Hereditary and Environmental Influences on Development
Learning Objectives
After studying this chapter, you should be able to:
• Describe the structure and function of normal human genes and chromosomes.
• Give examples of ways genes and chromosomes are studied.
• Describe the transmission of single gene traits from parent to child.
• Relate chromosome abnormalities to spontaneous abortion and to birth defects in the infant.
• Describe genetic components of selected disorders other than those related to reproduction.
• Explain characteristics of multifactorial birth defects.
• Describe the process of genetic counseling.
http://evolve.elsevier.com/McKinney/mat-ch/
Hereditary and environmental forces influence one’s development from before conception until death. The nurse needs a basic knowledge of these forces to understand disorders evident at birth and those that develop later in life.
Hereditary Influences
Hereditary influences on development result from the directions for cellular functions provided by genes that make up the 46 chromosomes in every somatic cell. Abnormal structure or function results if too much or too little genetic material is present in the cells or if an abnormal gene provides incorrect directions. The disorders that result may be merely annoying, or they may be devastating.
Structure of Genes and Chromosomes
A review of the structure of genes and chromosomes aids in understanding how disorders occur. Chromosomes are composed of genes that in turn are composed of deoxyribonucleic acid (DNA) (Figure 10-1).
DNA
DNA is the basic building block of genes and chromosomes. It has three units: (1) a sugar (deoxyribose), (2) a phosphate group, and (3) one of four nitrogen bases (adenine, thymine, guanine, and cytosine).
DNA resembles a spiral ladder, with a sugar and a phosphate group forming each side of the ladder and a pair of nitrogen bases forming each rung. The four bases of the DNA molecule pair with one another in a fixed way, allowing accurate duplication of the DNA during each cell division.
The sequence of base pairs within the DNA determines which amino acids are assembled to form a protein and the order in which they are assembled. Some of these proteins form the structure of body cells; others are enzymes that control metabolic processes within the cell. If the sequence of nitrogen bases in the DNA is incorrect or if some bases are missing or added, a defect in body structure or function may result.
Genes
A gene is a segment of DNA that directs the production of a specific product needed for body structure or function. Humans probably have between 30,000 and 40,000 genes in each cell (Hall, 2011; National Human Genome Research Institute [NHGRI], 2010).
Genes that code for the same trait often have two or more alternate forms (alleles). Many alleles are normal, such as those that code for a person’s blood type. Normal alleles that are common in the population, or polymorphisms (alternate healthy forms of a gene), provide genetic variation and sometimes a biologic advantage. However, mutations often involve change in a gene that alters or harms function, such as those that cause the production of abnormal hemoglobin in sickle cell disease, blood clotting disorders, or cells to grow in an uncontrolled way, causing cancer.
Genes are too small to be seen under a microscope, but through tissue analysis, many can be studied by:
• Measuring the products that the genes direct cells to produce, such as an enzyme or other substance
• Studying the gene’s DNA directly
The Human Genome Project is an international effort begun in 1990 to identify all genes contained in the 46 human chromosomes. The full sequence of human genes was completed in April, 2003 (NHGRI, 2010). Information gained from this project may allow advances such as:
• Basing reproductive decisions on more accurate and specific information than has been available
• Using gene therapy to modify a defective gene
The explosion of knowledge about the genetic basis for many diseases raises many legal and ethical issues for which we do not yet have answers. As our knowledge base grows, new issues are likely to emerge.
Chromosomes
Genes are organized into 46 paired chromosomes in the nucleus of most somatic cells (non-sex body cells). Twenty-two chromosome pairs are autosomes (non-sex chromosomes), and the 23rd pair makes up the sex chromosomes, either an X or a Y. Added or missing chromosomes or structurally abnormal chromosomes are usually harmful.
Mature gametes (reproductive cells) are haploid because they have half the chromosomes (23) of other body cells. One chromosome from each pair is distributed randomly in the gametes, allowing variation of genetic traits among people. When the ovum and sperm unite at conception, the total is restored to 46 paired chromosomes, or diploid.
