The Brain
There are four principal parts to the brain (Table 7.3):
- Brain stem (medulla oblongata, pons, midbrain).
- Cerebrum.
- Cerebellum.
- Diencephalon (hypothalamus, thalamus, pineal gland).
Table 7.3 Principal parts of the brain and their functions
Source: Levy et al. 2006; Tortora and Derrickson 2009.
Structural components | Anatomy and summary of the functions |
Brain stem | The medulla is continuous with the spinal cord, beginning at the foramen magnum and extending to the inferior border of the pons. The white matter of medulla contains all the sensory (ascending) tracts and all the motor (descending) tracts which extend between the spinal cord and the rest of the brain. Some of the white matter forms bulges on the anterior aspect of the medulla, which are known as pyramids. These contain the corticospinal tracts that run from the cerebrum to the spinal cord. Just above junction of medulla and spinal cord, decussation of the pyramids occurs. This is where 90% of axons in the right pyramid and 90% of axons in the left pyramid cross to their respective opposite pathways. This explains why the right side of the brain controls the left side of the body, and vice versa. |
Medulla | The medulla also contains several nuclei which are masses of grey matter where neurons form synapses with each other. A number of these nuclei control essential functions including:
Additionally the medulla contains nuclei associated with five pairs of cranial nerves:
|
Pons | The pons (bridge) lies directly above the medulla and anterior to the cerebellum. It consists of both nuclei and tracts and the primary function is to act as a bridge connecting various parts of the brain. Signals for voluntary movements originate in the cerebral cortex and are relayed through pontine nuclei into the cerebellum. Pneumotaxic area – (pontine respiratory group PRG) The PRG antagonises the apneustic centre, cyclically inhibiting inspiration. The PRG limits the burst of action potentials in the phrenic nerve, effectively decreasing the tidal volume and regulating the respiratory rate. Absence of the PRG results in an increase in depth of respiration and a decrease in respiratory rate. Apneustic area The apneustic centre of the lower pons appears to promote inspiration by the stimulation of the neurons in the medulla oblongata providing a constant stimulus. |
Midbrain | The midbrain extends from the pons to the diencephalon and contains both tracts and nuclei. The cerebral aqueduct passes through the midbrain connecting the third ventricle above it with the fourth ventricle below.
The midbrain contains the nuclei associated with two pairs of cranial nerves
|
Reticular formation | The reticular formation (RF) is a broad area which extends from the upper part of the spinal cord throughout the brain stem into the lower part of the diencephalon. Clusters of neuronal cell bodies (grey matter) interspersed among small bundles of myelinated axons (white matter) are found throughout the brain stem and form the RF. These neurones have both sensory and motor functions. Reticular Activating System (RAS) Composed of sensory axons which project to the cerebral cortex, the RAS helps maintain consciousness and is active when we are awakened from sleep. The RAS is also responsible for helping regulate muscle tone (degree of contraction in normal resting muscles) |
Cerebrum | The cerebrum is known as the ‘seat of intelligence’. The right and left halves of the cerebrum are known as cerebral hemispheres and are separated by the falx cerebri. The hemispheres are connected internally by the corpus callosum which is a broad band of white matter (containing axons). Each hemisphere consists of:
Each hemisphere can be further subdivided into four lobes: frontal, parietal, temporal and occipital.
|
Basal ganglia | Within each cerebral hemisphere are three nuclei collectively termed as the basal ganglia. Basal ganglia receive input from the cerebral cortex and provide output to the motor parts of the cortex via the medial and ventral group nuclei of the thalamus. In addition, the basal ganglia have extensive pathways which connect to each other. The major function of the basal ganglia to is to help regulate the initiation and termination of movements as well as controlling subconscious skeletal muscle contraction. |
Cerebellum | The cerebellum occupies the inferior and posterior portions of the cranial vault. It resembles a butterfly in shape with a central constricted area known as the vermis and lateral lobes which are the cerebellar hemispheres. Each hemisphere consists of lobes which are separated by deep and distinct fissures. The superficial layer of the cerebellum is the cerebellar cortex consisting of grey matter. Lying under the grey matter is the white matter, which lies in tracts known as arbor vitae. Buried within the white matter lie the cerebellar nuclei, which give rise to axons carrying impulses from the cerebellum to other brain structures and the spinal cord. Three paired cerebellar peduncles attach the cerebellum to the brain stem. Each of the peduncles carry different sets of information, e.g.
