CHAPTER 9 1. Describe the mechanisms of glucose homeostasis in the fetus and newborn. 2. Discuss hypoglycemia and hyperglycemia in the neonate. 3. Discuss infants of diabetic mothers. 4. Differentiate neonatal diabetes from hyperglycemia. Organ systems, especially the human brain, are primarily dependent on glucose as their major energy source. Compared with adults, infants have a higher brain-to-body-weight ratio, resulting in a higher glucose demand in relation to glucose production capacity. Cerebral glucose utilization accounts for 90% of the neonate’s total glucose consumption. Continuous glucose and energy delivery to the fetus is provided from the maternal circulation via the placenta so there is no need for fetal glucose production in utero. An essential part of the neonate’s successful transition to extrauterine life therefore is the maintenance of euglycemia. Whereas most infants are indeed able to readily adapt to the metabolic demands of extrauterine life, newborns in general remain extremely susceptible to any condition that may impair their ability to establish normal glucose homeostasis during this transition process (Hume et al., 2005; Jain et al., 2012; Kalhan and Devaskar, 2011). Glucose is vital for cellular metabolism throughout the body. Blood glucose concentration is determined by the balance between intake/production of glucose and glucose use by the body. 2. The other main source of glucose is gluconeogenesis: production of glucose and glycogen in the liver by means of nonglucose precursors such as lactate, pyruvate, glycerol (fat), and amino acids (Hume et al., 2005; Kalhan and Devaskar, 2011). B. Glucose metabolism (Blackburn, 2013; Ogata, 2005). 2. In the brain, oxidized glucose provides 99% of the cerebral energy production, a process dependent on a number of important enzymes and reactions. b. Within the cytoplasm, glucose is metabolized by glycolysis to pyruvate. Pyruvate is then oxidized to acetyl-coenzyme A (acetyl-CoA), which is transported to the mitochondrion for entry into the citric acid cycle. The end products are carbon dioxide, water, and energy released in the generation of adenosine triphosphate (ATP). c. Of importance to the neonate is that during hypoglycemia other substrates (ketone bodies, lactate, glycerol, and amino acids) can also be converted to pyruvate, enter the citric acid cycle, and produce ATP, thus serving as a source of energy for the brain. C. Hormonal regulation of glucose homeostasis (Blackburn, 2013; Ogata, 2005). 2. Glucagon: Secreted by the pancreatic beta cells when blood glucose levels decrease, glucagon promotes glycogenolysis and gluconeogenesis. Glucagon is called a counterregulatory hormone because it opposes the effect of insulin by raising the blood glucose level. Other counterregulatory hormones include catecholamines, cortisol, and growth hormone. Although these hormones may not be important regulators in the fast-feed cycle of healthy neonates, minimum basal levels may be needed to maintain euglycemia. D. Fetal glucose homeostasis. 1. Glucose reaches the fetus by carrier-facilitated diffusion across the placenta at a concentration of about 70% to 80% of the maternal glucose concentration (Kalhan and Devaskar, 2011). 2. Glycogen storage for postnatal energy needs begins early in gestation, with most glycogen accumulating during the third trimester. 3. Fetal insulin is detectable by 8 to 11 weeks of gestation, but the response to a glucose load is not fully developed even at term (Blackburn, 2013; Stokowski, 2007). 4. The fetus is capable of gluconeogenic activity, using substrates such as lactate if needed to meet metabolic demands in utero. E. Neonatal glucose homeostasis. 2. In the first postnatal hours, the neonatal brain metabolizes lactate, which is abundant, so that even though the glucose concentration is low, the brain is not fuel deficient. 3. The neonate gradually mobilizes glucose to meet energy needs by secreting glucagon and catecholamines and suppressing insulin release. Thus, even if a healthy term newborn infant is not fed soon after birth, blood glucose levels rise at 3 to 4 hours of age. 4. Hepatic glycogen, however, is rapidly depleted if feeding is not established early and the infant is dependent on gluconeogenesis and lipolysis as the primary modes of maintaining euglycemia (Hume et al., 2005; Kalhan and Devaskar, 2011). A. Definition of hypoglycemia (Adamkin and Committee on Fetus and Newborn, 2011; Blackburn, 2013; Rozance and Hay, 2006). a. Conceptual and postmenstrual age. b. Adequacy of gluconeogenic pathways. c. General health status. d. Presence or absence of symptoms. 