Cells for full chromosome analysis must have a nucleus and must be living (Jorde, Carey, & Bamshad, 2010). Chromosomes can be studied using any of several types of live cells: white blood cells, skin fibroblasts, bone marrow cells, and fetal cells from the chorionic villi (future placenta) or those suspended in amniotic fluid.
Unlike genes, chromosomes can be seen under the microscope, but only during division of live cells. Specimens must be obtained and preserved carefully to provide enough living cells for chromosome analysis. Temperature extremes, clotting of blood, or adding improper preservatives can kill the cells and render them useless for analysis.
Chromosomes look jumbled when viewed under a microscope (Figure 10-2). Photographing or using computer imaging allows paired chromosomes to be displayed in a karyotype from largest to smallest pairs (Figure 10-3). The karyotype is then analyzed.
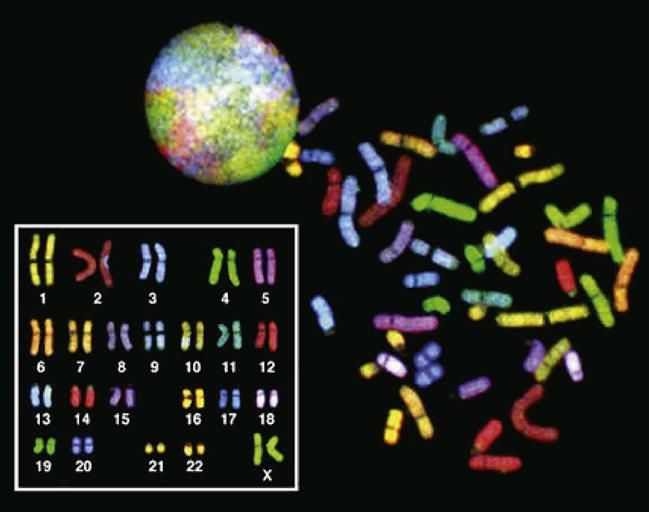
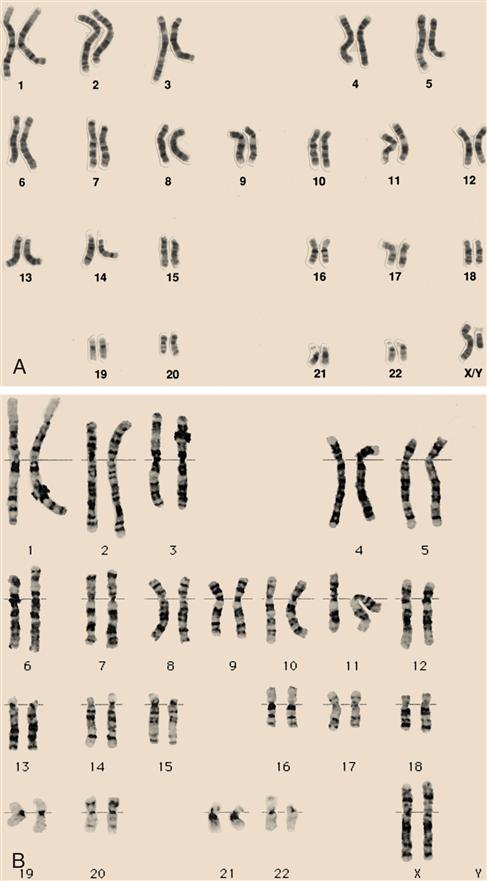
Finer analysis of chromosomes is possible using fluorescent in-situ hybridization (FISH) and spectral karyotyping. FISH uses fluorescent-labeled DNA probes that attach to specific chromosomes and permits testing for added, missing, or rearranged chromosome material that otherwise may not be visible. FISH analysis does not require living cells, unlike other chromosome analyses. Spectral karyotyping (SKY) colors, or “paints,” each chromosome differently to identify small rearrangements, losses, or gains of chromosome material (see Figure 10-3) (Jorde et al., 2010; NHGRI, 2008, 2009, 2010; Nussbaum, McInnes, & Willard, 2007).