Feedback signals are relayed to the motor areas of the cerebral cortex via the thalamus/red nuclei to correct abnormal movements. The cerebellum is also the main area for the regulation of balance and posture. Abnormalities within the cerebellum will lead to disorders of speech patterns and ataxias. |
Diencephalon | The hypothalamus is responsible for the maintenance of the body’s homeostatic balance. It achieves this through undertaking a number of roles:
|
Table 7.4 Hormones secreted by the hypothalamus and the area of the pituitary gland affected
Anterior pituitary | Posterior pituitary |
Thyrotrophin releasing hormone (TRH) Gonadotrophin releasing hormone (GnRH) Growth hormone releasing hormone (GHRH) Corticotrophin releasing hormone (CRH) Somatostatin Dopamine These are released into the blood and travel to the capillary bed in the anterior pituitary gland where they trigger the release of other hormones. | Vasopressin (ADH) Oxytocin These travel via the neurons to the posterior pituitary where they are released directly into the blood stream. |
The Blood–Brain Barrier
The blood–brain barrier exists to protect the brain cells from harmful substances and pathogens. The barrier is not a physical barrier as such, but a combination of the presence of tight junctions in the endothelial cells of the capillaries and the presence of a basement membrane around the capillaries.
The blood–brain barrier is selectively permeable to certain substances, including:
- Water.
- Oxygen and carbon dioxide.
- Glucose.
- Alcohol.
- Anaesthetic agents.
The blood–brain barrier is essentially impermeable to proteins and most antibiotics. Trauma, certain toxins and inflammation can cause a breakdown of the blood–brain barrier.
Neurological Assessment
Neurological assessment in the critically ill child is a challenging part of critical care nursing as many factors may affect the child’s neurological function, including the child’s underlying cognitive maturation, the effects of sedative or analgesic agents, the consequences of systemic critical illness, as well as the psychological effects of intensive care. Neurological assessment is important because it is the only guide to neurological function in children who are unable to communicate due to age or cognitive ability, and prevention of secondary insult is vital in improving morbidity (and mortality) in children with primary brain injury.
The immaturity of the infant’s neurological system is very evident, as is their subsequent growth and development. The major portion of brain growth occurs in the first year of life, along with completion of the myelination of brain and nervous system. Motor function maturation proceeds in a cephalocaudal direction and is a succession of integrated milestones. Brain growth continues up to 15 years of age. There are a number of primitive reflexes present in the infant which disappear over the first few months of life. The absence of these reflexes in the newborn, or their presence after the first year of life, is a cause of concern and merits further investigation.
- Palmar grasp (birth): disappears by 3 months.
- Plantar grasp (birth): disappears by 9 months.
- Moro reflex (birth): disappears by 6 months.
- Placing (4 days): disappearance variable.
- Stepping (0–8 weeks): disappears before walking.
- Fencing (2–3 months): disappears by 6 months.
Consideration for Practice
The plantar reflex (also known as the Babinski response), the presence of which is an abnormal finding in the older child or adolescent, is an unreliable reflex test in younger children who have yet to start walking and will be present in infants under the age of 1 year as a normal finding. A positive Babinski response means that when the sole of the foot is stroked in an upward motion, the toes will fan out rather than the foot flexing at the ankle and the toes curling inwards as would be expected.
The bones of the cranial vault are incompletely fused at birth, with the marginal gaps between them connected by membranous tissues. This facilitates a reduction in the skull size during the passage down the birth canal. The gaps between the bones are known as fontanelles and through a gradual process of ossification of the membranes, the gaps close in the first two years of life. The anterior fontanelle lies in the midline between the frontal and parietal bones and will close between 18 months and 2 years of age. The posterior fontanelle, which lies between the parietal and occipital bones, usually closes within the first two months of life.
A rapid assessment tool, advocated for use primarily for the first assessment of a critically unwell infant or child (e.g. in the Emergency Department or in an emergency situation) is the AVPU scale. This very simple tool assesses the child’s responsiveness, which is good indicator of general cerebral perfusion, however it does not provide the same depth of information regarding the function within the CNS so the infant or child with primary neurological dysfunction (traumatic or non-traumatic) should also be assessed using the appropriate GCSS (National Institute for Health and Clinical Excellence [NICE] 2007).
The most frequently used tool for neurological assessment used in critical care is the Glasgow Coma Scale Score (GCSS or GCS) which was originally developed in 1974 by Teasdale and Jennett for use in the adult population. The GCSS is divided into three sections– eye-opening response, verbal response and motor response – with a final score ranging from 3 to 15.The total score achieved is reflective of CNS functioning, with patients achieving low scores having decreased functioning. There have been a number of adaptations of the tool since its introduction and for the under 4 year olds, the modified Glasgow Coma Scale Score, adapted to take into account pre-verbal children, is now used. In addition, the James adaptation (JGCS), which includes a grimace score rather than a verbal score for intubated children (Tatman et al. 1997), ensures that this group of children can also be assessed fully.
