Chapter 6 Genetic Concepts for Medical-Surgical Nursing
Safe and Effective Care Environment
1. Coordinate with health care team members and genetics professionals when providing genetic testing information to patients and families.
2. Advocate for the patient with regard to whether or not to have genetic testing, informed consent before testing, and sharing of test results.
3. Ensure that confidentiality of genetic test results is maintained by all health care team members.
Health Promotion and Maintenance
4. Teach the patient and family who are at increased genetic risk for a disease or disorder to implement environmental modifications to reduce risk.
5. Assess patient and family responses to the findings of genetic testing.
6. Support the decision of the patient and family to have or not to have genetic counseling or testing.
7. Compare the concepts of phenotype and genotype.
8. Compare the patterns of inheritance for single gene traits.
9. Explain how genetic variations can affect adult health problems and their management.
10. Construct a three-generation pedigree from data obtained during the family history section of patient assessment.
11. Identify patients at increased genetic risk for health problems.
12. Explain how genetic testing is different from other laboratory tests.
13. Describe the role of the medical-surgical nurse in genetic counseling.
http://evolve.elsevier.com/Iggy/
Answer Key for NCLEX Examination Challenges and Decision\xE2\x80’Making Challenges
Review Questions for the NCLEX® Examination
Although not everything is known about human genomics, specific discoveries regarding each person’s genetic differences are being used to assess disease risk, enhance disease prevention strategies, and personalize disease management approaches. As a result, all health care professionals, including registered nurses, are expected to have at least a minimum knowledge of basic genetics to provide the best possible care for patients and families. Professional organizations, such as the American Nurses Association, the American Association of Colleges of Nursing, the American Academy of Nursing, and the National League for Nursing, as well as many specialty nursing organizations, support this position. These groups have agreed with the competencies established by the National Coalition for Health Professional Education in Genetics (NCHPEG) as the minimum essential genetic competencies required for safe nursing practice and have defined additional selected competencies. Table 6-1 lists selected genetic competencies considered particularly important in medical-surgical nursing.
TABLE 6-1 SELECTED ESSENTIAL GENETIC COMPETENCIES FOR MEDICAL-SURGICAL NURSING PRACTICE
Adapted from competencies identified by American Association of Colleges of Nursing. (2008). The essentials of baccalaureate education for nursing practice. Washington, DC: Author; American Nurses Association. (2008). Essentials of genetic and genomic nursing: Competencies, curricula guidelines, and outcome indicators (2nd ed.). Silver Springs, MD: Author; National Coalition for Health Professional Education in Genetics. (2007). Core competencies in genetics for health professionals (3rd. ed.). Retrieved October 2010, from www.nchpeg.org/core/core_comps_english2007.pdf.
Genetic Biology
Genes are composed of DNA, which is present as 46 separate large chunks within the nucleus (Fig. 6-1). During cell division, each large chunk of DNA replicates and then organizes into a chromosome form to ensure precise delivery of the genetic information to each of the two new daughter cells. Thus DNA, chromosomes, and genes are all the same basic thing; only the structures differ.
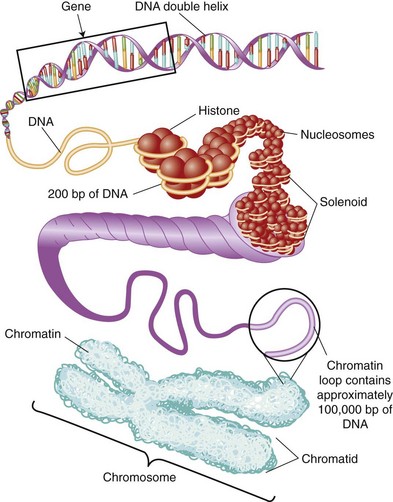
FIG. 6-1 The various forms of DNA from loose to coiling DNA tightly into a chromosome. bp, Base pair.
DNA
DNA Structure
Fig. 6-2 shows a very small piece of double-stranded DNA on the left (containing only four base pairs) taken from the larger piece of DNA on the right. The phosphate groups that hold the nucleotides together as a strand are in the red box. The green box in the lower left-hand section shows a whole nucleotide (a base with the sugar and the phosphate group) in place in the left-hand DNA strand. The blue box in the middle of the two strands shows how the base from the left strand lines up with and pairs to a complementary base in the right strand.
The four bases in DNA are adenine (A), guanine (G), cytosine (C), and thymine (T). Each base becomes a complete nucleotide when a five-sided sugar (known as a deoxyribose sugar) and a phosphate group are attached (Fig. 6-3). Nucleotides form the DNA strands with the phosphate groups holding the bases in place.
