Chapter 1 Peter S. Vickers The aim of this chapter is to introduce the reader to the various cells and tissues of the body in order to develop their insight and understanding. What is a cell? Put simply, a cell is a building block for the formation of all life and, particularly in this case, for the formation and development of the human body. There are many different types of cells and they play different roles in both the structure and functioning of the body. For example, certain cells come together to form skin (a tissue), which acts as a cover and protector for our internal organs (tissues). Other cells combine to form bone (tissue) and hence our skeleton. Then there are other different cells which combine to make up the brain and neurological tissue (nerves). Outside the cells that form our structure are the cells that help to keep us functioning, for example, the cardiac cells, which combine to make the heart (tissue), which in turn keeps blood (cells and a tissue) flowing around our body carrying nutrients to all our cells and tissues and removing waste products from them. Some cells are involved in protecting us from infectious organisms, whilst others form muscles (tissues) which allow us to work and move. So, it can be seen that cells are the basic building blocks of our bodies – indeed, our very ‘being’. All these different types of cells are actually produced from just two cells – ovum and sperm – which fuse together at the moment of conception. Within those two cells are all the plans and schemata for producing the number and diversity of cells that make a human body – truly a miracle! Once they fuse together at conception, they begin to multiply and divide into the different types of cells. This manufacture and diversification of cells is dictated by the genes carried in all of our cells (see Chapter 2, Genetics). This chapter will give a brief overview of the structure of cells and their roles within the body. In addition, it will discuss some of the problems that can occur and how these can affect the working and health of the body, commencing with the common characteristics of cells (Fig. 1.1). Figure 1.1 Examples of different types of cells in the body. Source: Tortora & Derrickson 2009, in: Peate & Gormley‐Fleming 2015. Reproduced with permission of Wiley. There are four main compartments of the cell: Within these compartments are many organelles (or small organs). These organelles perform numerous roles to keep cells alive and functioning. As can be seen in Fig. 1.2, the various structures of the cell are contained within a cell membrane (also known as the plasma membrane). This cell membrane is a semi‐permeable biological membrane separating the interior of the cell from the outside environment, and protecting the cell from its surrounding environment. It is semi‐permeable because it allows only certain substances to pass through it for the benefit of the cell itself. For example, it is selectively permeable to certain ions and molecules (Alberts et al., 2014). Inside the cells are the cytoplasm and the organelles, which include, for example, the lysosomes, mitochondria, and the nucleus of the cell. Figure 1.2 Structure of the cell. Source: Tortora & Derrickson 2009, in: Peate & Gormley‐Fleming 2015. Reproduced with permission of Wiley. The cell membrane, which can vary in thickness from 7.5 nm (nanometres) to 10 nm (Vickers, 2009) is made up of a self‐sealing double layer (bilayer) of phospholipid molecules with protein molecules interspersed amongst them (Fig. 1.3). A phospholipid molecule consists of a polar ‘head’, which is hydrophilic (mixes with water), and a tail that is made up of non‐polar fatty acids, which are hydrophobic (repel water). In the bilayer of the cell membrane, all the heads of each phospholipid molecule are situated on the outer and inner surfaces of the cell facing outwards, whilst the tails point into the cell membrane; it is this central part of the cell membrane consisting of hydrophobic tails that makes the cell impermeable to water‐soluble molecules (Marieb, 2014). In addition to the phospholipid molecules, the cell membrane contains a variety of molecules, mainly proteins and lipids, and these are involved in many different cellular functions, such as communication and transport. The proteins inserted within the cell membrane are known as plasma member proteins (PMPs), which can be either integral or peripheral. Integral PMPs are embedded amongst the phospholipid tails whilst others completely penetrate the cell membrane. Some of these integral PMPs form channels for the transportation of materials into and out of the cell, others bind to carbohydrates and form receptor sites (e.g., attaching bacteria to the cell so they can be destroyed). Other examples of integral PMPs include those that transfer potassium ions in and out of cells, receptors for insulin, and types of neurotransmitters (Vickers, 2015). On the other hand, peripheral PMPs bind loosely to the membrane surface, and so can be easily separated from it. The reversible attachment of proteins to cell membranes has been shown to regulate cell signalling, as well as acting as enzymes to catalyse cellular reactions through a variety of mechanisms (Cafiso, 2005). Figure 1.3 Cell membrane. Source: Tortora & Derrickson 2009, in: Peate & Gormley‐Fleming 2015. Reproduced with permission of Wiley. Briefly, the two major physiological functions of the cell membrane are endocytosis and exocytosis. These are both concerned with the transport of fluids and other essential particulates and waste matter into and out of the cell. Endocytosis involves part of the cell membrane being drawn into the cell interior, along with particulates or fluid, in order to facilitate their ingestion. This part of the membrane is then ‘pinched off’ to form a vesicle within the cell. At the same time, the cell membrane reseals itself. Once inside the cell, the fate of this vesicle depends upon the type of endocytosis involved and the material that is contained within the cell membrane surrounding it. In some cases, the vesicle may ultimately fuse with a lysosome (an organelle), following which the ingested material can be processed. Endocytosis is also the means by which many simple organisms – such as amoeba – obtain their nutrients. Selective permeability, as mentioned in the previous section, is very important to the process of transporting materials into and out of the cell, allowing certain materials to pass through the membrane, whilst preventing others that could harm the cell. This process depends upon the hydrophobicity of some of its molecules (as mentioned earlier). Because the phospholipid molecule tails are composed of hydrophobic fatty acid chains, it is difficult for hydrophilic (water‐soluble) molecules to penetrate the membrane. Hence it forms an effective barrier for these types of molecules, which can only be penetrated by means of specific transport systems that control what can enter or leave the cell. For example, the membrane controls the process of metabolism by restricting the flow of glucose and other water‐soluble metabolites into and out of cells – as well as between subcellular compartments. In addition, the cell stores energy in the form of transmembrane ion gradients by allowing high concentrations of particular ions to accumulate on one side of the membrane. Ions can pass through the membrane from inside the cell to the outside – or vice versa – so that there are more supplies of these ions just outside the cell or inside it. The membrane controls the speed/rate at which these ions pass through the membrane. The controlled release of such ions on the gradients can be used for: There are four factors involved in the degree of permeability of a cell membrane, namely: There are two ways for this to occur, namely, passive and active. A passive process is one in which the substances move under their own volition down a concentration gradient from an area of high concentration to an area of lower concentration. In this process, the cell expends little energy on the process (like rolling