One of the keys to effective assessment is validation of an individual practitioner’s findings with the other healthcare professionals involved in the child’s care to avoid irregularities in scoring based on subjective interpretation of the criteria applied within each section. Many centres have produced their own version of the GCSS/modified GCSS incorporated into printed observation charts such as that detailed in Figure 7.1, which allows for continuity of recorded observations in the child whose clinical condition changes (e.g. the child who either requires intubation or is successfully extubated).
Figure 7.1 Neurological observation chart for the under fours incorporating the grimace score.
Reproduced with permission from Bristol Royal Hospital for Children.
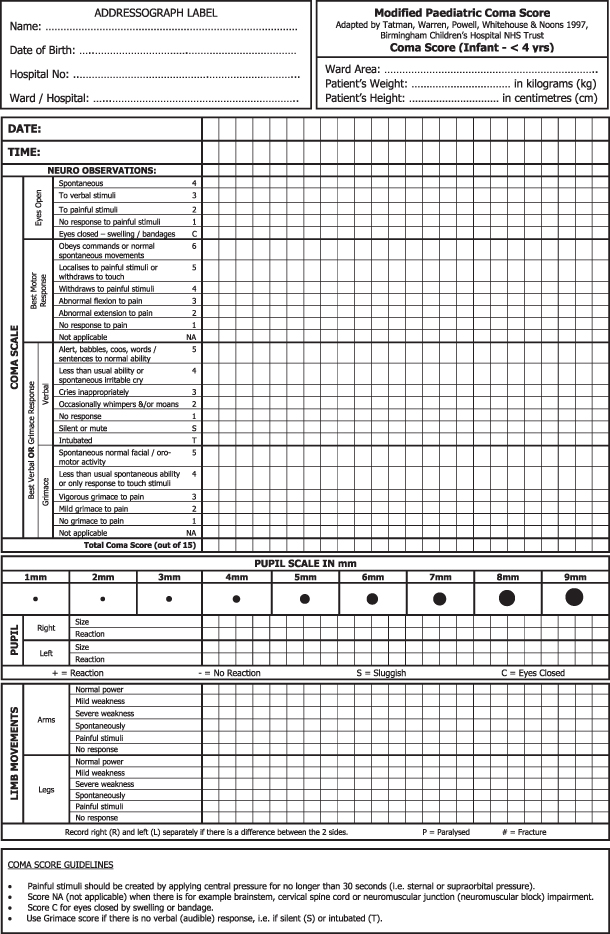
Pupillary assessment of size, shape and reactivity is an important component of a neurological assessment.
Considerations for Practice
- Pupil size can be influenced by poisons such as opiates (pinpoint) or tricyclic anti-depressants (dilated).
- Unequal pupils can indicate a unilateral lesion.
- Unilateral dilated pupil may be indicative of 3rd nerve compression on the same side or a subdural haematoma.
- Reactivity – sluggish pupil reactions may occur due to sedation.
- Administration of adrenaline and/or atropine during resuscitation may lead to abnormal pupillary reactions (e.g. dilated, seemingly unreactive pupils in the immediate post-event period).
- The use of dilating drops for ophthalmological examination, in cases of suspected non-accidental injury, may cause abnormal findings (e.g. dilated unreactive pupils). Cyclopentolate drops, which are a parasympathetic antagonist, act by paralysing the iris sphincter muscle. This will both make the pupil larger and paralyse the muscle involved in focusing of the lens. The effects of cyclopentolate may last for up to 24 hours.
- Fixed and dilated pupils in the absence of the above factors indicate brain stem death/herniation.
- Congenital or acquired conditions may produce effects that influence pupil reactions, for example:
- Coloboma: this is an uncommon, congenital condition characterised by a unilateral or bilateral partial iris defect.
- Horner’s syndrome: results from an interruption of the sympathetic nerve supply to the eye characterised by the classic triad of miosis (constricted pupil), the presence of a partial ptosis and loss of hemi-facial sweating (anhidrosis).
- Coloboma: this is an uncommon, congenital condition characterised by a unilateral or bilateral partial iris defect.
Other clinical parameters which should be incorporated as part of neurological assessment include:
- Heart rate, blood pressure, respiratory rate and pattern.
- Assessment for the presence of photophobia.
- Assessment for the presence of neck stiffness (meningism).
- Assessment of fontanelles – bulging anterior fontanelles may be indicative of volume overload or meningitis.
- Glucose – hypoglycaemia will lead to decreased levels of consciousness and possible coma.
- PaO2 and PaCO2 levels – hypoxia and hypercapnia both affect the child’s level of consciousness.
- Core temperature – hypothermia will lead to altered level of consciousness and in neonates may lead to irregularities in respiratory pattern, periods of apnoea and bradycardia.