2. A widely used cutoff point for plasma glucose concentration is 40 mg/dL as the typical threshold for intervention in both premature and term neonates, with some authors advocating 55 to 70 mg/dL (Rozance and Hay, 2006; Steinkrauss et al., 2005). 3. The optimal range for plasma glucose is 70 to 100 mg/dL, and there is no evidence that neonates have a lower requirement for glucose concentrations, or that the neonatal brain can better tolerate hypoglycemia when compared to older infants and adults (Jain et al., 2012). B. Incidence. 1. Overall incidence is 1 to 5 per 1000 live births. 2. The incidence in at-risk infants may be as high as 30%, occurring in 8% of large-for- gestational-age (LGA) infants and in 15% of premature and small-for-gestational-age (SGA) infants (McGowan, 1999). C. Pathophysiology (Blackburn, 2013; Jain et al., 2012; Ogata, 2005). 2. Glucose delivery is dependent on blood glucose concentration and blood flow rate. During hypoglycemia, the brain increases blood flow to improve glucose delivery that may predispose the neonatal brain to hemorrhagic and hyperoxic injury if there is diminished cerebral autoregulatory ability. 3. When glucose consumption exceeds delivery, the brain uses alternate fuels such as ketone bodies, lactic acid, free fatty acids, and glycerol if they are available. The production of energy from these sources involves the use of brain structural components such as proteins and phospholipids that may play a contributory role in neuronal damage. 4. Lactic acid becomes elevated in late fetal and early postnatal life and healthy term infants produce ketones effectively on days 2 and 3 of life, thus protecting their brains from fuel deficiency if the blood glucose level falls while feeding becomes established. However, the ability of preterm infants and of infants who are SGA to mount a counterregulatory ketogenic response at any time is severely limited, so these infants are heavily dependent on an adequate glucose supply (Rozance and Hay, 2006). 5. Prolonged hypoglycemia, when not compensated by a supply of alternative fuels, induces biochemical changes at the cellular level that may damage the neuronal and glial cells of the brain. It is thought that an accumulation of excitatory amino acids, especially glutamate, during hypoglycemia leads to prolonged cellular depolarization with entry of water and calcium into the cell, first impairing neuronal growth and eventually causing cell death. In addition, the hypoglycemic brain may be more vulnerable to the damaging effects of ischemia. Degrees of ischemia and hypoglycemia that alone would not result in brain injury might do so in combination (Volpe, 2008). D. Etiologies and precipitating factors. 1. Inadequate production or supply of glucose accounts for the more common causes of neonatal hypoglycemia. These conditions involve decreased substrate (glycogen, lactate, glycerol, amino acids) availability, immature or altered enzyme pathways, or altered responses to neural or hormonal factors (Blackburn, 2013; Jain et al., 2012; Ogata, 2005). b. Intrauterine growth restriction: low glycogen and fat stores, increased substrate utilization. c. Delayed feedings, insufficient breastfeeding, or fluid restriction. d. Inborn errors of metabolism: defective gluconeogenesis and/or glycogenolysis (e.g., galactosemia, amino acid disorders, organic acid deficiencies, fatty acid oxidation disorders). e. Glycogen storage disease: autosomal recessive defects characterized by a deficient or abnormally functioning enzyme involved with formation or degradation of glycogen in the liver. f. Perinatal stress/hypoxia, respiratory distress, hypothermia, polycythemia/hyperviscosity, infection, adrenal hemorrhage, congestive heart failure. 