down a hill). There are four types of passive transport processes, namely: Diffusion is the most common form of passive transport. A substance in an area of higher concentration moves to an area of lower concentration (Colbert et al., 2012). The difference seen between areas of different concentrations is known as the concentration gradient. This particular passive transport process is essential for respiration. It is through diffusion that oxygen is transported from the lungs to the blood and carbon dioxide from the blood into the lungs. Although similar to diffusion, facilitated diffusion differs from it by the use of a substance (a facilitator) to help in the process (see Fig. 1.4). As an example, glucose is moved using this process. To be able to pass through a membrane, glucose needs to attach itself to a carrier/transport protein (McCance & Huether, 2014). Figure 1.4 Facilitated diffusion. Source: Tortora & Derrickson 2009, in: Peate & Gormley‐Fleming 2015. Reproduced with permission of Wiley. Osmosis is the process by which water travels through a selectively permeable membrane so that concentrations of a solute (a substance that is soluble in water) are equal on both sides of the membrane. This gives rise to osmotic pressure. The higher the concentration of the solute on one side of the membrane, the higher the osmotic pressure available for the movement of water (Colbert et al., 2012). If osmotic pressure rises too much, then it can cause damage to the cell membrane, so the body attempts to ensure that there is always a reasonable constant pressure between the cell’s internal and external environments. We can see the possible damage if, for example, a red blood cell is placed in a low concentrated solute, then it will undergo haemolysis. On the other hand, if it is placed in a highly concentrated solute, the result will be a crenulated cell. If the red blood cell is placed in a solution with a relatively constant osmotic pressure, it will not be affected because the net movement of water in and out of the red blood cell is minimal. Filtration is similar to osmosis, with the exception that physical pressure is used in order to push water and solutes across a cell membrane. This is seen in renal filtration, where the heart beating exerts pressure as it pushes blood into the kidneys, where filtration of the blood can then take place to remove any impurities (Colbert et al., 2012). Active processes are: An active process is one in which substances move against a concentration gradient from an area of lower to higher concentration. In order for this to happen, the cell must expend energy, which is released by the splitting of adenosine triphosphate (ATP) into adenosine diphosphate (ADP) and phosphate. ATP is a compound of a base, a sugar and three phosphate groups (triphosphate), and is held together by phosphate bonds, which release a high level of energy when they are broken. Once one of the phosphate bonds is broken and phosphate has been released, that compound then becomes ADP. The ‘spare’ phosphate will then join another ADP group, so forming ATP (with energy stored in the phosphate bond). This process is continually recurring within the body. Active transport pumps need energy to be able to function. This energy occurs as a result of the reaction mentioned earlier. It is necessary when the body is attempting to move an area that already has a high concentration of that substance. The higher the concentration already present, the more energy is required to move further molecules of that substance into that area. Fig. 1.5 demonstrates the effect of solute concentration on a red blood cell. Figure 1.5 Effect of solute concentration on a red blood cell. Source: Tortora & Derrickson 2009, in: Peate & Gormley‐Fleming 2015. Reproduced with permission of Wiley. These are rather like small ‘organs’ within the cells. The following sections give a brief overview of the many cell organelles and their functions. Although, not strictly speaking, an organelle, the cytoplasm is a very important and