Causes of decreased conscious level in children are multifactorial, however coma in children is caused by diffuse metabolic insult in 95% of cases and by structural lesions in the remaining 5% (Advanced Paediatric Life Support 2011). Causes include:
- Head injury.
- Space-occupying lesions.
- Seizures.
- Infection.
- Metabolic causes, such as diabetic ketoacidosis, hypernatraemia, fulminant hepatic failure.
- Vascular lesions.
- Hypoxic, ischaemia, encephalopathy (HIE), post-cardiorespiratory failure.
- Administration of anaesthesia and/or sedative agents.
- Accidental or deliberate ingestion of toxins (e.g. alcohol and/or drugs).
Central Nervous System Infections
Bacterial Meningitis
This is less common than viral meningitis but bacterial meningitis has a reported overall mortality of 10–30% worldwide (Levine et al. 2010). Up to 10% of children affected will suffer permanent neurological impairment such as hearing loss, memory loss or altered mood patterns.
The causative pathogens vary with age although there are some common causes across the age range seen in children’s care (Table 7.5).
Table 7.5 Age-related bacterial causes of meningitis
Age group | Common causative pathogens |
Newborn (up to 3 months) | Group B streptococcus E. coli Listeria Haemophilus influenzae type B (uncommon) |
Infants/young children | Neisseria meningitidis (meningococcal infection) Streptococcus pneumoniae (pneumococcal infection) Haemophilus influenzae type B |
School-age children | Neisseria meningitidis (meningococcal infection) Streptococcus pneumoniae (pneumococcal infection) |
Pathophysiology of Bacterial Meningitis
Bacteria will either reach the subarachnoid space transported in the blood or may directly reach the meninges in patients with a parameningeal focus of infection, such as abscesses in the epidural or subdural spaces. Once the bacteria are established in the subarachnoid space, a host inflammatory response is triggered by bacterial cell wall products produced as a result of bacterial lysis. This response is mediated by the stimulation of specific cells within the brain parenchyma which respond as macrophages would and produce cytokines and other inflammatory mediators. This cytokine activation then initiates several processes that ultimately cause damage in the subarachnoid space, culminating in neuronal injury and cell death through apoptosis. As a consequence of the infective processes and the responses invoked there is swelling and proliferation of the endothelial cells in the local arterioles and the veins causing obstruction of flow through these vessels. This results in an increase in intracellular sodium and intracellular water. The development of cerebral oedema further compromises cerebral circulation, which can result in increased intracranial pressure. Increased secretion of antidiuretic hormone resulting in the syndrome of inappropriate antidiuretic hormone secretion (SIADH) occurs in most patients with meningitis, which causes further retention of free water. These factors contribute to the development of either focal or generalised seizures.
Pathophysiology of Neonatal Meningitis
Bacteria from the maternal genital tract can colonise the foetus after the rupture of membranes and specific bacteria, such as Group B streptococci (GBS), enteric gram-negative rods, and Listeria monocytogenes can reach the foetus via placental transfer and cause infection. Newborns can also acquire bacterial pathogens from their surroundings, and several host factors facilitate a predisposition to bacterial sepsis and meningitis. Bacteria reach the meninges via the bloodstream and cause inflammation. After reaching the CNS, bacteria spread from the longitudinal and lateral sinuses to the meninges, the choroid plexus and the ventricles. Inflammation of the meninges and ventricles produces a polymorphonuclear response alongside an increase in cerebrospinal fluid (CSF) protein content and an increased utilisation of glucose in CSF. Inflammatory changes and tissue destruction in the form of empyema and abscesses are more pronounced in gram-negative meningitis. Thick inflammatory exudate causes blockage of the aqueduct of Sylvius and other CSF pathways, resulting in both obstructive and communicating hydrocephalus.
Clinical Presentation
The clinical signs of bacterial meningitis develop over a relatively short period, normally within a few hours. Early signs are very similar to those found in viral meningitis, although the relative rapidity of their development may give a clue to a bacterial cause.
Clinical signs may be divided into non-specific and specific signs (Table 7.6).
Table 7.6 Clinical signs of bacterial meningitis
Non-specific signs | Specific signs |
Lethargy and/or drowsiness Irritability and poor handling in the neonate Poor feeding Nausea and/or vomiting Fever Headache | Bulging fontanelles in infants Light sensitivity/photophobia Neck stiffness (may not be evident in infants) Decreased level of consciousness Seizures or other focal neurological signs Positive Brudzinski’s or Kernig’s signs. These are physical signs of meningeal irritation which can be elicited when the spinal cord is stretched or flexed. They may be absent in the younger child.
|
Considerations for Practice
The presence of a non-blanching petechial rash is indicative of septicaemia and must be acted on immediately. Children may also present with coagulopathies, which again required prompt intervention.
