During PD, physiological solution or dialysis fluid is instilled into the peritoneal cavity. Uraemic toxins and solutes move across the membrane by the process of diffusion, from the blood stream into the dialysis fluid, or vice versa, depending on the concentration gradient. The composition of the dialysis fluid is near to that of normal extracellular fluid.
Fluid removal takes place by osmosis. The dialysis fluid is made hypertonic to plasma by the addition of osmotic agent, usually glucose. Other osmotic solutions are discussed later in this chapter.
The membrane is made up of three layers (Figure 9.2a):
Figure 9.2 (a) The three layers of the peritoneal membrane. (b) Diagrammatic representation of a normal mesothelial cell.
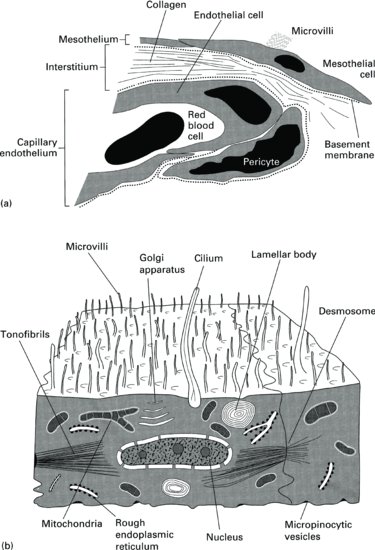
- The mesothelium. Underneath this lies the connective tissue. The luminal side of the mesothelium is covered with numerous microvilli that are believed to increase the surface area of the peritoneum to up to 40 m2 in a healthy individual (Figure 9.2b).
- The peritoneal interstitium. This is composed of fibres and bundles of collagen.
- The capillary endothelium. This forms a complex branching system.
Blood supply
The visceral peritoneum is supplied by the superior mesenteric artery. The parietal peritoneum is supplied by the intercostal, epigastric and lumbar arteries. Venous return from the visceral peritoneum is to the portal circulation whereas that from the parietal peritoneum goes into the caval circulation. This is important because it means that any drugs administered via the peritoneum will be transported to the liver, the normal route.
Lymphatic drainage
Lymphatic drainage from the peritoneal cavity returns excess fluid and proteins into the systemic circulation. Its other function is to remove foreign bodies from the peritoneal cavity. Lymphatic drainage is a one-way system, the flow rate of which may be affected by respiratory rate, intraperitoneal hydrostatic pressure, posture or peritonitis.
Peritoneal membrane transport characteristics
The peritoneal membrane is semipermeable and allows the passage of both water and solutes. During PD three processes are involved in removing fluid and wastes from the blood stream and balancing electrolytes. These are osmosis, diffusion and convection.
Osmosis
This is the movement of water through a semipermeable membrane from a solution of low concentration into a solution with a higher concentration. The solution into which the water moves in PD contains an osmotic agent, usually glucose (other osmotic agents are discussed later in this chapter). The higher the glucose concentration, the greater the osmotic effect will be, so removing more water from the patient’s bloodstream (Figure 9.3).
Figure 9.3 Osmotic ultrafiltration across the peritoneal membrane with a glucose dialysis solution in the peritoneal cavity.
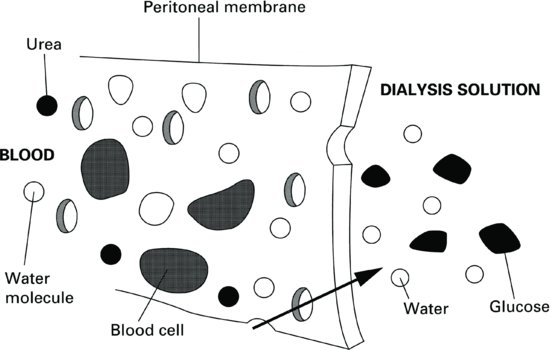
Diffusion
This is solute exchange between two solutions, usually separated by a semipermeable membrane. The solutes will travel in either direction across the membrane until equilibrium is achieved. The direction and speed at which the solutes flow depend on the concentration gradient. Solutes will flow from the stronger solution into the weaker solution (Figure 9.4). Therefore, solutes can pass in either direction across the peritoneal membrane. Other factors that affect diffusion rate are molecular weight and membrane resistance.
Figure 9.4 The peritoneum acts as a semipermeable membrane, allowing small solutes and water to diffuse through, but retaining large particles such as blood cells.
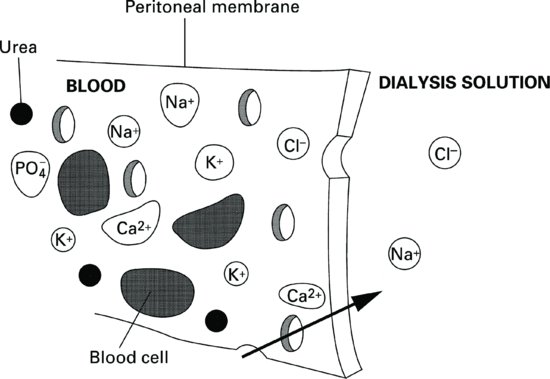
Molecular weight
Diffusion is a spontaneous process whereby solutes move randomly. Lighter, smaller molecules will move quicker than larger, heavier molecules. Urea (which has a molecular weight of 60 Da) diffuses from blood to dialysate more rapidly than creatinine (molecular weight 113 Da) or vitamin B12 (molecular weight 1352 Da). Unlike haemodialysis, where the membrane pore size is controlled, the peritoneal membrane allows transport of large molecules and even proteins. Protein transport into the dialysate is unfortunate as this is an essential nutrient, particularly in dialysis patients who may be catabolic.
Membrane resistance
The permeability of the individual’s membrane is an important factor controlling diffusion of solutes. Measurement of this is discussed later in this section.
A patient’s peritoneal permeability may be changed during illness. Acute episodes of peritonitis appear to greatly increase the membrane permeability to both solutes and water. However, fibrotic thickening of the membrane, may lead to a severe reduction in its permeability. Further reading on the functional structure of the peritoneum can be found in Gotloib (2009).
Convection
Owing to the large amount of osmotic ultrafiltration that takes place during PD, convective flow transports or ‘drags’ water and solutes across the membrane. This occurs at a much faster rate than that which may be accounted for by diffusion alone.