2. Increased uptake of glucose related to hyperinsulinism (De Leon and Stanley, 2007; Steinkrauss et al., 2005). a. Infant of diabetic mother (IDM). b. Persistent neonatal hyperinsulinism and nesidioblastosis: autosomal recessive disorders thought to be caused by regulatory defects in beta cell function. Surgical exploration may be necessary for definitive diagnosis, with subtotal pancreatectomy the required therapeutic measure. c. Beckwith–Wiedemann syndrome: possibly genetic as majority of cases, but not all, involve a defect in the short arm of chromosome 11; characterized by omphalocele, macroglossia, visceromegaly, and hypoglycemia. Pancreatic islet cell hyperplasia is noted. The resultant hypoglycemia may be quite profound and difficult to treat (Blackburn, 2013; Kalhan and Devaskar, 2011). d. Rh incompatibility: severe cases can have associated beta cell hypertrophy and hyperinsulinemia. e. High glucose infusion and tocolytics used before delivery. β-Adrenergic agonists such as terbutaline can stimulate fetal pancreatic beta cells. f. Iatrogenic: position of tip of umbilical artery catheter near the pancreas can cause glucose to be directly delivered to the pancreas via the celiac artery, resulting in excessive insulin secretion. E. Clinical presentation and assessment (Blackburn, 2013; Jain et al., 2012; Ogata, 2005; Volpe, 2008). a. Tremors, jitteriness, irritability, exaggerated Moro reflex. b. Abnormal cry: high-pitched or weak. c. Respiratory distress: apnea, irregular respirations, tachypnea, cyanosis. d. Stupor, hypotonia, lethargy, refusal to feed. e. Hypothermia, temperature instability. f. Seizures. F. Diagnostic studies. 1. Point-of-care blood glucose screening. a. Enzymatic reagent strips. Their reliability is questioned because they: (2) May fail to detect clinically important hypoglycemia because of unpredictable measurement errors; and (3) Are sensitive to error from technical and operator variables such as timing, blotting, and distribution of blood droplets (Adamkin and Committee on Fetus and Newborn, 2011). b. Absorption photometry and electrochemical glucose meters. c. Subcutaneous microdialysis (Jain et al., 2012; Kalhan and Devaskar, 2011). (1) Decreases the need for frequent blood sampling. (2) Enables long-term glucose monitoring. (3) Cannot measure glucose levels below 45 mg/dL with accuracy; large variance seen with high glucose values. 2. All current techniques require laboratory confirmation for establishing a diagnosis of neonatal hypoglycemia; however, appropriate interventions should be initiated promptly and not wait on laboratory confirmation (Adamkin and Committee on Fetus and Newborn, 2011). 3. In suspected hyperinsulinism, additional testing may include concurrent insulin and glucose levels, ketone and free fatty acid levels, and cortisol and growth hormone levels. G. Patient care management (Adamkin and Committee on Fetus and Newborn, 2011; McGowan et al., 2011). 2. Prevent hypoglycemia by providing glucose substrate. b. Intravenous (IV) glucose at 4 to 6 mg/kg/min. 3. Assess glucose status with blood glucose screening. a. Perform blood glucose screening test on infants at risk on admission, frequently during the first 4 hours of life, and then at 4-hour intervals until the risk period has passed (Ogata, 2005). b. Clinically unstable infants and any infant with signs of a possible low blood glucose concentration require regular monitoring. c. Early and exclusive breastfeeding will meet the nutritional and energy needs of healthy full-term infants, and routine blood glucose screening of these infants is not presently recommended. 4. If hypoglycemia persists despite feeding, correction is with IV glucose infusion. A minibolus (dextrose 10% in water, 2 mL/kg), followed by continuous infusion at a rate of 6 to 8 mg/kg/min, rapidly raises the blood glucose level but does not treat the underlying hormonal and metabolic causes of the hypoglycemia. 5. Monitoring of blood glucose levels must then continue, for documentation of the resolution of hypoglycemia with IV therapy and subsequently during the transition to full enteral feedings. Oral feedings should be initiated once clinical symptoms have resolved (Rozance and Hay, 2006). 6. Persistent hypoglycemia raises the possibility of hyperinsulinism, although some infants without biochemical hyperinsulinism also have transiently high glucose requirements. Those with true hyperinsulinism may require the following: b. Hormonal therapy, which may include the following (Kalhan and Devaskar, 2011): (2) Diazoxide: suppresses pancreatic insulin secretion. Its use is reserved for situations of profound hyperinsulinism that have failed other therapies. It has been used for prolonged periods (years) without significant side effects noted. Usual dose 5 to 20 mg/kg/day administered orally 2 to 3 times per day. (3) Somatostatin: suppresses insulin and glucagon secretion; however, its use is limited by its extremely short half-life and short duration of action. c. Corticosteroids, which stimulate gluconeogenesis from noncarbohydrate (protein) sources (McGowan et al., 2011). (2) Gradual decreases in corticosteroid dosage and decreases in parenteral glucose concentrations are required as oral intake is increased. d. Subtotal or total pancreatectomy if severe, persistent hyperinsulinism is unresponsive to medical therapy (De Leon and Stanley, 2007; Lindley and Dunne, 2005). H. Complications. 