Management of the Child with Bacterial Meningitis
Priorities of care for this group of children are geared towards treating the symptoms and, where possible, preventing complications, such as cerebral oedema or cerebral abscesses.
- The main treatment priority is the administration of appropriate IV antibiotics according to the sensitivity of the organism involved. Once samples have been obtained broad spectrum therapy can be given until results are available.
- The use of steroids is advocated in the management of bacterial meningitis, with the drug of choice usually being dexamethasone. However, its use is not recommended in infants under 3 months of age or if more than 12 hours has passed since the administration of the first dose of antibiotics (NICE 2010).
- Lumbar puncture is contraindicated in children who are demonstrating signs of possible raised intracranial pressure as the sudden release of pressure could cause herniation of the brainstem. It is also contraindicated in the presence of a coagulopathy or cardiovascular instability. A CT scan may be required to determine the presence of cerebral oedema in these children.
- Fluid and electrolyte management – fluid restriction reduces the risk of the child developing cerebral oedema. Maintaining electrolytes within normal limits can prevent fluid shifts and reduce the risk of the child developing cerebral oedema. Increased cellular activity increases the metabolic demands for glucose and other nutrients, and as hypoglycaemia may cause seizures in children, it is essential that the serum blood glucose levels are maintained within normal limits.
Complications of Bacterial Meningitis
- Hearing loss.
- Subdural effusions (particularly associated with pneumococcal meningitis).
- Cerebral abscesses, which will require surgical drainage.
- Seizures.
- Hydrocephalus.
Viral Meningitis
This is the most common cause of meningitis; most cases are relatively mild and seldom fatal. Viral meningitis does not usually result in septicaemia and the risk of complications is minimal in contrast with the bacterial form of the disease. It is extremely rare for a child with viral meningitis to warrant intensive care admission; indeed, few children will merit hospital admission at all.
Common causes include adenoviruses, herpes viruses, Epstein–Barr virus and, more rarely, the mumps virus. Clinical signs usually develop over a period of days, although they may have an acute onset (uncommon) but are rather non-specific and mimic those seen in a flu-type illness.
Viral Encephalitis
Encephalitis is a much rarer form of neurological illness than meningitis and refers to a more generalised inflammation of the brain tissue as well as possible involvement of the meninges. Encephalitis is normally viral in origin although bacterial and fungal-associated encephalitis can develop. The exact infective mechanism is virus-specific, with some being transmitted by human contact while others may occur as a consequence of insect or tick bites. Viral encephalitis can be primary (where virus is present in the CNS) or post-infection, due to immunoallergic mechanisms. More than 150 viruses have been implicated in the pathogenesis of encephalitis; however, because of limitations with diagnostic testing, causative factors of more than 50% of cases remain unknown (Yao et al. 2009).
Frequently identified viral strains responsible for encephalitis include:
- Herpes simplex virus (HSV) types 1 and 2.
- Epstein–Barr virus (EBV).
- Cytomegalovirus (CMV) (more common in immune-compromised children).
- Varicella zoster virus (VZV) (more common in immune-compromised children).
- Paramyxovirus family:
- Measles.
- Mumps.
- Measles.
- Togavirus family:
- Rubella.
- Human herpes virus 6.
- Arbovirus (transmitted by tick bite).
- Rabies (transmitted by animal bite).
- Rubella.
Pathophysiology of Viral Encephalitis
The virus usually replicates outside the CNS and gains entry through the blood or by travelling along neural and olfactory pathways. Once the virus has crossed the blood–brain barrier it enters the neural cells, causing significant disruption in their functioning. As a consequence of viral infection, perivascular congestion, haemorrhage and significant inflammatory responses occur which affect the grey matter disproportionately to the white matter. Localised pathology occurs as a result of neurone cell membrane receptors found only in specific portions of the brain. An example is the herpes simplex virus (HSV), which targets the inferior and medial temporal lobes in particular.
Clinical Presentation
- Fever.
- Uncharacteristic behaviour.
- Confusion and/or altered level of consciousness.
- Seizures.
- More focal neurological signs may be noted at initial presentation or they may become apparent as the illness progresses. These signs will be determined by the specific area(s) of the brain affected and will therefore vary from child to child.
Management of the Child with Encephalitis
Management of encephalitis is essentially supportive and follows the major principles detailed in the management of bacterial meningitis.
As the cause is normally viral in origin, the use of antibiotics is limited but aciclovir therapy should be continued until there is either confirmation of the viral organism involved or there has been exclusion of the HSV as the cause.