The ability of glucose to exert an effective osmotic pressure depends on its ability to stay in solution in the dialysate. If the peritoneal membrane were perfectly semipermeable (i.e. only permeable to water), the osmotic pressure would be maximised. However, the peritoneum is permeable to solutes as well as water, and therefore allows the glucose through. The osmotic gradient is therefore maximum at the beginning of the exchange. The ultrafiltration will decrease during the dwell time as glucose is absorbed into the blood stream. It is estimated that ultrafiltration is maximised at the beginning of the PD fluid exchange when the concentration gradient is highest. The total dialysate and ultrafiltrate volume continues to decrease after this point due to lymphatic absorption (Krediet et al. 2008).
Measurement of peritoneal permeability
The characteristics, pore size and dimensions of the peritoneal membrane do not come printed on the side of a packet as they do in haemodialysis. It’s therefore important to determine the characteristics of the patient’s peritoneal membrane before an appropriate prescription can be written. A number of methods have been described, including standardised peritoneal assessment (SPA), the Peritoneal Equilibrium Test (PET) including Fast or mini PET, Personal Dialysis Capacity (PDC) test and more recently double mini PETs. The PET is the most commonly used.
It was described in 1987 by Twardowski et al. and determines solute transport rates over time and the ultrafiltration capacity of the membrane. The results of the test can therefore be used to determine the optimum length of time that PD fluid should be left inside the peritoneal cavity to gain maximum fluid and solute removal. It also alerts the practitioner to any changes in membrane function that could affect patient’s dialysis adequacy and outcomes. A review of evaluation methods of peritoneal membrane characteristics has been written (Van Biesen et al. 2010).
Performing a PET
The PET test was traditionally carried out by an experienced PD nurse in a clinic environment, however with the adoption of the ‘fast’ PET where blood samples are taken at 4 hours only (Kazancioglu et al. 2012) this can now be performed at home, allowing for greater flexibility. Prior to performing the test the patient’s peritoneal dialysis catheter function should be checked so that poor drainage due to mechanical problems with the catheter (such as constipation) are not attributed to membrane function. In addition, patients should be normally hydrated, and patients with diabetes should have serum glucose levels within normal limits. This enables the results of the test to be interpreted accurately.
To enable accurate reproduction of the test according to Twardowski’s original work, the following is the original protocol, which is the basis for all tests and should be used if there is any doubt over the reliability of the ‘fast’ or modified PET.
Calculating results
In order to determine the patient’s membrane solute transport characteristics, the level of creatinine that is in the PD fluid at 0, 2 and 4 hours is compared with the level of creatinine in the patients plasma (D/P ratio). This gives a ratio of between 0 and 1. The closer the dialysate-to-plasma ratio is to 1, the faster solutes equilibrate across the membrane. Glucose absorption, from the PD solution into the patient’s serum, is calculated in the same way. The level of glucose in the PD fluid at 0, 2 and 4 hours is compared with the level in the patient’s serum (D/P ratio). The closer the ratio is to 1, the faster glucose crosses the membrane. Patients who equilibrate solutes fast will therefore have good solute removal during PD, however as glucose will also be absorbed quicker, the glucose concentration gradient will not be sustained. Thus, fluid removal will be poor.
The results can be plotted to determine the transporter status of the peritoneum.
Analysis of results
When the patient’s transport characteristics have been identified, it is easy to tailor the treatment to suit the membrane. The patient’s peritoneal membrane is categorised into one of the four membrane classifications. Each membrane classification has specific characteristics that guide the clinician in tailoring the patient’s prescription (see Table 9.1)
Table 9.1 Classification of membrane type
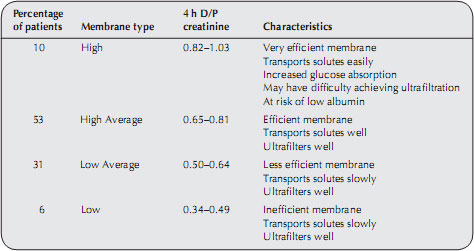
Patients who have high transport membranes should have rapid exchanges of fluid, usually achieved by using APD. Patients who transport glucose and solutes more slowly are better suited to receiving CAPD where the fluid remains in the peritoneal cavity for longer periods. Any prescription modification should be accompanied by an assessment of the patient’s lifestyle to ensure that the treatment is achievable within work and social constraints. By performing this test regularly it is possible to monitor peritoneal membrane function over time and therefore diagnose acute membrane injury, inadequate ultrafiltration and poor solute clearance.
A PET should be performed after 4–8 weeks on dialysis. It has been suggested (Renal Association 2010) that repeat tests should be routinely performed every one to two years or if problems arise such as an apparent loss of ultrafiltration or if there is a change in therapy such as transfer from CAPD to APD. Changes over time in the test results can then be related to clinical performance and treatment regimens may be altered accordingly.
Peritoneal Dialysis Access and Exit Site Care
Despite peritoneal dialysis (PD) catheter insertion being a relatively simple procedure, the implications of getting it wrong can be catastrophic for the patient at a most vulnerable time – when they are new to PD. Not only can poor insertion technique lead to a malfunctioning catheter; it can also be responsible for infections and the failure of PD in about 20% of patients (Flannigan 2005), which can be devastating for a patient who has chosen this therapy. Unsuccessful PD catheter insertion also has cost implications and can adversely affect the management of a renal unit.
There are many facets to successful PD access:
- choosing the right catheter type and length;
- good insertion technique;
- meticulous preoperative and postoperative care.
However, what is fundamental is effective teamwork from the multidisciplinary team, in particular the medical and nursing staff.
Types of catheters
The perfect PD catheter is one that provides reliable and rapid inflow and outflow from the peritoneal cavity without leaks or infections. Catheters should be designed to stay in the pelvic cavity and be easy to insert.
Peritoneal dialysis catheters generally have three portions (Figure 9.5):
- The outer segment, which connects via an adapter to the solution transfer set.
- The intramural segment. This has Dacron cuffs, spaced about 8 cm apart. By creating an inflammatory response, fibrous tissue fixes the catheter into position, thereby minimising leakage of dialysate and preventing bacteria entering the catheter ‘tunnel’ and intra-peritoneal segment
- The intraperitoneal part, which has many small holes up the side, is situated inside the peritoneal cavity for the in and outflow of the solution.
Variations in the design of catheters include the number of cuffs (one versus two), the design of the intramural segment (permanently bent versus straight), and the intraperitoneal portion (straight versus curled). Despite the number of types of catheters available the most widely used are the straight (Figure 9.6) and curled (Figure 9.7)Tenckhoff catheters. They are easily inserted under local or general anaesthetic and choice of catheter will usually depend on local preference.