integral part of the cell interior. Cytoplasm is ground substance (a ‘matrix’) in which various cellular components are found. It forms part of the protoplasm of the cell (protoplasm is the collective name for everything within a cell). Cytoplasm is a thick, semi‐transparent, elastic fluid containing suspended particles along with the cytoskeleton (the cell framework). The cytoskeleton provides support and shape to the cell and is involved in the movement of structures within the cytoplasm – for example, phagocytic cells. Chemically, cytoplasm is made up of 75–90% water along with solid compounds, particularly carbohydrates, lipids and inorganic substances. The cell nucleus is the control centre of a family of cells known as eukaryotes. Eukaryotic cells are found in animals (including humans) and plants. These cells include the prokaryotic cells that are very typical of bacteria – these cells tend to be less complex and often smaller than eukaryotic cells. However, not all human cells have a nucleus. A good example is the red blood cell. The mature red blood cell has lost its nucleus and consequently is concave in shape because it has ‘collapsed in’ on itself. There are also some human cells (some muscle fibre cells) that have more than one nucleus (see Fig. 1.1). In order for the body to grow and also for the replacement of body cells that die or are damaged, our cells must be able to reproduce themselves. In order that the genetic information contained in the cells of the body is not lost, this must be achieved accurately. As mentioned earlier, in humans there are a total of 23 pairs of chromosomes in each human cell that contains a nucleus. Cells reproduce by means of two processes: mitosis and meiosis, with most cells being reproduced by mitosis, whilst meiosis is restricted to the gender cells, namely the spermatozoa and the ova. The majority of the cells that are reproduced by mitosis are exact replicas of the parent cells, and contain the full complement of 23 pairs of chromosomes, whilst those cells reproduced by meiosis are totally different in that they only contain one of each of the 23 chromosomes. To ensure that all the genetic information is passed on accurately, in mitosis, the chromosomes reproduce themselves and then the cell divides into two, ensuring that one pair of each of the chromosomes is found in each new cell. Meiosis, however, is different in that, although initially the process is the same as in mitosis, the cells then undergo other procedures so that the end product is cells with only one copy of each chromosome. This is essential for the reproduction, not of cells, but of humans, as explained briefly in the following paragraph. During the reproduction of humans, an egg (ovum) is penetrated by a sperm (spermatozoa), which then releases its chromosomes containing DNA which will then combine with the DNA of the egg. Because these two cells (ovum and spermatozoa) only contain one copy of each chromosome rather than the two carried by all other cells of the body, the ovum will only have two copies of each chromosome containing DNA. If the process of meiosis did not take place and the egg and sperm were like all the other cells in the body and had the normal two copies of each chromosome, then the resulting embryo would end up with four copies of each chromosome. If this process was repeated, then the next generation would end up with eight copies of each chromosome, and the following generation with 16 copies …, and so on. This is obviously not practical, which is why the ova and spermatozoa undergo meiosis to ensure that only two copies of the chromosomes are present in each succeeding generation. This will be explored more fully in Chapter 2. All cells contain many organelles, and these are discussed in the following sections. The endoplasmic reticulum (ER) consists of membranes that form a series of channels known as cisternae (see Fig. 1.6). These divide the cytoplasm into compartments. There are two types of cisternae: Figure 1.6 Endoplasmic reticulum. Source: Tortora & Derrickson 2009, in: Peate & Gormley‐Fleming 2015. Reproduced with permission of Wiley. Ribosomes include tiny particles of RNA – these are formed in the cell nuclei and are associated with the synthesis of proteins need by the cell. The Golgi complex (also known a Golgi apparatus) is a collection of membranous tubes and elongated sacs. These are actually flattened cisternae that are stacked together. The Golgi complex has two major roles: Proteins for export from the cell are, first of all, synthesised on the ribosomes. They then travel through the ER to the Golgi vesicles (a vesicle is a fluid‐filled sac). The vesicles leaving the Golgi complex then fuse with the cell membrane by the process of exocytosis. This allows the contents of the vesicles to be exported out of the cell. In addition, the Golgi complex is itself involved in the formation of glycoproteins. Lysosomes are organelles that are bound to the cell membrane and they contain a variety of enzymes. They have a number of functions: It is crucially important that lysosomes do not rupture and release their contents inside living cells that we need to function, otherwise the lysosomal enzymes would start to digest and destroy the cell that is needed. If this occurs, the results can be seen in certain degenerative diseases, such as rheumatoid arthritis. The rupturing and breaking down of lysosomes from macrophages causing the release of lysosomal enzymes may be a significant factor in the attacking and destruction of essential living cells and tissues. Lysosomes also contribute to the production of hormones, such as thyroxine. Thyroxine is a hormone that affects a wide range of physiological activities, such as the rate of metabolism throughout the body. Peroxisomes are organelles that are similar in structure to lysosomes. However, they are much smaller. These organelles are particularly abundant in the cells of the liver, and they contain several enzymes that are toxic to cells of the body. The role of peroxisomes in cells appears to be one of detoxification of harmful substances – such as alcohol and formaldehyde – within the cell. Importantly, they also neutralise dangerous free radicals. Free radicals are highly reactive chemicals that contain electrons that have not been ‘paired off’, and so are ‘free’ to disrupt the structure of molecules (Marieb, 2014). Mitochondria (mitochondrion, singular) are often thought of as the ‘power houses’ of the cell because they generate most of the cell’s supply of adenosine triphosphate (ATP), used as a source of energy. The mitochondria are often found concentrated in regions of the cell associated with intense metabolic activity. Anatomically, mitochondria consist of two membranes (an inner and an outer) and an intermembrane space. The inner membrane has many folds (cristae) that increase the surface area available for chemical reactions to occur, such as the production of ATP (adenosine triphosphate – a coenzyme used as an energy carrier in the cells of all known organisms, which is responsible for the process in which energy is moved throughout the cell). This process is collectively known as internal respiration. The inner membrane is of the same thickness as the outer membrane and is responsible for oxidative phosphorylation. The mitochondrial matrix is the name given to the space that is surrounded by the inner membrane. It contains enzymes of the tricarboxylic acid (TCA) cycle, as well as those enzymes involved in fatty acid oxidation. About two‐thirds of the total protein is found in mitochondria, and, with the inner membrane, they play an important role in the production of ATP, and contain a very concentrated mixture of hundreds of enzymes. The intermembrane space: Because the outer membrane is freely permeable to small molecules, there is a high concentration of small molecules, such as ions and sugars. However, larger molecules cannot enter this space unless they possess a specific signalling code that allows them to be able to pass through the outer membrane. The outer mitochondrial