1. Hypoglycemia will often recur when a bolus of glucose is not followed by a continuous infusion. 2. Extravasations of peripheral glucose infusions may cause necrosis of skin and other tissue (Lund and Kuller, 2007). b. Skin injury can be prevented by making multiple puncture holes using an aseptic technique over the involved area and allowing the fluid to be infiltrated. c. Elevation of the affected area, if an extremity, may limit the leakage of the exudate. d. Use of an occlusive dressing (hydrocolloid dressing; hydrogel sheets, and amorphous gel) will provide for exudate management, adherence without damaging the surrounding tissue, and a microbial barrier; débridement and compression should be used. 3. Reactive hypoglycemia with return of symptomatology may occur if the IV glucose infusion infiltrates or is stopped abruptly. I. Outcome. 1. Potential long-term sequelae remain unclear since there often are coexisting medical conditions that also predispose to brain injury (Ogata, 2005). 2. In general, outcome studies show that transient hypoglycemia in an otherwise healthy neonate has a good prognosis while neonates with seizure-associated and/or persistent hyperinsulinemic hypoglycemia have the worst neurologic prognosis (Kalhan and Devaskar, 2011; Rozance and Hay, 2006; Volpe, 2008). A. Incidence: 20% of infants of women with gestational diabetes mellitus and 35% of infants of mothers with other forms of diabetes (Blackburn, 2013). B. Pathophysiology: The hormonal and metabolic changes that complicate diabetic pregnancy can adversely affect the developing fetus and neonate in a number of ways (Blackburn, 2013; Stokowski, 2007). 2. Throughout pregnancy and particularly in the third trimester, the pregnant diabetic woman is increasingly insulin resistant and often has hyperglycemia and hyperaminoacidemia. Excess glucose and amino acids are freely delivered to the fetus, but maternal insulin is not. These nutrients stimulate the fetal pancreas to produce insulin to use the excess fuels, resulting in beta cell hyperplasia and hyperinsulinemia. This fetal hyperinsulinemia, in turn, stimulates protein, lipid, and glycogen synthesis, causing a high rate of fetal growth, increased deposition of fat and visceral enlargement (especially heart and liver), and subsequent macrosomia. This fetal macrosomia does not involve the brain or kidneys. 3. After birth, the neonate’s pancreas continues to produce insulin, and available glucose is rapidly used. The infant’s ability to mobilize glycogen stores is decreased. The IDM may exhibit an exaggerated and persistent hypoglycemia. Maternal glucose homeostasis during pregnancy as well as maternal glycemia during delivery influences the degree of neonatal hypoglycemia. 4. The IDM is at risk of having neural impairment because, even with plentiful adipose tissue, ketogenesis and lipolysis are suppressed by hyperinsulinemia, leaving the brain without a supply of alternative fuels for metabolism during hypoglycemia. C. Clinical presentation and assessment (McGowan et al., 2011). 2. Macrosomia/LGA (approximately 35% of IDMs): Some infants are SGA because of placental insufficiency in advanced stages of the maternal diabetes. 3. Increase in preterm births in association with diabetic pregnancy: Hyperbilirubinemia in IDMs may be secondary to prematurity. 4. Respiratory distress syndrome and other conditions such as transient tachypnea of the newborn infant: Fetal hyperinsulinemia may delay the maturation of various aspects of the pulmonary surfactant system and not just inhibit surfactant production, delaying lung maturation. 5. Polycythemia (venous hematocrit >65%): The insulin-induced high glucose uptake and high metabolic rate cause a cellular hypoxia, leading to an elevated erythropoietin level (Ogata, 2005). Newborns with polycythemia do not always look plethoric, so the hematocrit level must be measured. 6. Hypocalcemia, hypomagnesemia thought to result from a functional hypoparathyroidism due to maternal magnesium loss (Jain et al., 2012). 7. Cardiomyopathy, visceromegaly possibly related to maternal diabetes control and to fetal/neonatal hyperinsulinemia. 