicine, 349, 959–967.
Bakewell-Sachs, S. (2002). After the NICU. Comprehensive primary care for preterm infants. Advance for Nurse Practitioners, 10(41–43), 45–46.
Bancalari, E., Abdenour, G. E., Feller, R., & Gannon, J. (1979). Bronchopulmonary dysplasia: Clinical presentation. Journal of Pediatrics, 95, 819–823.
Bell, E. F., Strauss, R. G., Widness, J. A., Mahoney, L. T., Mock, D. M., Seward, V. J., et al. (2005). Randomized trial of liberal versus restrictive guidelines for red blood cell transfusion in preterm infants. Pediatrics, 115, 1685–1691.
Bhandari, A., & Panitch, H. B. (2006). Pulmonary outcomes in bronchopulmonary dysplasia. Seminars in Perinatology, 30, 219–226.
Bhat, R. Y., Hannam, S., Pressler, R., Rafferty, G. F., Peacock, J. L., & Greenough, A. (2006). Effect of prone and supine position on sleep, apneas, and arousal in preterm infants. Pediatrics, 118, 101–107.
Bhatt-Mehta, V., & Schumacher, R. E. (2003). Treatment of apnea of prematurity. Paediatric Drugs, 5, 195–210.
Bingham, P. M., Ashikaga, T., & Abbasi, S. (2009). Prospective study of non-nutritive sucking and feeding skills in premature infants. Archives of Disease in Childhood—Fetal and Neonatal Edition, 95, F194–F200.
Bose, C., Van Marter, L. J., Laughon, M., O’Shea, T. M., Allred, E. N., Karna, P., et al. (2009). Fetal growth restriction and chronic lung disease among infants born before the 28th week of gestation. Pediatrics, 124, e450–e458.
Brion, L. P., Primhak, R. A., & Ambrosio-Perez, I. (2000). Diuretics acting on the distal renal tubule for preterm infants with (or developing) chronic lung disease. Cochrane Database of Systematic Reviews, (3), CD001817.
Brown, E. R. (1979). Increased risk of bronchopulmonary dysplasia in infants with patent ductus arteriosus. Journal of Pediatrics, 95, 865–866.
Calhoun, L. K. (1996). Pharmacologic management of apnea of prematurity. Journal of Perinatology & Neonatal Nursing, 9, 56–62.
Carlo, W. A., Finer, N. N., Walsh, M. C., Rich, W., Gantz, M. G., Laptook, A. R., et al. (2010). Target ranges of oxygen saturation in extremely preterm infants. New England Journal of Medicine, 362(21), 1959–1969.
Charsha, D. S. (2009). Gently caring: Supporting the first few critical hours of life for the extremely low birth weight infant. Critical Care Nursing Clinics of North America, 21, 57–65.
Colaizy, T. T., Morris, C. D., Lapidus, J., Sklar, R. S., & Pillers, D. A. (2007). Detection of ureaplasma DNA in endotracheal samples is associated with bronchopulmonary dysplasia after adjustment for multiple risk factors. Pediatric Research, 61, 578–583.
Committee on Fetus and Newborn (2003). Apnea, sudden infant death syndrome, and home monitoring. Pediatrics, 111, 914–917.
Darnall, R. A., Ariagno, R. L., & Kinney, H. C. (2006). The late preterm infant and the control of breathing, sleep, and brainstem development: A review. Clinics in Perinatology, 33, 883–914, abstract x.
de Gamarra, E. (1992). Energy expenditure in premature newborns with bronchopulmonary dysplasia. Biology of the Neonate, 61, 337–344.
Di Fiore, J. M., Arko, M., Whitehouse, M., Kimball, A., & Martin, R. J. (2005). Apnea is not prolonged by acid gastroesophageal reflux in preterm infants. Pediatrics, 116, 1059–1063.