Transmission of Traits by Single Genes
Inherited characteristics are passed from parent to child by the genes in each chromosome. These traits are classified according to whether they are dominant (strong) or recessive (weak) and whether the gene is located on one of the autosome pairs or on the sex chromosomes. Both normal and abnormal hereditary characteristics are transmitted by these mechanisms.
Alleles
Because humans have a pair of matched chromosomes (except the sex chromosomes in the male), they have one allele for a gene at the same location on each member of the chromosome pair. The paired alleles may be identical (homozygous) or different (heterozygous).
Some alleles, both normal and abnormal, occur more frequently in certain groups than they do in the population as a whole. For example, the gene that causes Tay-Sachs disease is carried by about 1 of every 27 Ashkenazi Jews in the United States, whose families have their roots in Eastern Europe. However, a higher incidence of Tay-Sachs is found in non-Jewish French-Canadians, Louisiana Cajun people, and the Pennsylvania Amish. An estimated 1 of every 250 people outside this group, including non-Ashkenazi Jews, carries the gene (NHGRI, 2010; National Tay-Sachs and Allied Diseases Association [NTSAD], 2007; Wapner, Jenkins, & Khalek, 2009; Nussbaum et al., 2007). Other disorders that are prevalent in certain ethnic groups are cystic fibrosis (primarily whites of northern European descent) and sickle cell disease (primarily people of African, Mediterranean, Indian, or Middle Eastern descent).
A new trait (harmful, neutral, or sometimes beneficial) may emerge because of a change in the gene within the gamete. The DNA in the gamete is then different from that in the person’s somatic cells. The offspring who receives the new version of the gene will have it in all somatic cells and can transmit it to future generations.
Dominance
Dominance describes how one’s genetic composition is translated into the phenotype, or observable characteristics. In the case of a dominant gene, one copy is enough to cause the trait to be expressed. For example, in the ABO blood system, genes for type A and type B are dominant. Therefore a single copy of either of these genes is enough to be expressed in the person’s blood type.
Two identical copies of a recessive gene are required for the trait to be expressed. The gene for blood group O is recessive. Only if a person receives a gene for blood group O from both parents will laboratory testing identify his or her blood group as O. If the person receives a gene for group O from one parent and group A from the other parent, group A will be expressed in laboratory blood typing.
Other alleles are equally dominant. The person who receives a gene for blood group A from one parent and group B from the other will have type AB blood because both alleles are equally dominant and both are expressed in blood typing.
Dominance and recessiveness are not absolute for all genes. Some people with a single copy of an abnormal recessive gene (carriers) may have a slightly abnormal level of the gene product (e.g., an enzyme) that can be detected by laboratory methods. These people usually do not have the disease because the normal copy of the gene directs production of enough of the required product to allow normal or near-normal function.
Chromosome Location
Genes located on autosomes are either autosomal dominant or autosomal recessive, depending on the number of identical copies of the gene needed to produce the trait. However, genes located on the X chromosome are paired only in females because males have one X and one Y chromosome.
A female with an abnormal recessive gene on one of her X chromosomes usually has a normal gene on the other X chromosome that compensates and maintains relatively normal function. However, the male is at a disadvantage if his only X chromosome has an abnormal gene. The male has no compensating normal gene because his other sex chromosome is a Y. The abnormal gene will be expressed in the male because it is unopposed by a normal gene.
Patterns of Single-Gene Inheritance
Three important patterns of single-gene inheritance are (1) autosomal dominant, (2) autosomal recessive, and (3) X-linked. Box 10-1 summarizes characteristics and transmission of each pattern. The inheritance patterns are graphically illustrated with a genogram, or pedigree, to represent a family’s history and the relationships among family members.