membrane encloses the entire organelle. It contains large numbers of integral membrane proteins, known as porins, which form channels in the membrane to allow small molecules to diffuse easily from outside the mitochondria to the intermembrane space, and vice versa. Any disruption of the outer membrane allows proteins in the intermembrane space to leak into the intercellular fluid in the cell (cytosol), leading to certain cell death. By using ATP, the mitochondria are able to generate the energy needed by the cell for it to be able to function by converting the chemical energy contained in molecules of food. The production of ATP, therefore, requires the breakdown of food molecules, and it occurs in several stages, each requiring the appropriate enzyme. Note that an enzyme is a protein that can initiate and speed up a chemical reaction (it acts as a catalyst). The enzymes in the mitochondria are stored in the membranes in the required order so that the chemical reactions occur in the correct sequence. This mechanism is very important, as it would be disastrous if the chemical reactions occurred out of sequence. Mitochondria are self‐replicating, in that, although most of a cell’s DNA is contained in the cell nucleus (see Chapter 2), the mitochondrion has its own independent genetic organisation that is really quite similar to that of bacteria. DNA that is incorporated into the mitochondrial structure controls its own replication system. The cytoskeleton is a lattice‐like collection of fibres and fine tubes and these are found in the cytoplasm of the cell. It is involved with the cell’s ability to maintain and alter its shape as required (see Fig. 1.7). Figure 1.7 The cytoskeleton. Source: Peate & Gormley‐Fleming 2015. Reproduced with permission of Wiley. There are three components that make up the cytoskeleton: Microfilaments are rod‐like structures that are approximately 6 nanometres (6 nm) in diameter and are made of a protein called actin. In muscles, both actin (which is thick) and another protein – myosin (which is thin) combine together to allow the contraction of muscle fibres. In non‐muscle cells, microfilaments help to provide support and shape to the cell. Microfilaments also assist in the movement of the cells themselves, as well as movement within the cells. Microtubules are relatively straight, slender cylindrical tubules that range in diameter from 18 to 30 nm. They consist of another protein – tubulin. These help to determine the shape of a cell, and range in size from 8 to 12 nm. Examples of intermediate filaments are neurofilaments, which are found in nerves. These cylindrical structures are found in most animal cells, and are composed of nine sets of microtubule cylinders arranged in a circular pattern. They are particularly involved in cell reproduction. Centrioles are involved in the organisation of the mitotic spindle (see Chapter 2). These ‘hair‐like’ structures extend from the surface of some cells. They possess the facility to bend, which causes the cells to move. In humans, cilia generally have the function of moving fluid or particulates over the surface of the cells. However, ciliated cells of the respiratory tract have a very important role to play in our immune and respiratory system in that they are able to move mucus that has trapped foreign particles, including bacteria and viruses, over the surface of respiratory tissues and towards the mouth or nose, thus preventing them from causing illness. A flagellum (singular of flagella) is usually a much larger structure than a cilium (singular of cilia), and it is often used like a fish’s tail to propel the cell forward. The only example of a human cell with a flagellum is a spermatozoon, where the flagellum acts as a tail and propels the spermatozoon towards an ovum. This ends the section on cells; an understanding of them and their roles in the anatomy and physiology of bodies is of utmost importance in allowing us to understand how the human body functions. The next section in this chapter will look at the various structures