The Swan-neck (Figure 9.8), and Toronto Western (Figure 9.9) catheters were both developed to aid outflow of the dialysate.
Swan-neck
With two downward-pointing segments, migration of this type of catheter is rare thereby serving to aid outflow. It is also thought to prevent infections at the exit site as this segment of the catheter also points downwards, thus preventing accumulation of sweat and pus.
Toronto Western
This has two discs at the intraperitoneal segment helping to prevent migration. This catheter also has a Dacron flange and bead at the deep cuff, which is sewn in position by a pursestring suture, helping to reduce leaks at the exit site.
Literature shows that there is little difference between risk of infection for the various types of catheters, however one study showed that there was increased catheter survival in the coiled catheter when compared to the straight (36% v 77% p = 0.01 Neilson et al. 1995). If properly placed, dual-cuff Tenckhoff catheters have a lower incidence of infection and a longer lifespan than single cuff catheters (Ash 2003). Overall there seems to be no superior catheter to the standard straight Tenckhoff catheter.
Preinsertion preparation of the patient
A full preoperative assessment of the patient is essential to identify existing or potential hernias. If present these can be repaired during the catheter insertion. The catheter exit site should be determined before the catheter is inserted. The preferred site should first be discussed with the patient to help promote participation in and an understanding of the therapy. The following points should be followed when determining the exit site of the patient’s catheter:
- the site should be determined whilst the patient is in a relaxed sitting or standing position;
- it should be either above or below the belt line, whichever the patient prefers;
- skin folds and abdominal scars should be avoided;
- the catheter exit site should be located where the patient can effectively carry out exit-site care;
- once the exit site has been determined, it should be marked clearly with a permanent skin marker.
The patients lifestyle, work and hobbies should be taken into consideration when placing the catheter
Preoperative care of the patient
On the morning of the operation, the patient should bathe or have a shower. If necessary, abdominal hair should be clipped (Leaper et al. 2008). A major cause of catheter failure, particularly at the start of PD, is constipation and it is therefore recommended that a powerful aperient such as Picolax is given the day before catheter insertion. It is also important that the patient has an empty bladder before the insertion procedure takes place. Other usual preoperative practices should also be followed.
Staphylococcus aureus screening
There is evidence (Piraino et al. 2005) that patients who have nasal carriage of staphylococcus aureus have an increased risk of exit site infection and peritonitis and that the use of Mupirocin cream or ointment at the exit site is recommended to reduce the incidence of infection. It is therefore advisable to screen all patients for Staphylococcus aureus prior to catheter insertion and treat accordingly.
Prophylactic antibiotics
There is evidence that prophylactic antibiotics prevent catheter infections and peritonitis (Galallah et al. 2000). Recently published guidelines recommend that prophylactic antibiotics should be administered at the time of catheter insertion (Piraino et al. 2005).
Psychological preparation of the patient
This is of absolute importance for someone who is new to PD and this is discussed in detail in Chapter 4.
Insertion techniques
Peritoneal dialysis is a life-maintaining therapy. Access to the peritoneal cavity, via the PD catheter, is therefore particularly important in ensuring its success (see Figure 9.10 for the correct position of the catheter in the abdomen). The insertion technique should be treated as a skill acquired by experienced surgeons physicians and specialist nurses, rather like implantation of a pacemaker or similar device. A team approach is essential and nurse involvement is most important. The nurse’s role in insertion of PD catheters starts with the preoperative preparation described above, all of which can be carried out by the nurse. In many centres the PD nurse accompanies the patient to the operating theatre or procedures room to ensure correct procedures are carried out and that the catheter is functioning well before final wound closure is made.
The four most frequently performed methods of catheter insertion include:
- surgical placement by dissection;
- blind placement using Tenckhoff trocar;
- blind placement using guidewire;
- minitrocar placement using peritoneoscopy.
(Wilkie et al. 2008)
An alternative method of catheter insertion is performed by radiologists using a percutaneous modified Seldinger technique under fluoroscopic guidance and has been shown to be a clinically noninferior and cost-effective alternative to surgical laparoscopic insertion (Voss et al. 2012).
There is no evidence (with randomised controlled trials) to support one method of insertion over another; however, the method needs be determined by patient characteristics. For more complicated patients, including those with previous significant abdominal surgery, a technique that involves direct vision is necessary, such as laparoscopic or open insertion (Figueiredo et al. 2010).
Postoperative care of the patient
The goals of postoperative catheter care are to:
- minimise any bacterial colonisation of the exit and tunnel during the early healing period;
- prevent trauma to the exit site and traction on the cuffs by immobilisation of the catheter;
- minimise intra-abdominal pressure to prevent leakage.
Ideally, the exit site should be undisturbed for 7–10 days following insertion of the catheter. The patient may be discharged home during this period. If, during the first 10 days, the dressing becomes soiled, it should be redressed by a nurse. If it merely becomes dislodged, it should simply be replaced with a fresh sterile dressing. During this period, the catheter tubing must be immobilised by securely taping it to the patient’s abdomen. Local unit policies will be in place and should be followed.
Before discharge from hospital, during this resting of the patient’s catheter, clear instructions must be given as to the correct procedures for caring for the catheter at home. It may be appropriate to involve the community district nursing team if no specific community PD nurse is available. In this case, adequate training and support must be provided for the nursing team.
Directly following insertion of the catheter, it may be flushed with 500–1500 ml of PD fluid to check patency. It may then be ‘capped off’ using a small locking cap on the catheter adapter (this adapter is available in titanium metal or plastic) and left covered until PD commences. Ideally, PD should not be started until healing of the exit site and tissue ingrowth into the catheter’s Dacron cuffs have taken place, usually after 10 days. If dialysis is necessary before this time, automated PD would be the preferred method. This is because this treatment will help to minimise the risk of leakage of PD fluid by allowing the patient to be treated using small fill volumes of fluid in a supine position. It is worth noting that patient-healing mechanisms may be altered in those patients who are uraemic or who have diabetes mellitus.
Long-term care of the exit site
As with any wound, care is aimed at keeping the site clear of exudate or debris that could encourage bacterial growth. A method of exit-site care that best fits in with the patient’s lifestyle is most likely to encourage full participation in the treatment, and therefore reduce the risk of complications. A number of studies have been undertaken to attempt to ascertain which particular method of exit-site care is preferred. Various protocols do exist – for example cleansing with soap and water or cleaning with povidone-iodine; however, there is to date no consensus on which method will reduce the incidence of infection (Twardowski and Nichols 2009).