The nurse may need to interpret the genogram for the patient. For example, when taking a genetic family history, the nurse might say, “I’m going to use several symbols to depict your family tree and its members’ health histories. This diagram is often called a genogram or a pedigree.”
Single-gene traits have mathematically predictable and fixed rates of occurrence. For example, if a couple has a child with an autosomal recessive disorder, the risk that future children from the same couple will have the disorder is one in four (25%) at every conception. The risk for the disorder is the same at every conception, regardless of how many of the couple’s children are or are not affected.
Autosomal Dominant Traits
An autosomal dominant trait is produced by a dominant gene on a non-sex chromosome. The expression of abnormal autosomal dominant genes may result in multiple and seemingly unrelated effects in the person. The gene’s effects may vary substantially in severity, leading a family to think that a trait skips a generation. A careful physical examination may reveal subtle evidence of the trait in each generation. Some people may carry the dominant gene but may have no apparent expression of it in their physical makeup.
In some autosomal dominant disorders, such as Huntington disease, the person having the gene will always have the disease if he or she lives long enough. In other disorders, only a portion of those carrying the gene will ever exhibit the disease. Achondroplasia is the most common type of dwarfism and is present at birth (congenital) while degeneration of the brain in Huntington disease is not usually apparent until adulthood. See www.lpaonline.org and www.hdsa.org for support information for these disorders.
New mutations often account for the introduction of autosomal dominant traits into a family that has no prior history. Men who father children in their fifth decade or later are more likely to have offspring with a new autosomal dominant mutation.