and organs of our bodies – all of which are made up of billions of cells. Each of us, as humans, began life as a single cell – a fertilised egg. As soon as fertilisation takes place, the egg divides continuously into many cells by division, leading to the development of an embryo, then a fetus, and finally to a baby (see Chapter 2). However, these cells do not just divide endlessly and haphazardly. Rather, they divide and grow together in such a way that they become specialised and form, for example, muscle cells, skin cells, blood cells, and so on. These specialised cells then group together to become tissues, which themselves join with other tissues to form a human being. So tissues are simply groups of cells that are similar in structure and generally perform the same functions (McCance & Huether, 2014). There are four primary types of tissues: These four primary tissue types then interweave to form the fabric of the body (Marieb, 2014). Each of the four types of tissue has a specific role to perform within the body. In simple terms: These specialist cells form themselves into tissues in one of two ways. Epithelial tissue lines and covers areas of the body – both outside and inside, as well as forming the glandular tissue of the body. Thus, the exterior of the body (i.e., skin) is covered by one type of epithelial tissue whilst other types of epithelial tissue line digestive organs, such as the stomach and the small intestines, along with the kidneys, and so on. So it can be seen that epithelial tissue covers or lines most of the internal and external surfaces of the body. Epithelial tissue is classified in two ways: Figure 1.8 Simple epithelium. Source: Nair & Peate 2013, in: Peate & Gormley‐Fleming 2015. Reproduced with permission of Wiley. Figure 1.9 Stratified epithelium. Source: Nair & Peate 2013, in: Peate & Gormley‐Fleming 2015. Reproduced with permission of Wiley. Simple epithelial tissue is most concerned with absorption, secretion and filtration of fluids and particulates. However, because this tissue is usually very thin, it is not involved in protection. Simple squamous epithelium rests on a basal layer known as the ‘basement membrane’. This is composed of a structural material that is secreted by the cells themselves (Marieb, 2014). The basement membranes provide a layer of cells that supports and separates epithelial tissue from the underlying connective tissue (Fig. 1.10). The squamous epithelial cells fit very closely together to give a thin sheet forming the tissue. This type of epithelial tissue is found in the alveoli of the lungs as well as in the walls of capillaries. It is this very thin tissue that easily allows for rapid diffusion into and out of the cell, thus facilitating oxygen and carbon dioxide exchange through the epithelial tissue that is lining the alveoli of the lungs, and allowing nutrients and gases to pass easily through the epithelial tissue from the cells into the capillaries – and vice versa. Figure 1.10 Connective tissue reinforces epithelial tissue. Source: Tortora & Derrickson 2009, in: Peate & Gormley‐Fleming 2015. Reproduced with permission of Wiley. Simple squamous epithelial cells also form the serous membranes that line certain body cavities and organs. Simple cuboidal epithelial tissue consists of one layer of cells resting on a basement membrane (Fig. 1.11). However, because cuboidal epithelial cells are thicker than squamous epithelial cells, they are found in different places within the body and also perform different functions. This type of epithelial tissue is to be found in glands, such as the salivary glands and the pancreas. In addition, they form the walls of the kidney tubules as well as covering the surface of the ovaries (Marieb, 2014). Figure 1.11 Simple cuboidal. Source: Tortora & Derrickson 2009, in: Peate & Gormley‐Fleming 2015. Reproduced with permission of Wiley. Simple columnar epithelium (Fig. 1.12) is the third type of simple epithelial tissue. Like the other types of simple epithelium, it is composed of a single layer of