8. Congenital malformations: Cardiac defects (especially transposition), neural tube defects, sacral agenesis, and caudal regression are 2 to 4 times more frequent than in the general population. The anomalies are thought to be due to the diabetic intrauterine environment during organogenesis, frequently before the pregnancy is recognized and prenatal diabetic treatment initiated. D. Diagnostic studies. 1. Blood glucose screening with laboratory confirmation of plasma glucose. 2. Other laboratory analyses, including calcium and magnesium levels and venous hematocrit. 3. X-ray examination if fractures from traumatic delivery are suspected. 4. Echocardiography. E. Patient care management (McGowan et al., 2011; Stokowski, 2007). 2. Anticipate problems of IDM before delivery; prompt recognition and treatment of neonatal morbidities postdelivery. 3. Provide early feeding of human milk or formula, orally or via gavage tube if the infant does not feed well. IV administration of glucose is necessary for infants too small or sick to tolerate enteral feeding. F. Complications. 1. Seizures resulting from cerebral fuel deficiency. 2. Shoulder dystocia: Macrosomic infants delivered with forceps or vacuum extraction may have brachial plexus injury (Erb’s palsy) or fractures. 3. Renal vein thrombosis (secondary to polycythemia/hyperviscosity). 4. Development of juvenile insulin-dependent diabetes with a 2% transmission risk for female IDM and 6% for male IDM (Jain et al., 2012). G. Outcome (McGowan et al., 2011; Stokowski, 2007). 2. Morbidities associated with IDM include neurologic sequelae, developmental delay, behavioral differences, obesity, and diabetes. 3. Maternal complications, including poor glycemic control, vascular disease, infection, and pregnancy-induced hypertension, are associated with poorer perinatal outcome. Improved outcomes are seen in IDMs when maternal diabetes is metabolically controlled. 4. The outcomes for SGA infants born to women receiving intensive therapy for gestational diabetes may be worse than those of appropriate-for-gestational-age or LGA infants. B. Incidence: Prevalence of 29% to 86% in low birth weight infants overall; 2% of infants weighing more than 2000 g; 45% of infants less than 1000 g; and up to 80% of infants weighing less than 750 g (Jain et al., 2012; Le Compte et al., 2012; McGowan et al., 2011). C. Pathophysiology. 2. Hepatic glucose production in this latter group continues in the presence of hyperglycemia and circulating insulin. This represents a failure of glucose autoregulation involving both the pancreas and liver of infants with hyperglycemia. 3. Corticosteroid therapy stimulates glycogenolysis and gluconeogenesis and may block insulin secretion as well as inhibit its peripheral action. D. Etiologies and precipitating factors. 1. Low birth weight, extreme prematurity, and intrauterine growth restriction (IUGR). 2. Excessive glucose load (>6 to 8 mg/kg/min), with 50% of infants receiving a glucose infusion rate (GIR) of 11 mg/kg/min and all infants receiving a GIR of 14 mg/kg/min developing hyperglycemia. 3. Stress related to clinical problems such as sepsis or infection. 4. Transient or permanent neonatal diabetes mellitus (see “Transient or Permanent Neonatal Diabetes”). 5. Side effects of medications such as corticosteroids. 6. Lipid infusion, which may contribute to hyperglycemia. 7. Release of catecholamines secondary to surgery/anesthesia. E. Clinical presentation and assessment. 1. Onset can be as early as 24 hours of age; usually before 3 days of life. 2. No characteristic clinical presentation. 3. Symptoms may include glycosuria, dehydration, weight loss, fever, ketosis, metabolic acidosis and failure to thrive. F. Diagnostic studies. 1. Serum or plasma blood glucose levels greater than 125 mg/dL or 150 mg/dL, respectively. 2. Urinary glucose. Very low birth weight (VLBW) infants have a low renal threshold for glucose and may spill sugar at blood glucose levels as low as 80 to 100 mg/dL. 3. Investigation for possible underlying cause (sepsis workup to rule out infection or insulin level to rule out neonatal diabetes mellitus). G. Patient care management. 2. Decrease glucose load as much as possible to allow the blood glucose level to stabilize in a normal range (<125 mg/dL, or plasma glucose <150 mg/dL). 3. Monitor weight, urine output, fluid intake, GIR, electrolytes, and acid–base balance. 