Whichever solution is chosen, the following points should be considered:
- Harsh solutions should be avoided as they have the potential to cause skin damage, which may predispose to bacterial colonisation.
- Different agents may be preferred in different circumstances. In an immunosuppressed patient the normal skin flora may represent an infection risk; in this case an antiseptic solution may be preferred.
- Whichever method is used, it is important to ensure that the exit site is carefully dried to avoid skin maceration, which could predispose the site to bacterial colonisation.
There is also some debate as to whether it is necessary to keep the catheter exit-site covered. The use of no dressing and a simple exit-site care routine for a well-healed exit site would appeal to many; however, most centres do use some kind of cover (Piraino 2008).
Catheter immobilisation
From the moment the catheter is inserted, it should always be securely anchored to the patient’s skin to avoid torque movements at the exit site. This has been shown to reduce the risk of exit-site infection (Piraino et al. 2005) and can be achieved by using tape or a commercially available immobilisation device or tube holder.
Swimming and bathing
Swimming and bathing can be discussed with patients once the exit site is fully healed and ideally free from infection, with the following recommendations.
A waterproof or occlusive dressing should be applied (there are specialist products available) and the exit site should be cleaned and new dressing applied immediately after immersion in water using normal technique.
It is not recommended to immerse the exit site in water without a waterproof dressing, particularly hot tubs, jacuzzis and so forth. Diving should be avoided as this may put tension on the catheter at the exit site (Wild and Ansell 2010).
Indications for catheter removal
A PD catheter is designed to be a permanent access device, and its removal should not be routine. However, catheters may have to be removed under the following conditions:
- if they are no longer needed;
- in recurrent peritonitis without an identifiable cause;
- in peritonitis due to an exit-site and/or tunnel infection;
- with an unusual causative organism of peritonitis, for example fungus, tuberculosis;
- in bowel perforation accompanied by peritonitis;
- with persistent and severe pain due either to the catheter impinging on internal organs or during solution inflow;
- when there is Dacron cuff erosion and infection.
Peritoneal Dialysis Therapy Options
Dialysis should be prescribed according to each individual patient’s clinical and lifestyle needs. The dose of PD can be increased or decreased by adjusting any one of the following parameters:
- dialysis fluid fill volume per exchange;
- number of dialysis fluid exchanges;
- length of dialysis fluid dwell time;
- osmotic strength or type of dialysis fluid.
By using the PET each patient’s membrane characteristics can be determined, therefore allowing optimisation of the therapy. There are two general methods of performing peritoneal dialysis: CAPD and APD.
Continuous ambulatory peritoneal dialysis
Continuous ambulatory peritoneal dialysis (CAPD) (Figure 9.11) is carried out during the day time, manually by patients themselves or sometimes by a carer. Dialysis fluid is infused into the peritoneal cavity and left to dwell for between 3 and 10 h. After this time the dialysate is drained from the cavity, fresh solution is infused and the whole process starts again. Patients usually perform four exchanges of PD fluid each day, fitting them in as appropriately as possible with their normal lifestyle. For example, exchanges may be performed at breakfast, lunch and supper time with the last exchange of the day being carried out at bedtime. Each exchange takes about 20–30 min to complete.
Continuous ambulatory peritoneal dialysis is most suited to those patients whose membranes transport solutes at an average rate (i.e. low, low-average and high-average ‘transporters’) as it provides the opportunity for longer dwell times. This long dwell period is best achieved during the night time whilst the patient sleeps. Those patients whose membranes transport solutes quickly (‘high transporters’) may need to use an icodextrin solution for the longest dwell overnight. This will avoid dialysate fluid absorption which could occur in these patients during long dwell periods.
Automated peritoneal dialysis
Automated peritoneal dialysis (APD) is carried out by a machine, which performs the dialysis fluid exchanges at night whilst the patient sleeps. An APD machine automatically controls the fill volume, dwell time and length of treatment the patient receives. The dose of dialysis can easily be increased during APD as it is easy and convenient to alter the parameters of the treatment. Dialysis fluid fill volumes can be more safely increased due to the reduction in intraabdominal pressure achieved whilst the patient is supine. This not only decreases the risk of problems associated with high intraabdominal pressure such as leaks around the catheter exit site, abdominal hernias and back pain, but it also increases the amount of dialysis the patient can achieve.
The length of the dwell time and the number of exchanges can effectively be altered without disruption to the patient’s lifestyle. This is a major advantage to ‘high transporters’. Performing frequent exchanges is essential for these patients to achieve adequate dialysis but this can be inconvenient if the patient is on CAPD. APD provides an effective and convenient alternative by enabling rapid exchanges of dialysis fluid whilst the patient sleeps. This increases clearances of solutes whilst maintaining maximum ultrafiltration. As with CAPD, the osmotic strength of the fluid can be altered according to each patient’s need.
Automated peritoneal dialysis is particularly suitable for those patients needing to be free during the day-time. Patients who work or who are studying can benefit from this treatment, as the preparation time for the treatment is short and the dialysis takes place whilst they sleep, leaving them free during the day. Automated peritoneal dialysis is also suitable for those patients who rely on a carer to perform their dialysis, for example children, older people or those with disabilities. The carer simply prepares the machine, connects the patient to the machine at bedtime and disconnects them the following morning. Automated peritoneal dialysis is therefore an effective therapy option for those patients who require more dialysis and/or freedom during the day time. However, in order to achieve adequate dialysis, many patients will also need to perform an exchange in the early evening.
Some APD machines have the facility to store information regarding the patient’s therapy on a data card that is fitted inside the machine. This data card also stores the patient’s individual prescription, enabling the prescription to be altered by a healthcare professional, without the need for the patient’s intervention.
There are three different types of APD:
- continuous cycling peritoneal dialysis (CCPD);
- tidal peritoneal dialysis (TPD);
- optimised cycling peritoneal dialysis (OCPD).
Continuous cycling peritoneal dialysis (Figure 9.12)
The ‘continuous’ part of the acronym is derived from the fact that the patient has dialysis fluid in constant contact with the peritoneal membrane. There are typically between five and seven exchanges of fluid with relatively short dwell periods, so maximising the ultrafiltration and clearance capabilities of those patients whose membrane transports solutes quickly. The treatment regime can be programmed to end with a ‘fill’, giving the patient a ‘wet day’ (the fluid would be left inside the peritoneal cavity during the day and drained when the patient next starts treatment on the machine at bedtime). Icodextrin solution, designed for long dwells, should preferably be used in these circumstances.