Base pairs are the linked bases in the two opposite strands of DNA. The bases always link together across from each other in a very specific way. Thymine always forms a pair with adenine, and cytosine always forms a pair with guanine. Thus the bases of each pair are complementary to each other. Because these complementary base pairs in DNA are specific, if the base sequence of one strand of DNA is known, the opposite strand’s sequence could be accurately predicted. For example, if the left-hand section of DNA (strand 1 in Fig. 6-4) had the sequence A-G-G-C-T-C-A-A-C-C-T-G, the corresponding (complementary) right-hand section (strand 2 in Fig. 6-4) of DNA would have the sequence T-C-C-G-A-G-T-T-G-G-A-C.
When the two strands of DNA are lined up properly, they twist into a loose helical shape (see Fig. 6-1). In this shape, the DNA is so fine that it can be seen only with electron microscopes. Only when a cell begins to divide (i.e., undergoes mitosis) does the DNA super-coil tightly into dense pieces called chromosomes (see Fig. 6-1), which can be seen with standard microscopes.
DNA Replication
DNA must reproduce itself (replicate) every time a cell divides. Cell division (mitosis) occurs in a regulated pattern known as the cell cycle. The purpose of mitosis is for one cell to reproduce into two new cells, each of which is identical to the cell that started mitosis. For each new cell to have exactly the right amount of DNA and genes, the DNA in the dividing cell must exactly replicate. This process involves having the double strands of DNA separate and then enzymes read the sequence of the original strands and build two new strands that are perfectly complementary to the original strands (Fig. 6-5).
Chromosomes
As shown in Fig. 6-1, a chromosome is a specific large chunk of highly condensed double-stranded DNA, with each chunk containing billions of bases and hundreds (and sometimes thousands) of genes. Each chromosome forms and moves to the center of the cell that is about to divide. Just before the cell splits into two cells, each chromosome is pulled apart so that half of each chromosome goes into one new cell and the other half goes into the other new cell. Thus chromosomes are temporary structures, but their job is important: precise delivery of DNA to the two new cells. Humans have 46 chromosomes divided into 23 pairs. This number is the “diploid” number of chromosomes for humans.
Fig. 6-6 shows a metaphase chromosome. The “pinched in” area of the chromosome is the centromere. Each left and right half of the chromosome is a chromatid. The “arms” above the centromere are the short arms, or the “p” arms. The longer arms (not legs) below the centromere are the “q” arms. A particular gene may be listed as located on 9q, meaning that the gene has its location (locus) on the long arm of the number 9 chromosome.
A karyotype is an organized arrangement of all of the chromosomes present in a cell during the metaphase section of mitosis (Fig. 6-7). A picture of the chromosomes is made. Chromosomes are first paired up and then arranged according to size (largest first) and centromere position. This gross organization of DNA can be used to determine missing or extra whole chromosomes and some large structural rearrangements. A missing gene or a mutated gene would not show up at this level of analysis. What can be learned about the person from whom the karyotype in Fig. 6-7 was made is that the person is human, female, and euploid (has the correct number of chromosome pairs for the species). This person is chromosomally “normal,” although she might have one or more genes that are mutated. If the karyotype is abnormal in any way (had more or less than the normal number or had broken chromosomes), the karyotype would be called aneuploid.
Autosomes are the 22 pairs of human chromosomes (numbered 1 through 22) that do not code for the sexual differentiation of a person. Autosomal chromosomes contain genes that code for all the structures and regulatory proteins needed for normal function. Sex chromosomes are the pair of chromosomes that include the genes for the sexual differentiation of the person. Chromosomally normal males have an X and a Y as the sex chromosomes. Chromosomally normal females have two XXs as the sex chromosomes (see Fig. 6-7).
Gene Structure and Function
A gene is a specific segment(s) of DNA that contains the code (recipe) for a specific protein (see Fig. 6-1). One gene usually codes for one protein; thus genes are the smallest functional unit of the DNA. Each chromosome contains hundreds of genes (and each chromosome is made up of a large segment of DNA). Thus an individual gene is a very small segment of DNA.
If a person has inherited a blood type A allele from his or her mother and a blood type B allele from his or her father, he or she has the A and B alleles; the blood type expressed when the blood bank determines type is type AB. Fig. 6-8 shows this concept. In Fig. 6-8, two people are about to become pregnant. What are the possibilities for this baby to have a specific type of ear shape (pointy, rounded, square, triangular)? The gene for ear shape is trait 1, and it (for the purposes of this explanation) is on chromosome number 6.
Each of the father’s sperm contains only one number 6 chromosome, and each of the mother’s eggs contains only one number 6 chromosome (so that when the sperm fertilizes the egg, the resulting person conceived will have only one pair of chromosome number 6 instead of two pairs of chromosome number 6). In Fig. 6-8, the paternal chromosomes are represented in blue and the maternal chromosomes are represented in pink. Throughout this text, when single gene health problems are described using either a chromosome depiction or a Punnett square depiction, paternal genetic input is represented in blue and maternal genetic input is represented in pink.
Half the father’s sperm have the 1a allele for ear shape, and the other half have allele 1b for ear shape. Half the mother’s eggs have 1c for ear shape, and the other half have 1d. The resulting baby can inherit only a 1a or a 1b from the father, not both; and this same baby can inherit only a 1c or a 1d from the mother—again, not both. The lower portion of Fig. 6-8 shows all the combinations possible for each ear shape gene alleles for any child these two people have.