Ehrenkranz, R. A., Walsh, M. C., Vohr, B. R., Jobe, A. H., Wright, L. L., Fanaroff, A. A., et al. (2005). Validation of the National Institutes of Health consensus definition of bronchopulmonary dysplasia. Pediatrics, 116, 1353–1360.
Evans, M., Palta, M., Sadek, M., Weinstein, M. R., & Peters, M. E. (1998). Associations between family history of asthma, bronchopulmonary dysplasia, and childhood asthma in very low birth weight children. American Journal of Epidemiology, 148, 460–466.
Fanaroff, A. A., Stoll, B. J., Wright, L. L., Carlo, W. A., Ehrenkranz, R. A., Stark, A. R., et al. (2007). Trends in neonatal morbidity and mortality for very low birthweight infants. American Journal of Obstetrics & Gynecology, 196(147), e1–e8.
Frank, L. (1992). Antioxidants, nutrition, and bronchopulmonary dysplasia. Clinics in Perinatology, 19, 541–562.
Gannon, B. A. (2000). Theophylline or caffeine: Which is best for apnea of prematurity? Neonatal Network, 19, 33–36.
Gerhardt, T., & Bancalari, E. (1984). Apnea of prematurity: I. lung function and regulation of breathing. Pediatrics, 74, 58–62.
Hansen, A. R., Barnes, C. M., Folkman, J., & McElrath, T. F. (2010). Maternal preeclampsia predicts the development of bronchopulmonary dysplasia. Journal of Pediatrics, 156(4), 532–536.
Harding, C. (2009). An evaluation of the benefits of non-nutritive sucking for premature infants as described in the literature. Archives of Disease in Childhood, 94, 636–640.
Henderson-Smart, D. J., & Steer, P. A. (2010). Caffeine versus theophylline for apnea in preterm infants. Cochrane Database of Systematic Reviews, CD000273(1). DOI: 10.1002/14651858.CD000273.pub2.
Hunt, C. E., Corwin, M. J., Baird, T., Tinsley, L. R., Palmer, P., Ramanathan, R., et al. (2004). Cardiorespiratory events detected by home memory monitoring and one-year neurodevelopmental outcome. Journal of Pediatrics, 145, 465–471.
Inder, T. E., Graham, P. J., Winterbourn, C. C., Austin, N. C., & Darlow, B. A. (1998). Plasma vitamin A levels in the very low birthweight infant—relationship to respiratory outcome. Early Human Development, 52, 155–168.
Jackson, D. F. (1986). Nursing care plan: Home management of children with BPD. Pediatric Nursing, 12, 342–348.
Janvier, A., Khairy, M., Kokkotis, A., Cormier, C., Messmer, D., & Barrington, K. J. (2004). Apnea is associated with neurodevelopmental impairment in very low birth weight infants. Journal of Perinatology, 24, 763–768.
Jobe, A. H., & Bancalari, E. (2001). Bronchopulmonary dysplasia. American Journal of Respiratory & Critical Care Medicine, 163, 1723–1729.
Kee, J. L., Hayes, E. R., & McCuistion, L. E. (2009). Pharmacology: A nursing process approach (6th ed.). St. Louis, MO: Saunders.
Kennedy, J. D., Edward, L. J., Bates, D. J., Martin, A. J., Dip, S. N., Haslam, R. R., et al. (2000). Effects of birthweight and oxygen supplementation on lung function in late childhood in children of very low birth weight. Pediatric Pulmonology, 30, 32–40.
Kinsella, J. P., Greenough, A., & Abman, S. H. (2006). Bronchopulmonary dysplasia. Lancet, 367, 1421–1431.
Klein, N. P., Massolo, M. L., Greene, J., Dekker, C. L., Black, S., & Escobar, G. J. (2008). Risk factors for developing apnea after immunization in the neonatal intensive care unit. Pediatrics, 121, 463–469.