Rarely, the treatment ends following the ‘drain’, giving the patient a ‘dry day’. This may help to prevent absorption of the PD fluid in ‘high transporters’. It should be noted, though, that utilising a ‘dry day’ will result in severely reduced clearances and possibly underdialysis.
Tidal peritoneal dialysis (Figure 9.13)
Tidal PD is a form of APD where the dialysis fluid fill volume is not fully drained after each cycle. It is typically used for patients who experience pain at the end of each drain phase. Particularly new or acute patients getting used to the sensation of fluid in the peritoneal cavity. By leaving a small “sump” volume of about 10% of the dialysis fill volume in the patients peritoneal cavity at the end of each drain phase, pain is minimised. A full drain takes place at the end of the therapy during the final drain. The sump volume should be minimised as patient comfort allows to ensure the patient receives optimum therapy. It is important to remember to check the minimum drain volume parameters to avoid IIPV (Increased intraperitoneal volumes) and associated risks.
Optimised cycling peritoneal dialysis (OCPD) (Figure 9.14)
This method of APD enables the delivery of more dialysis as the patient’s residual renal function declines. During OCPD the patient performs overnight CCPD or TPD as well as adding a day-time exchange of PD fluid. Some APD machines are designed so that the patient can perform both the night and day exchanges using the same disposable equipment and solution bags. The longer dwell of fluid during the day time will optimise clearance opportunities and the shorter dwells of the night-time dialysis will help to achieve ultrafiltration goals.
Whichever method of PD is chosen for the patient at any particular time, it should provide a balance between clinical and lifestyle needs. Figure 9.15 shows an example of an APD machine.
Figure 9.15 Baxter Healthcare Ltd HomeChoice Pro automated peritoneal dialysis machine (used with kind permission from Baxter Healthcare Ltd).
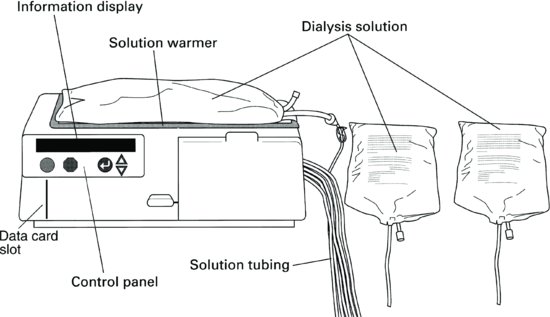
Patient Selection
An important consideration when developing a successful PD programme is good patient selection. Treating the most appropriate patients with PD will avoid the patient being subjected to increased risk of morbidity and mortality. In the early years of PD, selection was mainly based on the patient’s inability to have haemodialysis – for example, those patients with poor or no vascular access, poor biochemical control on haemodialysis with high predialysis serum levels of creatinine, those patients with poorly controlled hypertension and those with excessive fluid gain between dialysis sessions. Positive selection criteria are now preferred as PD has proven to be a successful viable alternative to haemodialysis as a treatment for chronic renal disease.
The National Institute of Health and Clinical Excellence (2011) guidelines recommend that PD should be first line treatment for patients without significant co-morbidity and those with residual renal function. In 2011, Molnar published a paper reporting that PD pre-transplantation had a positive effect on survival post transplant. As there are two different therapy modes within the broad title of PD (i.e. CAPD and APD), the selection criteria for each are seen to be slightly different with many overlaps. Tables 9.2 and 9.3 indicate which patients are more suited to APD and those who are more suited to CAPD.
Table 9.2 Patients who are most suited to continuous ambulatory peritoneal dialysis (CAPD) or automated peritoneal dialysis (APD).
Suited to CAPD | Suited to either CAPD or APD | Suited to APD |
Patients whose membranes transport solutes slowly or at an average speed. | Those who prefer a home-based therapy | Patients who seek day-time freedom to attend work or school |
Patients with complicating cardiovascular disease. | Patients at risk of complications associated with raised intraabdominal pressure | |
Children with small body size. | Patients who require a carer to perform their dialysis | |
Patients with poor vascular access. | Patients with chronic backache | |
Patients with diabetes mellitus. | Patients who require enhanced dialysis, i.e those without residual renal function | |
Patients with severe hypertension. | ||
Patients with anaemia. | ||
Patients who wish to travel. |
Table 9.3 Patients who are unsuitable for continuous ambulatory peritoneal dialysis (CAPD) or automated peritoneal dialysis (APD).
Unsuitable for CAPD | Unsuitable for either CAPD or APD |
Patients with chronic back pain. | Patients with chronic obstructive lung disease. |
Patients at risk of complications associated with raised intraabdominal pressure. | Patients with diverticular disease Patients who are unable to care for themselves and do not have the assistance of a full-time carer. |
Peritoneal Dialysis for those with Diabetes
Diabetic nephropathy is the leading cause of end-stage renal disease worldwide (Atkins and Zimmet 2010). Although death rates of patients with diabetes on haemodialysis and peritoneal dialysis (PD) have decreased substantially, they remain higher than rates in nondiabetics on both modalities. PD offers equal survival compared with haemodialysis for younger patients with diabetes during early years of dialysis. Mehrotra et al. (2011) describes the benefit of preserving vascular access in patients who often have poor vasculature.
Blood glucose control
A patient using only glucose-based solutions will absorb between 100 and 150 g of glucose per day from the dialysis fluid. This can lead to problems, such as hyperinsulinaemia and premature arteriosclerosis. Alternative solutions offer benefits for those with diabetes. Glucose-free solutions (Icodextrin and amino-acid solutions) provide an excellent solution for people with diabetes as the use of these fluids significantly reduces the amount of glucose absorbed by the patient.
There are a number of methods of blood sugar control for patients with diabetes on PD. These include subcutaneous or intraperitoneal insulin, oral agents or dietary control. Any combination of these methods can be used. No single method has been shown to be more suitable than another for all patients as there appear to be no studies that compare the effectiveness of different methods of insulin administration; however, all methods appear to be effective in achieving metabolic control of blood sugar. Poor glycemic control appears to be associated with higher mortality in patients on PD (Duong et al. 2011). It is very important to be aware of the danger of using inappropriate point-of-care glucose meters in patients using icodextrin dialysate (Perera et al. 2011).