4. Insulin is sometimes administered to ELBW infants along with parenteral nutrition in an attempt to normalize blood glucose levels without reducing the caloric intake. Insulin is also used to treat transient neonatal diabetes. Insulin normalizes blood glucose by suppressing hepatic glucose production and by increasing peripheral glucose utilization. However, premature infants have a very small mass of insulin-dependent tissue, with only 10% of their glucose utilization being insulin dependent. Severe hyperglycemia may occur in the VLBW infant despite decreased glucose infusion rates. Continuous insulin infusion may be used, although routine use not advised because of adverse effects (Alsweiler et al., 2012). 5. Begin enteral feedings when feasible because the subsequent release of gut hormones may promote insulin secretion, allowing for improved blood glucose control. H. Complications. 1. Neonatal hyperglycemia may be accompanied by urinary loss of glucose and an osmotic diuresis with its risk of dehydration. In addition, possible resultant hyperosmolarity of extracellular fluid in the brain may increase the premature infant’s chance for intraventricular hemorrhage (IVH) (Jain et al., 2012). 2. Insulin management may be difficult in the ELBW infant; blood glucose levels can fluctuate widely. A precisely controlled continuous-infusion pump is essential. Priming of the tubing is required because insulin tends to adhere to the catheters. Whether insulin therapy promotes linear growth or just converts glucose into fat is not yet fully understood (Alsweiler et al., 2012). 3. Electrolyte abnormalities, elevated CO2 retention, and an elevated triglyceride level. I. Outcome. 1. Controversy exists concerning the outcome of neonatal hyperglycemia. b. There is a strong association between neonatal hyperglycemia and increased mortality rates or poor neurodevelopmental outcomes among survivors (Hays et al., 2006; Kao et al., 2006). c. Neonatal hyperglycemia is usually self-limiting and not associated with adverse outcomes. d. Possible association between hyperglycemia and severe retinopathy of prematurity in ELBW infants (Ertl et al., 2006). A. Definition: Hyperglycemia requiring insulin therapy occurring within the first month of life and lasting at least 2 weeks to several months can be lifelong (Ozlu et al., 2006). B. Incidence: 1:500,000. Predominantly occurring in SGA infants, with 46% developing permanent diabetes in the neonatal period, 23% in childhood or adolescence; 31% are resolved in the neonatal period (Ogata, 2005). 1. Appears in the first week of life and persists for greater than 2 weeks (Stokowski, 2007). 2. Etiology is variable, including dysfunctional insulin molecule or receptor deficiencies and activating mutations of the KATP channel (Stokowski, 2007). 3. Instances of transient neonatal diabetes are associated with abnormalities of an imprinted region on chromosome 6q24. Mutations in encoder genes have been attributed to instances of permanent and transient neonatal diabetes (Babenko et al., 2006). C. Pathophysiology. 1. Both transient and permanent neonatal diabetes mellitus are due to a failure of pancreatic beat cells causing an endogenous insulin deficiency, with the exact pathogenesis involved not yet fully understood (Ozlu et al., 2006; Stokowski, 2007). 2. The IUGR commonly seen with affected infants is due to insufficient insulin secretion (Stokowski, 2007). D. Clinical presentation. 1. The transient form appears within the first week of life, resolves within weeks or months, and reappears in late childhood. The permanent form appears within days or months of birth and persists throughout life (Stokowski, 2007). 2. Hyperglycemia (frequently >600 mg/dL), polyuria, glycosuria, weight loss, dehydration, fever, failure to thrive, ketosis, metabolic acidosis (may or may not be evident), and low levels of C-peptide and plasma insulin (Ozlu et al., 2006; Stokowski, 2007). E. Patient care management. 1. Restore intravascular volume. 2. Replace fluid and electrolyte losses as indicated and provide for ongoing needs. 3. Correct the hyperglycemia; monitor glucose levels. 4. Provide adequate nutrition for growth and development. 5. Usually a daily dose of 0.2 to 3 units/kg/day of insulin is necessary to achieve plasma glucose levels of 100 to 180 mg/dL; however, individualization of insulin requirement is needed. 6. Average length of insulin therapy varies from 2 weeks to 18 months. Tapering of insulin dose as requirement decreases lessens the risk of recurrent hyperglycemia (Ozlu et al., 2006; Stokowski, 2007). F. Complications: See Hyperglycemia section above. G. Outcome. 1. Course of disease is highly variable. 2. Close follow-up is recommended after remission because of a high rate of recurrence later in childhood.