Krishnan, U., Krishnan, S., & Gewitz, M. (2008). Treatment of pulmonary hypertension in children with chronic lung disease with newer oral therapies. Pediatric Cardiology, 29, 1082–1086.
Kwinta, P., Bik-Multanowski, M., Mitkowska, Z., Tomasik, T., Legutko, M., & Pietrzyk, J. J. (2008). Genetic risk factors of bronchopulmonary dysplasia. Pediatric Respiratory, 64, 682–688.
Lamarche-Vadel, A., Blondel, B., Truffer, P., Burguet, A., Cambonie, G., Selton, D., et al. (2004). Re-hospitalization in infants younger than 29 weeks’ gestation in the EPIPAGE cohort. Acta Paediatrica, 93, 1340–1345.
Lavoie, P. M., Pham, C., & Jang, K. L. (2008). Heritability of bronchopulmonary dysplasia, defined according to the consensus statement of the national institutes of health. Pediatrics, 122, 479–485.
Levit, O., Jiang, Y., Bizzarro, M. J., Hussain, N., Buhimschi, C. S., Gruen, J. R., et al. (2009). The genetic susceptibility to respiratory distress syndrome. Pediatric Respiratory, 66, 693–697.
Liu, W. F., Laudert, S., Perkins, B., Macmillan-York, E., Martin, S., & Graven, S. (2007). The development of potentially better practices to support the neurodevelopment of infants in the NICU. Journal of Perinatology, 27, S48–S74.
Martin, J. A., Kung, H. C., Mathews, T. J., Hoyert, D. L., Strobino, D. M., Guyer, B., & Sutton, S. R. (2008). Annual summary of vital statistics: 2006. Pediatrics, 121, 788–801.
MedImmune (2009). Synagis® (Palivizumab). Package Insert.
Northway, W. H., Jr., Moss, R. B., Carlisle, K. B., Parker, B. R., Popp, R. L., Pitlick, P. T., et al. (1990). Late pulmonary sequelae of bronchopulmonary dysplasia. New England Journal of Medicine, 323, 1793–1799.
Northway, W. H., Jr., Rosan, R. C., & Porter, D. Y. (1967). Pulmonary disease following respiratory therapy of hyaline-membrane disease. Bronchopulmonary dysplasia. New England Journal of Medicine, 276, 357–368.
Pantalitschka, T., & Poets, C. F. (2006). Inhaled drugs for the prevention and treatment of bronchopulmonary dysplasia. Pediatric Pulmonololgy, 41, 703–708.
Pantalitschka, T., Sievers, J., Urschitz, M. S., Herberts, T., Reher, C., & Poets, C. F. (2009). Randomised crossover trial of four nasal respiratory support systems for apnoea of prematurity in very low birthweight infants. Archives of Disease in Childhood. Fetal and Neonatal Edition, 94, F245–F248.
Pereira, K. D., MacGregor, A. R., McDuffie, C. M., & Mitchell, R. B. (2003). Tracheostomy in preterm infants: Current trends. Archives of Otolaryngology—Head & Neck Surgery, 129, 1268–1271.
Pierce, M. R., & Bancalari, E. (1995). The role of inflammation in the pathogenesis of bronchopulmonary dysplasia. Pediatric Pulmonology, 19, 371–378.
Ramanathan, R., Corwin, M. J., Hunt, C. E., Lister, G., Tinsley, L. R., Baird, T., et al. (2001). Cardiorespiratory events recorded on home monitors: Comparison of healthy infants with those at increased risk for SIDS. JAMA, 285, 2199–2207.
Sawyer, M. H., Edwards, D. K., & Spector, S. A. (1987). Cytomegalovirus infection and bronchopulmonary dysplasia in premature infants. American Journal of Diseases in Childhood, 141, 303–305.
Sherman, J. M., Davis, S., Albamonte-Petrick, S., Chatburn, R. L., Fitton, C., Green, C., et al. (2000). Care of the child with a chronic tracheostomy. This official statement of the American Thoracic Society was adopted by the ATS Board of Directors, July 1999. American Journal of Respiratory & Critical Care Medicine, 161, 297–308.