cells, although these cells are relatively tall. However, similar to the other types, they still fit closely together. It is this type of epithelial tissue, which also contains goblet cells, that lines the entire length of the digestive tract from the stomach to the anus. Goblet cells produce mucus, and so, consequently, the simple columnar epithelial tissues that line all the body cavities that are open to the exterior of the body are known as mucous membranes (Marieb, 2014). Figure 1.12 Simple columnar. Source: Tortora & Derrickson 2009, in: Peate & Gormley‐Fleming 2015. Reproduced with permission of Wiley. Stratified epithelial tissue, unlike simple epithelial tissue, consists of two or more cell layers – so they lie in strata (hence the name). Because these stratified epithelial tissues have more than one layer of cells, they are stronger and hardier than simple epithelia. As a consequence, a primary function of stratified epithelia is protection. Stratified squamous epithelial tissue consists of several layers of cells and is the most common stratified epithelium found within the human body (Marieb, 2014). Despite being called ‘squamous epithelium’, it is not composed entirely of squamous cells. Only the cells at the free edges of the epithelial tissue are squamous cells, while those cells that are close to the basement membrane are made up of either cuboidal or columnar cells. This tissue is to be found in parts of the body that are most at risk of everyday damage, such as the oesophagus, the mouth, and the outer layer of the skin. Stratified cuboidal epithelial tissue has just two layers of cells and is only found in the ducts of large glands, such as the sweat glands, mammary glands, and salivary glands where its role is to protect the ducts of these glands. Stratified columnar epithelial tissue is also found only in a few particular places in the body, such as in the conjunctiva of the eye, parts of the pharynx and anus, the uterus, and the male urethra and vas deferens. It is also to be found in ducts located within the salivary glands. Transitional epithelium is a highly modified stratified squamous epithelium and forms the lining of just a few organs and other structures – all of which form part of the urinary system, namely the urinary bladder, the ureters, and part of the urethra. This tissue has been modified so that it can cope with the considerable stretching that takes place within these organs. When one of these organs/structures is not being stretched, the tissue is seen to have many layers, with the superficial cells (i.e., those in the top layer) having a rounded appearance like domes. However, when one of these organs/structures is distended with urine, then the epithelium becomes thinner as the surface cells flatten and become just like normal squamous cells. These transitional cells are able to slide past one another and change their shape, thus allowing the wall of the ureters, for example, to stretch as a greater volume of urine flows through it. This type of ‘elastic’ epithelium allows for more urine to be stored in the bladder, until micturition takes place.
The cell and body tissue
Aim
CELLS
Introduction
Characteristics of cells
The structure of the cell
The cell membrane
Functions of the cell membrane
The cell membrane and transport
Cell membrane permeability
Movement of substances across the membrane
Passive processes
Active processes
The organelles
Cytoplasm
The role of the cytoplasm
The nucleus (see Fig. 1.2)
Some facts about the nucleus
Cell reproduction
Other organelles
Endoplasmic reticulum
Golgi complex (Fig. 1.2)
Lysosomes (Fig. 1.2)
Peroxisomes (Fig. 1.2)
Mitochondria (Fig. 1.2)
The cytoskeleton
Microfilaments
Microtubules
Intermediate filaments
Centrioles
Cilia and flagella
Conclusion
TISSUES
Introduction
Types of tissues
Epithelial tissue
Classification
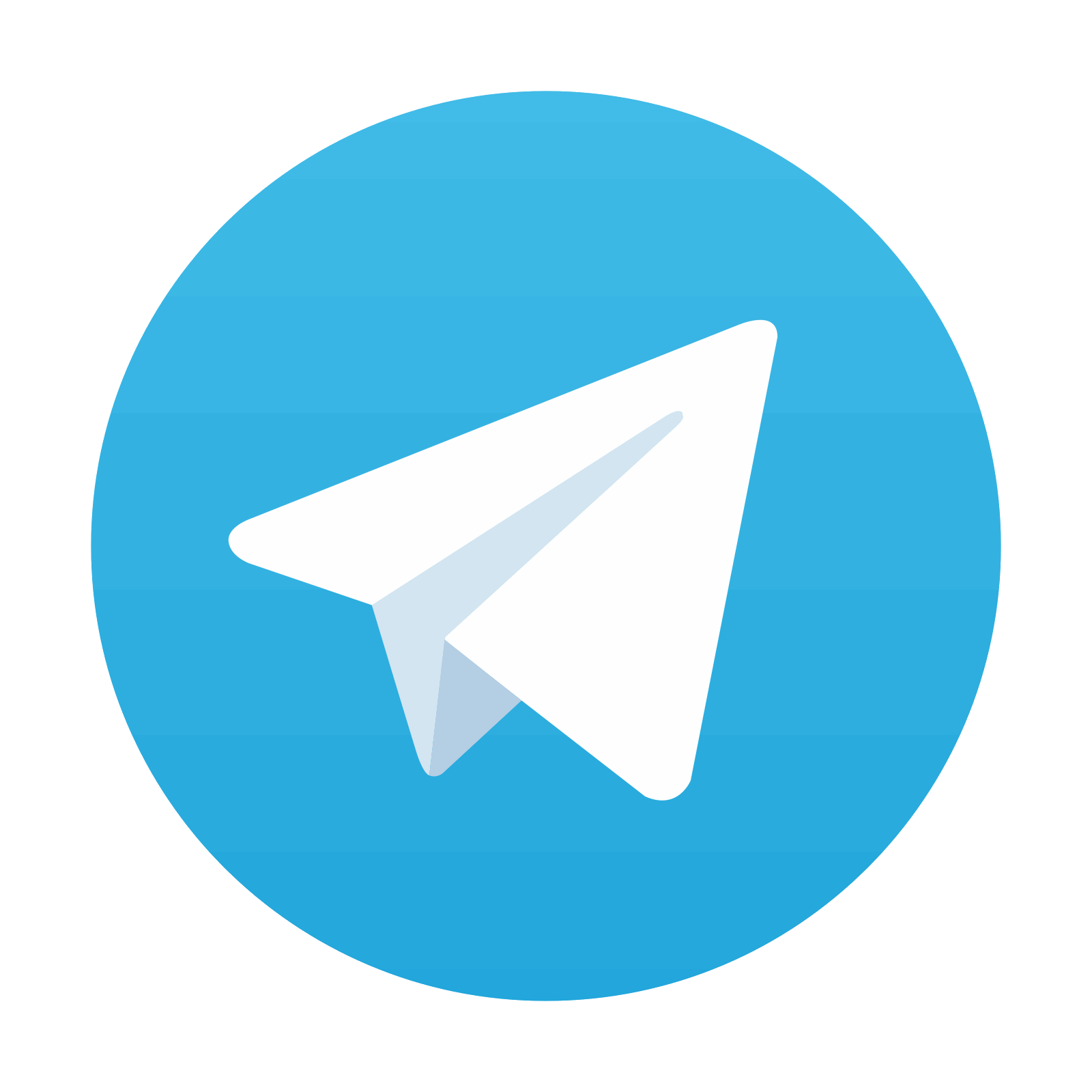
Stay updated, free articles. Join our Telegram channel

Full access? Get Clinical Tree
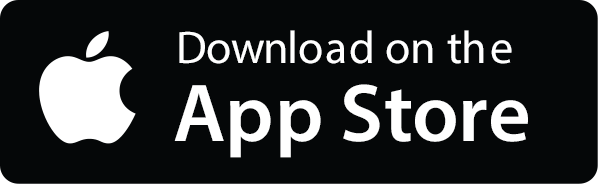
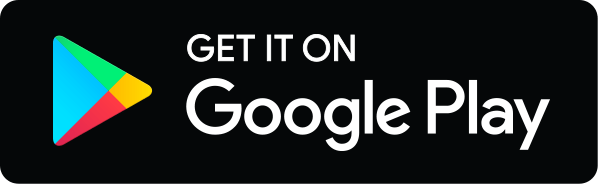