The person who is affected with an autosomal dominant disorder is usually heterozygous for the gene, that is, the person has a normal gene on one chromosome and an abnormal gene on the other chromosome of the pair that overrides the influence of the normal gene. Occasionally a person receives two copies of the same abnormal autosomal dominant gene. Such an individual is usually much more severely affected than someone with only one copy.
Autosomal Recessive Traits
An autosomal recessive trait occurs when a person receives two copies of a recessive gene carried on an autosome. Most people carry a few abnormal autosomal recessive genes without problems because a compensating normal gene produces enough of the gene’s product for normal function. Because the probability that two unrelated people will share even one of the same abnormal genes is low, the incidence of autosomal recessive diseases is relatively low in the general population.
Situations that increase the likelihood that two parents will share the same abnormal autosomal recessive gene are:
• Consanguinity, or, blood relationship, of the parents
• Membership in groups that are isolated by culture, geography, religion, or other factors
Many autosomal recessive disorders are severe, and affected people may not live long enough to reproduce. Two exceptions are phenylketonuria and cystic fibrosis. Improved care of people with these disorders has allowed them to live into their reproductive years. If one member of the couple has the autosomal recessive disorder, all of their children will be carriers. Their risk for having similarly affected children is higher as well, depending on the prevalence of the abnormal gene in the general population.
X-Linked Traits
X-Linked Recessive Disorders
X-linked recessive traits are more common than X-linked dominant ones. Sex differences in the occurrence of X-linked recessive traits and the relationship of affected males to one another distinguish these disorders from autosomal dominant or recessive disorders. Males usually show full effects of an X-linked recessive disorder because their only X chromosome has the abnormal gene on it. Females can show the full disorder in two uncommon circumstances:
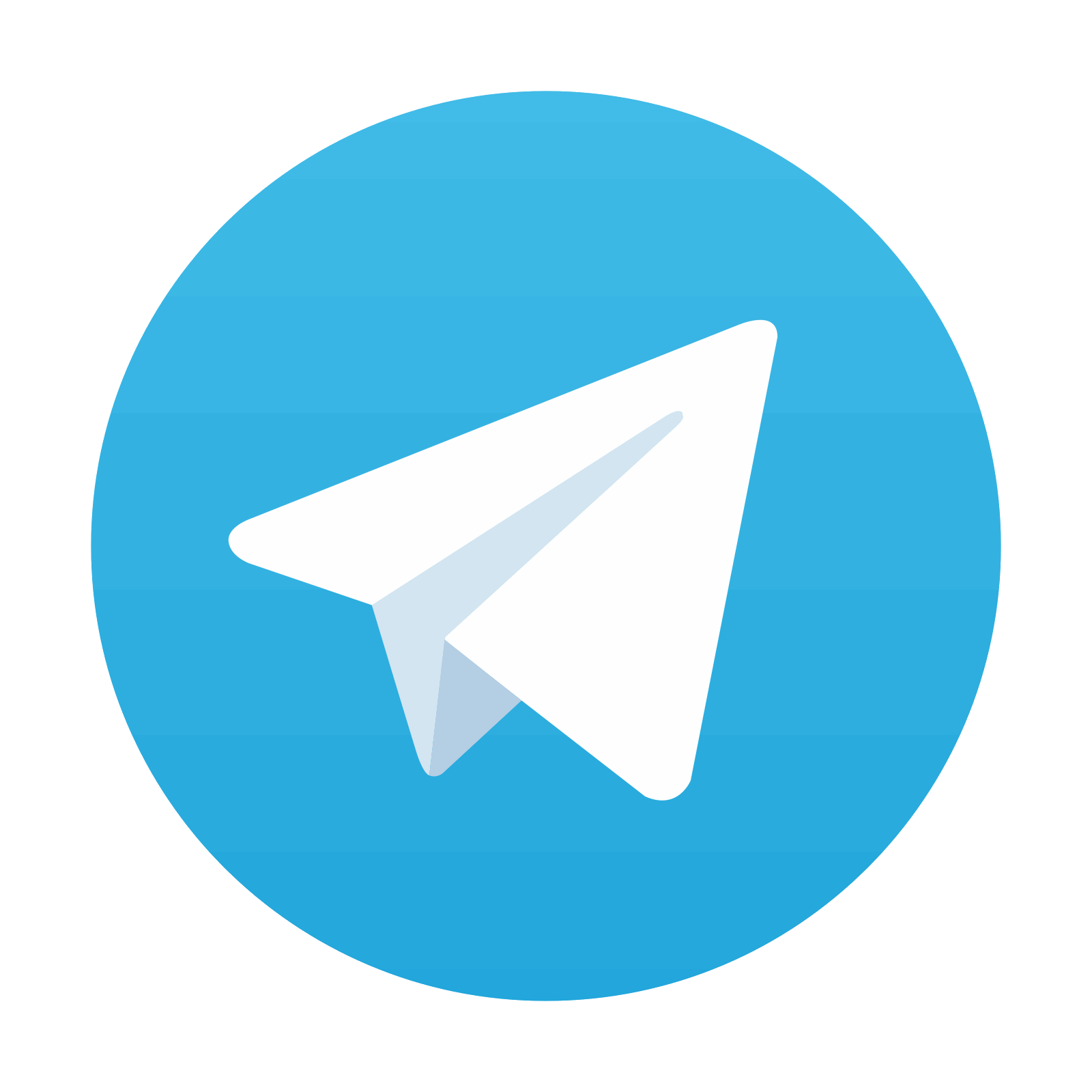
Stay updated, free articles. Join our Telegram channel

Full access? Get Clinical Tree
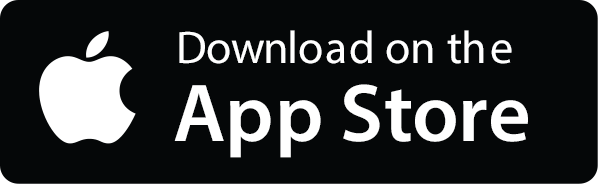
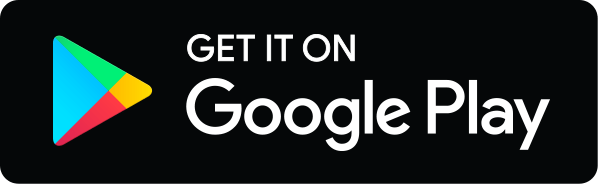