Short, E. J., Klein, N. K., Lewis, B. A., Fulton, S., Eisengart, S., Kercsmar, C., et al. (2003). Cognitive and academic consequences of bronchopulmonary dysplasia and very low birth weight: 8-year-old outcomes. Pediatrics, 112, e359.
Smith, V. C., Zupancic, J. A. F., McCormick, M. C., Croen, L. A., Greene, J., Escobar, G. J., et al. (2004). Rehospitalization in the first year of life among infants with Bronchopulmonary Dysplasia. Journal of Pediatrics, 144, 799–803.
Stokowski, L. A. (2005). A primer on apnea of prematurity. Advances in Neonatal Care, 5, 155–170, quiz 171–174.
Tamim, H., Khogali, M., Beydoun, H., Melki, I., & Yunis, K. (2003). Consanguinity and apnea of prematurity. American Journal of Epidemiology, 158, 942–946.
Theobald, K., Botwinski, C., Albanna, S., & McWilliam, P. (2000). Apnea of prematurity: Diagnosis, implications for care, and pharmacologic management. Neonatal Network, 19, 17–24.
Thomas, W., & Speer, C. P. (2008). Nonventilatory strategies for prevention and treatment of bronchopulmonary dysplasia—what is the evidence? Neonatology, 94, 150–159.
Tourneux, P., Leke, A., Kongolo, G., Cardot, V., Degrugilliers, L., Chardon, K., et al. (2008). Relationship between functional residual capacity and oxygen desaturation during short central apneic events during sleep in “late preterm” infants. Pediatric Respiratory, 64, 171–176.
Van Marter, L. J., Leviton, A., Allred, E. N., Pagano, M., & Kuban, K. C. K. (1990). Hydration during the first days of life and the risk of bronchopulmonary dysplasia in low birth weight infants. The Journal of Pediatrics, 116, 942–949.
Walsh, M. C., Szefler, S., Davis, J., Allen, M., Van Marter, L., Abman, S., et al. (2006). Summary proceedings from the bronchopulmonary dysplasia group. Pediatrics, 117, S52–S56.
Wang, L. Y., Luo, H. J., Hsieh, W. S., Hsu, C. H., Hsu, H. C., Chen, P. S., et al. (2010). Severity of bronchopulmonary dysplasia and increased risk of feeding desaturation and growth delay in very low birth weight preterm infants. Pediatric Pulmonology, 45, 165–173.
Watterberg, K. L., Demers, L. M., Scott, S. M., & Murphy, S. (1996). Chorioamnionitis and early lung inflammation in infants in whom bronchopulmonary dysplasia develops. Pediatrics, 97, 210–215.
Watterberg, K. L., Gerdes, J. S., Gifford, K. L., & Lin, H. M. (1999). Prophylaxis against early adrenal insufficiency to prevent chronic lung disease in premature infants. Pediatrics, 104, 1258–1263.