< div class='tao-gold-member'>
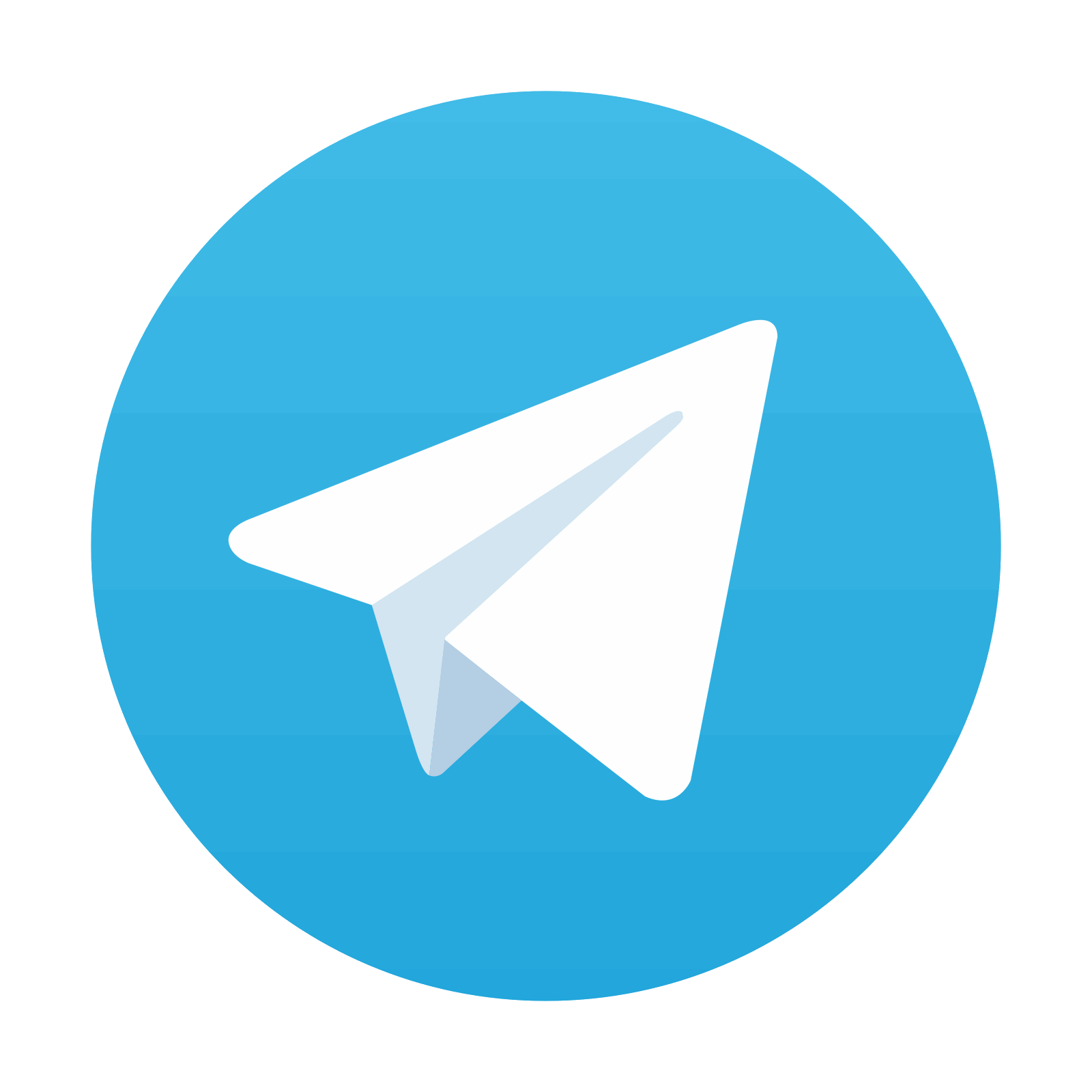
Stay updated, free articles. Join our Telegram channel

Full access? Get Clinical Tree
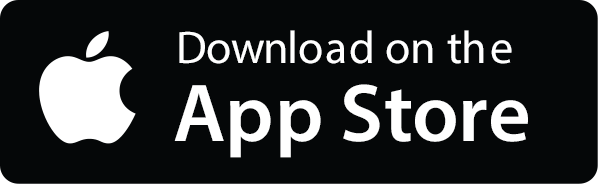
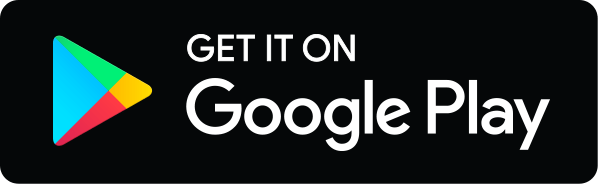