HSV is one of the least common causes of encephalitis but it can have devastating consequences if not managed correctly, with a mortality rate of >70% if untreated and ongoing neurological impairment in many of the children who survive. It may occur either as a primary infection or due to reactivation of latent infection, especially in the neonatal population. Clinical presentation is normally associated with a history of fever and malaise, in addition to which there may have been disturbances of memory or behaviour. The illness progresses with reduced levels of consciousness and seizures which are often focal and ongoing. HSV causes necrotic lesions, typically in the temporal lobes, but these can be widespread and even involve the brainstem. There is raised ICP and papilloedema in approximately 15% of cases and these children will require intensive care management (see Raised intracranial pressure).
Approximately 40% of children who survive will have long-term adverse consequences (Tunkel et al. 2008):
- Minor to major learning disabilities.
- Memory impairment.
- Neuropsychiatric abnormalities.
- Epilepsy.
- Fine motor control deficits.
- Dysarthria.
Acute Disseminating Encephalomyelitis (ADEM)
ADEM is an uncommon presentation of an inflammatory demyelinating disease which affects only a small number of children each year in the United Kingdom. The onset of ADEM usually occurs in the wake of either a clearly identifiable febrile prodromal illness or an episode of immunisation (although there remains considerable discussion in the literature about the strength of the evidence which supports this) and in association with encephalopathy of varying degrees, which is one of the differential diagnostic criteria distinguishing ADEM from multiple sclerosis (Dale and Branson 2005).
Clinical Presentation
- Abrupt onset of lethargy and irritability.
- Altered level of consciousness.
- Behavioural changes.
- Fever.
- Headache.
- Focal or generalised signs of neurological dysfunction:
- Focal or generalised seizures.
- Ataxia.
- Cranial nerve palsies.
- Hemiparesis.
- Focal or generalised seizures.
Management of the Child with ADEM
Management is focused on reducing the inflammatory processes through the use of steroids and, in cases of refractory or relapsing disease, plasma exchange and the provision of IV immunoglobulin therapy. Intensive care support is matched to the individual child’s needs, some of whom may require intubation and ventilatory support due to inadequate airway protective mechanisms or rapid deterioration in their clinical condition (Barry et al. 2010).
The prognosis is good for the majority of children, with full recovery occurring within weeks. Current acute mortality rates in the published literature are thought to be around 2% or less and mortality is most usually associated with children who have either fulminant cervical transverse myelitis or cerebral oedema. There is an increased risk of the child developing MS as a consequence of experiencing ADEM, with long-term figures being estimated at anywhere between 6 and 25% (Rust 2010; Wingerchuk 2006).
Guillain-Barré Syndrome (GBS)
Classic GBS is a demyelinating neuropathy associated with ascending weakness, however there are a number of well-documented clinical variants. Among these are acute inflammatory demyelinating polyradiculoneuropathy, which is the most widely recognised form in Western countries, with variants known as acute motor axonal neuropathy (AMAN) and acute motor-sensory axonal neuropathy (AMSAN) also being well recognised (Andary 2011). The most common presentation is characterised by an acute monophasic, non-febrile, post-infectious illness manifesting as ascending weakness and areflexia. Sensory, autonomic and brainstem abnormalities may also be seen during the child’s clinical course (Chibber 2011).
Clinical presentation
- Abnormal gait.
- Progressive, symmetrical muscle weakness.
- Pain.
- Dysaesthesia (abnormal sensations such as a burning sensation).
- Autonomic instability resulting in orthostatic hypotension (secondary to standing upright), hypertension, pupillary dysfunction, sweating abnormalities and sinus tachycardia.
- Respiratory weakness leading to respiratory failure (in severe cases).
- Bulbar muscle weakness (in severe cases).
There are a number of stages in the disease process from the onset (1–2 weeks before becoming acutely unwell) through to acute presentation (for up to 2 weeks, with progression of symptoms during this phase), then a plateau phase before reaching recovery or the resolution phase. Just as symptoms develop in an ascending order, recovery occurs in descending order; therefore the last symptoms to develop should be the first to show signs of improvement.
Management of the Child with GBS
As with the management of ADEM, the management of GBS is aimed at controlling symptoms and providing intensive care interventions such as ventilatory support (if required). Very few children require intensive care support; however, they may well need to be admitted for close observation if they have significant cardiovascular changes associated with autonomic instability.
Treatment strategies include:
- Regular assessment of respiratory function (4–6 hourly).
- Intubation and mechanical ventilation if respiratory failure present.
- Provision of IV immunoglobulin (IVIG) therapy over the first 3–5 days after acute presentation.*
- Use of plasma exchange for refractory disease.*
- Management of pain and or dysaesthesia with the use of agents such as gabapentin.
- Early physiotherapy to minimise the effects of immobility.