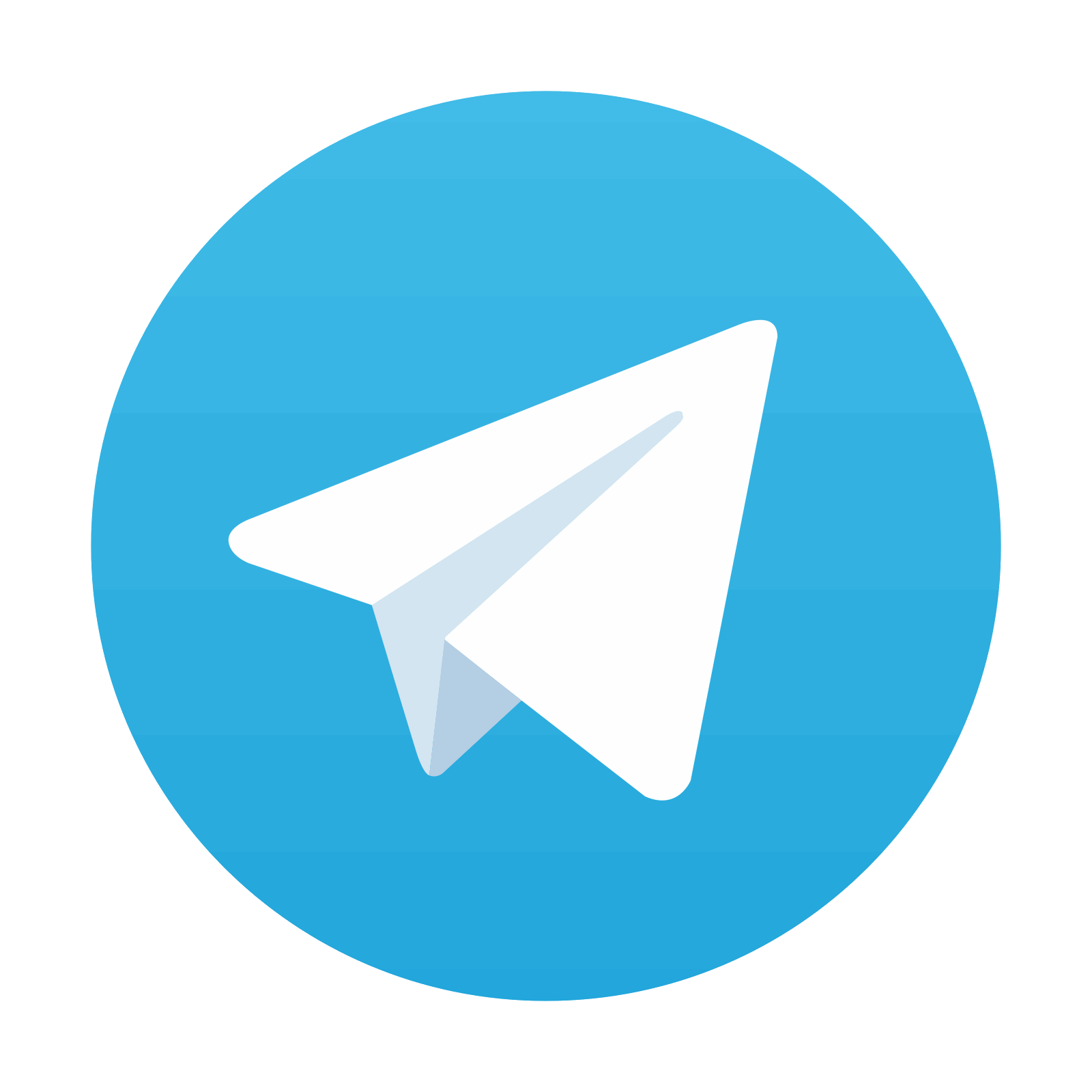
Stay updated, free articles. Join our Telegram channel

Full access? Get Clinical Tree
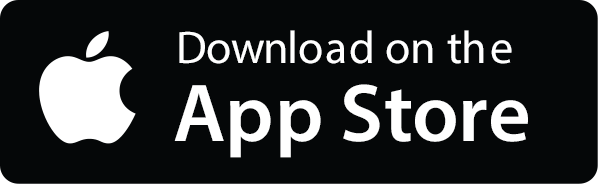
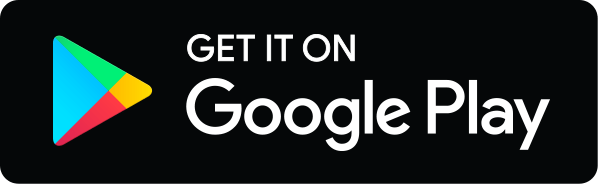