Glucose Management
GLUCOSE HOMEOSTASIS
HYPOGLYCEMIA
INFANT OF DIABETIC MOTHER
HYPERGLYCEMIA
TRANSIENT OR PERMANENT NEONATAL DIABETES
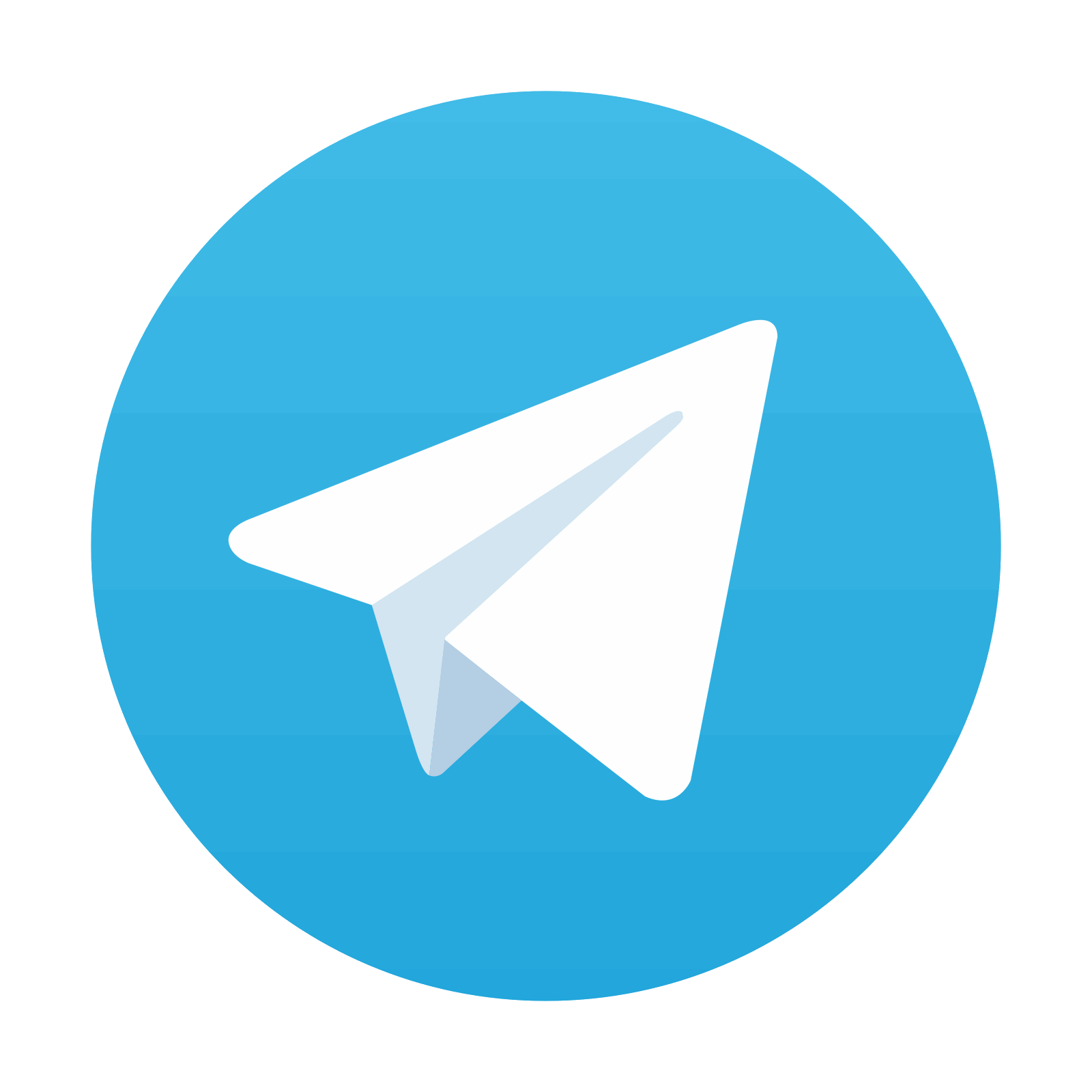
Stay updated, free articles. Join our Telegram channel

Full access? Get Clinical Tree
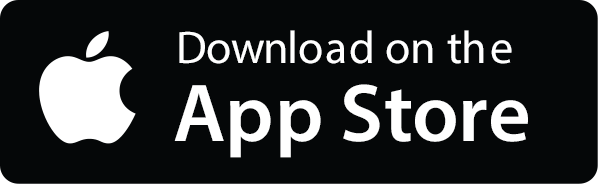
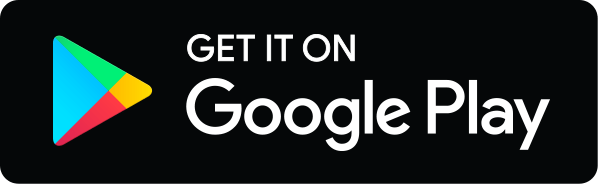