*There is an evidence base for the use of both IVIG and plasma exchange in the management of GBS with reported reductions in recovery time of as much as 50% (Miller 2011). There is no significant difference in terms of recovery demonstrated to date between the use of IVIG and plasma exchange in managing children or adults with GBS therefore most centres will use IVIG as a first-line intervention, given its ease of administration in comparison to plasma exchange. Similarly, there is no evidence to suggest that this combination of therapies administered together improves recovery rates (Hughes et al. 2010).
Seizure Disorders
Seizure disorders in children are an extremely complex group of clinical presentations, the majority of which will not be addressed in this text. Rather, this section focuses on the care of the child presenting in the intensive care unit in status epilepticus (SE).
Basic Pathophysiology of Seizures
The brain has over 10 million neurones the function of which is to transmit information from cell to cell. This is achieved by the presence of chemical messengers which make the cells more or less excitable. During episodes of super-excitability, many cells will fire together and the brain activity at a cellular level becomes out of control. A single burst of excessive activity is known as an epileptic spike discharge and groups of these discharges, which are uninhibited or uncontrolled, will lead to epileptic fits or seizures.
Classification of Seizures
There is a myriad of seizure types, but they can usually be classified as:
- Generalised – these arise over a wide area of the brain and affect both sides of the body from the beginning.
- Partial/focal – these arise from a specific focus in the brain and may be either simple or complex. These seizures may also develop into generalised seizures if they persist.
Acute Causes of Seizures
There is no identifiable cause in 50% of seizures. However, clinical conditions predisposing the infant or child to seizures include:
- Febrile convulsions.
- Toxic ingestion – poisons or alcohol.
- Metabolic disorders.
- Pre-existing seizure disorder – sub-therapeutic serum levels of anticonvulsants or concomitant illness.
- Trauma – head injury or birth trauma.
- Cerebral pathology – tumours, bleeds or abscesses.
- Systemic disturbances – sepsis, hepatic or renal failure.
Neonatal Seizures
Most healthy babies display startle-like movements or fine tremors when stimulated or during active sleep, however seizure activity is usually unstimulated. Due to the immaturity of the neonate’s brain, clinical signs of seizure activity may well be subtle and can therefore be missed. This is particularly applicable in the pre-term population (Lissauer and Clayden 2007). The causes of neonatal seizures differ slightly from those for children, although there are some common causes:
- Metabolic causes – hypoglycaemia, hypo-/hypernatraemia, hypocalcaemia, inborn errors of metabolism.
- Hypoxia.
- Hypoxic-ischaemic encephalopathy (HIE).
- Drug withdrawal (from maternal source).
- Kernicterus (condition in which bilirubin is deposited in brain tissue as a consequence of jaundice).
- Intracranial haemorrhage.
- Congenital infections – Group B streptococcus, hepatitis B and C, HSV, cytomegalovirus (CMV).
As noted, the clinical signs may be subtle in this age group, therefore frequent assessment of the neonate is essential if there are concerns about the possibility of seizure activity. Of concern are:
- Movement of the mouth in either a chewing motion or lip smacking.
- Grimacing.
- Deviation of the eyes or staring.
- Increased tone in one or more limbs.
- Hiccups not associated with feeds.
More general clinical signs include:
- Tachycardia.
- Tachypnoea.
- Bradycardia.
- Apnoeas.
- Posturing (abnormal flexion or extension of the limbs).
Children presenting in the intensive care unit with SE may well already be receiving anti-epileptic drugs (AEDs). Medications will vary according to type and presentation of seizures and the individual child’s response to treatment strategies. Common anti-convulsants recommended for use in the management of seizure disorders in infants and children include (www.nice.org.uk/CG020):
- Phenobarbitone (used in infants <1 month).
- Phenytoin.
- Sodium valproate.
- Gabapentin.
- Carbamazepine.
- Lamotrigine.
- Clobazam.
- Keppra (levetiracetam).
- Topiramate.
Status Epilepticus (SE)
SE is defined as a prolonged seizure lasting more than 30 minutes or recurrent seizures within a 30-minute period during which the infant or child does not regain consciousness. Such seizures are life-threatening and may result in permanent neurological injury. There can also be significant haemodynamic consequences of prolonged seizure activity which also contribute to potential long-term damage (Figure 7.2). The continued firing of the neurons increases the metabolic demands of the cerebral cells which eventually exceed the available nutrients leading to possible cell death.
Figure 7.2 The haemodynamic effects of prolonged seizures.