Intraperitoneal insulin
There are many benefits to adding insulin via the intraperitoneal (IP) route. Insulin administered by the IP route crosses the peritoneal membrane by passive diffusion and is predominantly delivered to the liver via the portal circulation before reaching the systemic circulation. Following IP administration, the concentration of peripheral-free insulin is lower when compared to the peripheral free insulin concentration achieved following subcutaneous dosing. A proposed advantage of IP insulin administration is that insulin is delivered to the liver without creating hyperinsulinemia, a situation thought to be potentially atherogenic.
Absorption of insulin from the peritoneal cavity is concentration dependent. Insulin administered into an empty peritoneal cavity will be absorbed more rapidly and completely than if the insulin is administered in a large volume of dialysis solution. Direct injection of multiple daily doses of insulin into the peritoneum may be impractical for most patients. Therefore IP insulin is usually administered via the routine dialysis fluid exchange. Conversion from a stable subcutaneous insulin dose to IP insulin dosing at initiation of PD usually requires a 2.5 to 3.5-fold increase in the insulin dose. This increase is needed because of the incomplete absorption during the dialysate dwell period, an increased insulin requirement due to the hypertonic dextrose-containing dialysate, and possible adsorption (binding) of the insulin to the polyvinyl chloride surface of the dialysis bags. Approximately 10 to 20% of the administered dose may bind to the surface of the PD bag and tubing. Regular (shortacting) insulin should be used for IP dosing.
For patients using CAPD, the total daily insulin dose should be divided among the exchanges according to anticipated calorie intake from food and dialysate dextrose. Additional amounts of insulin may need to be added to exchanges containing more hypertonic dextrose concentrations. The exact amount varies between patients but may be assessed at onset by using a sliding scale of insulin with capillary blood glucose monitoring. Dose stabilisation may require several days. Insulin requirements often increase during episodes of peritonitis.
There are a few issues that should be taken into consideration when deciding to use this method of administration: Patients who have dexterity and/or visual problems may experience difficulties injecting insulin into the medication port and the potential exists for contamination of the sterile dialysate solution when adding any medicine via the intraperitoneal route. Patients also require frequent blood glucose monitoring when attempting to regulate IP insulin initially (Farina 2004).
Insulin should be added to the bag of dialysis fluid before it is connected to the patient. In this way, the bag may be discarded if accidentally contaminated or punctured by the needle. Strict aseptic technique must be followed when adding the insulin, which is usually done through the specially designed medication port. All bags should be inverted several times before the fluid is drained into the patient to ensure thorough mixing of the insulin. There is no evidence to suggest that IP insulin administration increases the risk of peritonitis (Williams et al. 2000).
A recent meta-analysis of subcutaneous versus IP insulin for patients with diabetes mellitus on continuous ambulatory peritoneal dialysis found that use of IP insulin provides adequate glycaemic control, which appears superior to that seen following treatment with conventional SC insulin. However the authors reported that research data are limited and further studies are needed to assess for the long-term safety of this approach (Almalki et al. 2012).
Solution Formulation
Peritoneal dialysis solution is presented in sterile plastic bags with a protective overpouch in volumes of 500, 750, 1000, 1500, 2000 and 2500 ml. Bags of 5000 ml are available for use with APD machines. The electrolyte concentration of dialysis fluid is similar to that of normal serum, with lactate acting as a bicarbonate-generating agent to combat metabolic acidosis, which is common amongst patients.Electrolyte composition of the dialysis fluid has been changed several times over the years. A major challenge when treating patients with kidney disease has been effective phosphate control. Consequently, dialysis fluid does not contain any phosphate, and patients are given oral phosphate-binding agents to help eliminate dietary phosphate intake from the body. Calcium is an effective phosphate binder given in the form of calcium carbonate or calcium acetate. These binders can lead to raised serum calcium levels, particularly if the patient is also having vitamin D therapy. This led to the manufacture of a dialysis fluids containing physiological levels of ionised calcium and this solution (a) is compared to the original solution (b) in Table 9.4.
Table 9.4 Comparison of dialysis fluid containing ionised calcium (a) with original solution (b).
a (mmol/l) | b (mmol/l) | |
Sodium (Na) | 132 | 132 |
Chloride (Cl) | 95 | 95 |
Lactate | 40 | 35 |
Magnesium (Mg) | 0.25 | 0.75 |
Calcium (Ca) | 1.25 | 1.75 |
Glucose-based solutions
Up until the early 1990s, the most commonly prescribed and the most widely available osmotic agent used in PD was glucose. Glucose based dialysis fluid comes in three strengths (1.36%, 2.27% and 3.86% monohydrate glucose). The strong (hypertonic) solutions provide the greater osmotic strength. Patients should use 1.36% glucose most often whilst their weight is within 0.5 kg of their ‘dry’ or ‘target’ weight. Hypertonic solutions should be used when the patient has fluid overload. Most patients ultrafiltrate an extra 200–600 ml by using a 2000 mL medium (2.27%) glucose solution bag and an extra 400–1000 ml by using a 2000 ml strong (3.86%) glucose solution bag. Patients should be taught to record their body weight on a daily basis and choose the appropriate solution strengths for that day.
Ultrafiltration by hypertonic solutions is maintained for approximately 8 h in most patients (Figure 9.17). After this time some reabsorption of fluid may occur. In those patients whose membranes transport solutes quickly (high transporters), reabsorption may occur sooner than 8 h, giving rise to the need to use an icodextrin fluid exchange for the longer dwell times (i.e. the overnight dwell in CAPD or the day-time dwell in APD).
Icodextrin peritonal dialysis solution
There are problems associated with the use of glucose as an osmotic agent, such as hyperglycaemia and hyperlipidaemia. Patients who have poor ultrafiltration provide the greatest challenge in this area. Worsening fluid balance results in reduced technique and patient survival. Icodextrin solution is known to improve ultrafiltration in the long dwell during PD (Paniagua et al. 2009).
Icodextrin 7.5% is a formulation of a large molecular-weight glucose polymer, which is a more biocompatible solution as it is approximately iso-osmolar with serum. The use of this larger molecule means that less glucose is available for absorption through the peritoneal membrane. Its long-acting ultrafiltration performance (up to 12 h) is combined with low carbohydrate absorption. Icodextrin reduces the exposure of the peritoneal membrane to glucose for more than 50% of the dialysis time if used for the long dwell and improves fluid balance and BP control compared with 2.27% glucose (Lin et al. 2009).
Paniagua et al. (2009) demonstrated that patients using Icodextrin had significally reduced glucose exposure and this was, in turn, associated with stabilisation of weight in patients with diabetes.
The 2010 Renal Association standards recommend that specialised solutions such as glucose polymers (Icodextrin) are preferable for patients with high or high average solute transport.
Bicarbonate/lactate-based peritoneal dialysis solutions
Bicarbonate/lactate solutions were developed following extensive research to identify the most suitable alternative formulation to the traditional lactate buffered solutions. The commercially available solution comes in a double-chambered bag because some of the compounds of the bicarbonate/lactate solution are not stable during the steam sterilisation process. Glucose needs to be in acidic conditions (low pH) in order to prevent caramelisation. Since the bicarbonate/lactate solution is neutral, the glucose has to be separated from the other compounds. The glucose sits in the upper, smaller chamber, together with calcium and magnesium salts, under acidic conditions. The bicarbonate, lactate and sodium salt sit in the lower, larger chamber. The two solutions are mixed just before infusion (usually whilst the patient is draining the used fluid during CAPD or during the set up of the APD machine).
This solution, with a combination of bicarbonate 25 mmol and lactate 15 mmol as the buffer, is intended for all exchanges. The solution has a physiological buffer system, with a physiological pH of 7.4, and reduced glucose degradation product levels. It allows a significant reduction of inflow pain and/or discomfort in sensitive patients and has a high potential for improved long-term preservation of the peritoneal membrane (Han et al. 2009).
The composition of a bicarbonate /lactate-based solution is:
- glucose monohydrate: 1.36%, 2.27% or 3.86%;
- sodium chloride: 5.38 g/l;
- calcium chloride: 0.184 g/l;
- magnesium chloride hexahydrate: 0.051 g/l;
- sodium bicarbonate: 2.10 g/l;
- sodium lactate: 1.68 g/l.
They have several advantages as a buffer for PD solutions
- physiological pH and bicarbonate concentration;
- reduced pain on infusion;
- improved membrane preservation;
- retrospective studies have suggested that biocompatible PD solutions may reduce the rates of peritonitis, although a long-term follow-up study not detect any clinically significant advantages in terms of technique survival or peritonitis (Srivastava et al. 2011);
- preservation of residual renal function (Johnson et al. 2012).
Physiological pH and bicarbonate concentration
Bicarbonate is the natural buffer of the body. As a result of the metabolism of ingested foods, our body produces hydrogen ions (protons). This acid must be excreted or neutralised in order to maintain the body’s acid–base balance. The kidneys play a key role in maintaining the acid–base balance. Not only do they excrete hydrogen ions, but they also regenerate bicarbonate. In people with renal failure this function is impaired or lost. Therefore it is important to replace the lost bicarbonate to maintain the acid–base balance and prevent the occurrence of acidosis. Using bicarbonate in the PD solution is the most natural way of restoring the bicarbonate level. Alternative buffers, like lactate, need some conversion (metabolism) before they result in bicarbonate.
The biocompatibility of a PD solution has been defined as the ability of a solution formulation to permit adequate long-term dialysis without a clinically significant undesirable host response (Consensus Conference on Biocompatibility 1994). These objectives will best be met by solutions whose composition is identical to the composition of extracellular fluid in the body, in particular to the composition of blood. Bicarbonate and lactate PD solution has been developed as an alternative buffer component.
Reduced pain on infusion
A clinical study on the treatment of pain on infusion (Cho et al. 2012) compared a bicarbonate/lactate solution and another experimental solution based on 38 mmol/l of bicarbonate (without lactate). The experimental solution and the bicarbonate/lactate solution both reduced the infusion pain, but the bicarbonate/lactate mix appeared the most effective.
Improved membrane preservation
In a study comparing lactate and physioneal, physioneal showed significant improvements in cytokines and markers of peritoneal membrane damage such as effluent advanced glycosylation end-products (AGE) and IL-6 (Fusshoeller 2004).
Amino acid based peritoneal dialysis solution
Intraperitoneal amino acid (IPAA) solution contains 15 amino acids. Eight are essential amino acids, two are considered essential to patients with kidney disease and five are nonessential. The electrolyte formulation is shown in Table 9.5. The osmotic agent glucose is replaced by 1.1% amino acids, which have the comparative ultrafiltration capability of 1.36% glucose solution. After a 4–6 h dwell, 65–95% of the amino acids are absorbed, the equivalent to about 18 g (from a 2000 ml bag containing 22 g of amino acids) or 0.3 g/kg/day in a 70 kg patient. This is approximately 25% of the daily protein requirement for a patient on PD.
Table 9.5 The electrolyte formulation of intraperitoneal amino acid solution.
Sodium | 132 mmol/l |
Chloride | 95 mmol/l |
Lactate | 40 mmol/l |
Magnesium | 0.25 mmol/l |
Calcium | 1.25 mmol/l |
Intraperitoneal amino acid is administered in the same way as other PD solutions. It is prescribed for one exchange per day, replacing one of the usual glucose dialysis solution bags or mixed with overnight glucose solutions during APD. If given during the day, it should be given either at a main meal or with a high-calorie snack, as this will ensure that the amino acids are used in the anabolic process to generate protein rather than being expended as an energy source. The use of 1.1% amino acid PD solution, when used instead of one glucose exchange decreases both peritoneal membrane glucose exposure and systemic glucose absorption.
It now seems obvious that patients will benefit from using a combination of all the available solution formulations, including amino acid, polyglucose, bicarbonate/lactate and glucose. Renal Association Guidelines offer advice on using different PD solutions (Woodrow and Davies 2011).
Assessing Peritoneal Dialysis Adequacy
It is well documented that many patients survive well on dialysis and this is due to its ability to remove nitrogenous waste products, correct electrolyte and acid-base imbalance, and remove excess fluid. Patients feel unwell if they are inadequately dialysed; they eat less, become malnourished, and are therefore at increased risk of infection. Inadequate fluid removal causes hypervolemia, which results in hypertension, fluid overload, and cardiac complications.
The goals of PD are to prolong life, reverse the symptoms of uraemia, maintain patients in positive nitrogen balance, have an adequate energy intake and have their maximum level of quality of life in a way that is least disruptive to their lifestyle. A major benefit of a home-based therapy is promoting patients’ control over their own condition and treatment, which can have positive physical and psychological effects (Mathers 2011). This can be summarised by saying that well dialysed patients feel well enough to eat a sufficient diet rich in protein and experience a minimum of complications to their treatment.
There are a number of ways in which dialysis adequacy can be measured and all parameters must be taken into consideration:
- creatinine clearance – a solute-removal test based on body surface area;
- Kt/V – a urea index relating urea clearance to the volume of urea distributed in the body (see below);
- protein nutrition;
- general wellbeing.