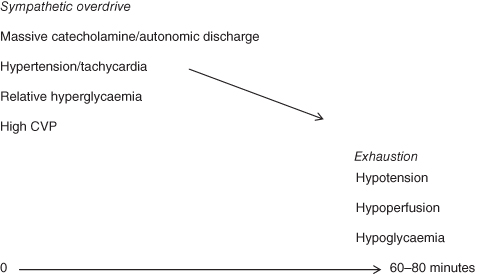
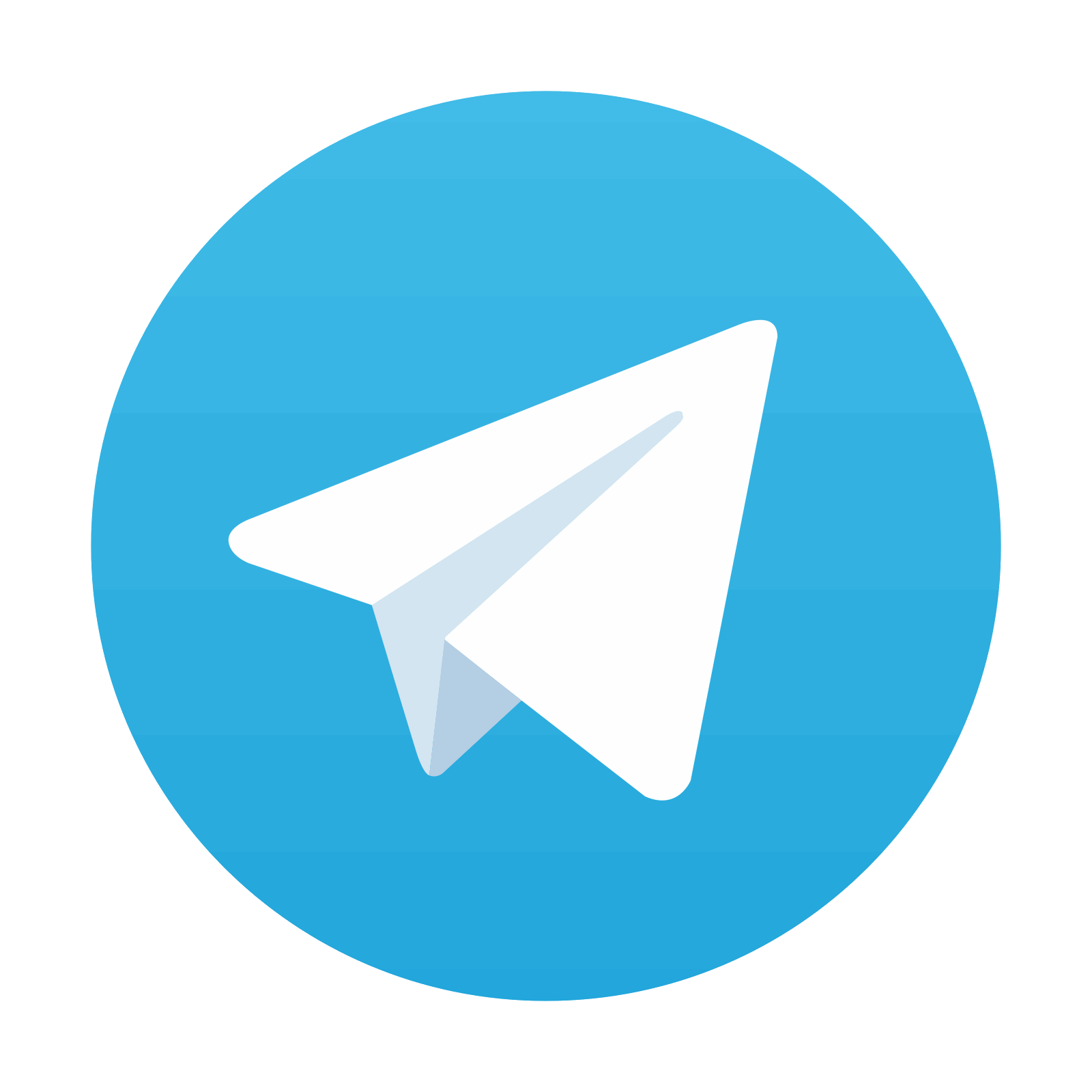
Stay updated, free articles. Join our Telegram channel

Full access? Get Clinical Tree
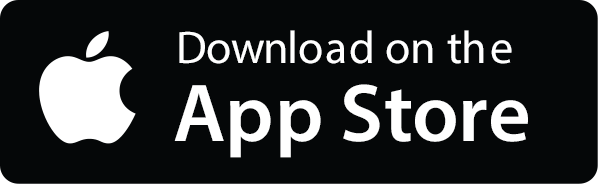
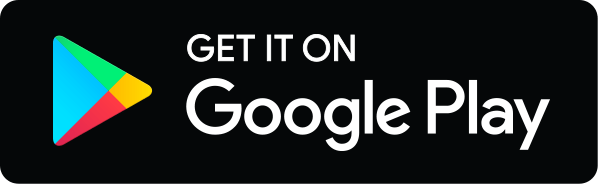