Creatinine as a measure of dialysis adequacy
Creatinine is a metabolic product from the breakdown of muscle. Patients who have a larger muscle mass therefore tend to have higher serum creatinine levels. Creatinine, with a molecular weight of 113 Da, will equilibrate more slowly across the peritoneal membrane than urea (molecular weight 60 Da).
A normalised BSA of 1.73 m2 is used to enable an assumption of the creatinine generation rate based on a patient’s size, provided that the patient is infection free and in nitrogen balance.
Urea as a measure of dialysis adequacy
Urea is a metabolic product of the protein we eat. As more protein is eaten, more urea is generated. It therefore follows that when examining a patient’s serum urea levels it is important to take into consideration recent protein intake. A low serum urea in a patient with ERF may simply be due to a low protein intake, rather than adequate dialysis. A high serum urea on the other hand may indicate increased catabolism, a deterioration in residual renal function or inadequate dialysis. Urea is a small molecule (molecular weight 60 Da), which is distributed in body water. It diffuses readily and is therefore easily removed from the blood.
The prescription index Kt/V was developed by Gotch et al. (1983) and Gotch and Sargent (1985) in the National Co-operative Dialysis Study of patients on haemodialysis. Kt/V is an index of urea removal, which is patient-specific as it looks at urea removal achieved over time (K ∞ t) and measurement of urea in the body water (V) for each patient. Therefore: K = clearance; t = time; V = volume of body water in which urea is distributed.
The index does not account for dietary protein intake, protein catabolic rate or urea generation rate. It is just as important to measure residual renal Kt/V as it is to measure dialysis Kt/V. The two should be added together to give a total weekly Kt/V value. Kt/V for both the patient’s dialysis regime and residual renal function can be calculated using the worksheet shown in Figure 9.16.
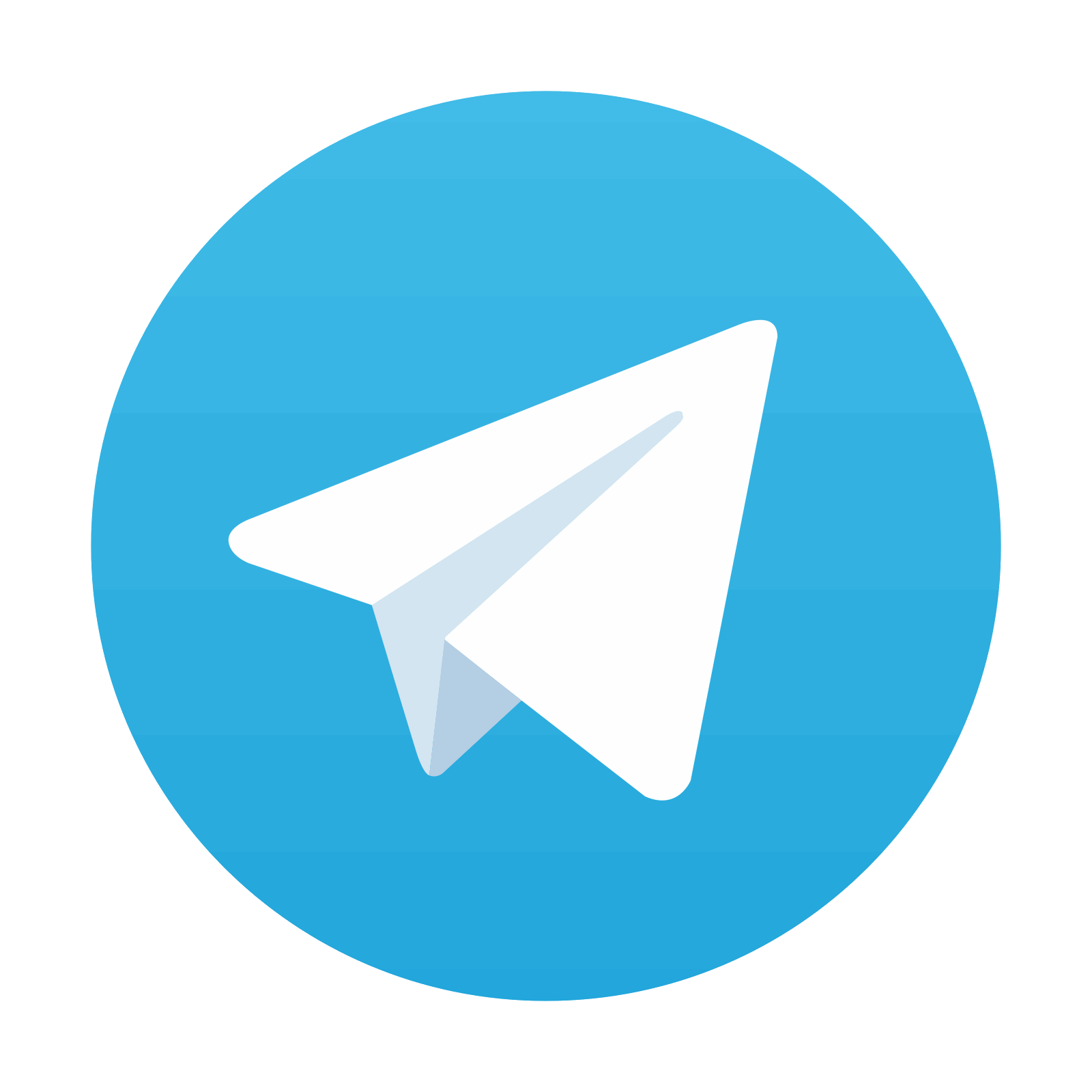
Stay updated, free articles. Join our Telegram channel

Full access? Get Clinical Tree
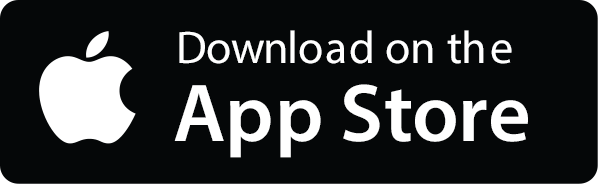
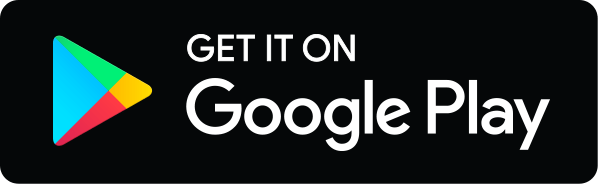