17 Overview of Solid Organ Transplantation
History
History of Solid Organ Transplantation
In the early 1950s steroids were used to suppress rejection, and this resulted in some successful kidney transplants. In 1954, Dr Joseph Murray21 performed the first truly successful kidney transplant between identical twins. Although human liver transplantation was attempted by Starzl in 1963,32 it was not successful until 1967. Also in 1967, the first successful human heart transplant was performed by Christiaan Barnard on a 55-year-old man who survived 18 days.
Evolution of Immunosuppression
In 1994, the next advance in transplantation came with the introduction of mycophenolate mofetil (MMF) and tacrolimus (another calcineurin inhibitor). Tacrolimus has gradually supplanted cyclosporine in many posttransplant protocols, because tacrolimus has been associated with lower rates of steroid-resistant acute rejection than cyclosporin.20 In contrast, MMF quickly and almost universally replaced azathioprine in posttransplant protocols.20,22
A combination of biologic agents (Table 17-1) and immunosuppressants (Table 17-2) are used to prevent and treat rejection. Because immunosuppressive regiments vary from institution to institution, providers should consult their institutional protocols and the institutional pharmacy to verify drugs and dosing regimens used.
Biological Agent | Mechanism of Action | Toxicity |
Antithymocyte globulin | Depletes lymphocytes | Increases risk of infection |
Corticosteroid | Suppresses eicosanoid production: Increases TGF expression | Increases risk of infection and malignancy |
IL-2 antibody | Selectively blocks IL-2 receptors on T helper cells | Increases risk of malignancy |
Muromonab CD3 (OKT3) | Targets CD3 receptor complex on T cells Depletes T lymphocytes | Increases risk of infection |
Alemtuzumab | Targets CD52 on T cells, B cells, and NK cells causing depletion | Increases risk of infection |
Rituximab | Binds CD20 and B cells | Increases risk of infection |
Belatacept | Binds to T cells and prevents their activation, preventing CD28 signaling |
CD: Cluster of differentiation or cluster of designation (numerical nomenclature that identifies white blood cell surface molecules that affect cell signaling and other functions).
Pediatric heart transplantation
Overview
As of 2011, approximately 5437 pediatric heart transplants have been performed in the United States for recipients to the age of 17,24 and many more have been performed worldwide.7 Pediatric patients less than 17 years of age comprise approximately 10% of all heart transplant recipients.24
Heart transplantation is now an acceptable therapy for end-stage heart failure in infants, children, and adolescents (see, also, Chapter 8). One-year patient survival is 83% to 90%, with 5-year survival of 71% to 77%.24 The graft survival is somewhat lower than patient survival (82%-89% 1-year and 68%-75% 5-year graft survival), with some children requiring retransplantation.24 Unfortunately, about 20% of children and about 30% of infants less than 6 months of age who are waiting for a heart transplant die before receiving a heart.
Timing is crucial when listing a patient for a heart transplant, and the risks and benefits of transplantation must be evaluated in light of the limited donor pool. Bridge to transplant therapies such as extracorporeal membrane oxygenation (ECMO) and ventricular assist devices (VADs) are commonly needed for short- or long-term support (see Chapter 7). A recent review has demonstrated improved survival using the Berlin heart.16
Immediate Postoperative Care
If a PA catheter is not in place, evaluation of the balance between oxygen delivery and consumption is accomplished through frequent evaluation of central venous oxygen saturation (SCVO2), typically drawn from a central venous catheter placed in the superior vena cava. The difference between arterial and central venous oxygen saturation is typically 30% if cardiac output is adequate (for further information, see Chapter 6, Clinical Recognition and Management of Shock, Use of Central Venous Oxygen Saturation). If arterial oxygen content is stable, a fall in SCVO2 indicates either a fall in cardiac output or an increase in tissue oxygen consumption. If a central venous catheter is not in place, a venous sample can be drawn simultaneous with an arterial blood gas sample to assess arterial and venous oxygen saturations and evaluate the balance of oxygen delivery and consumption.
Both perioperative and immediate postoperative management focus on optimizing allograft function. Challenges to the function of a newly transplanted heart include: denervation, graft ischemia, and acclimation to the recipient’s hemodynamics.7 The newly transplanted heart has a relatively fixed stroke volume; therefore, an increased heart rate is necessary to augment cardiac output. The heart rate can be increased through administration of inotropes or with atrial pacing.
Pulmonary Hypertension and Right Ventricular Failure
Several measures can be taken to reduce pulmonary vascular resistance and decrease right ventricular afterload, including the administration of milrinone, prostaglandin, prostacyclin, nitroprusside, and inhaled nitric oxide. Milrinone is often used because it has both vasodilatory properties (that can reduce pulmonary and systemic vascular resistance) and inotropic effects.1 Alveolar hypoxia and acidosis should be avoided, because these conditions can exacerbate pulmonary artery constriction, worsening right ventricular failure (for further information, see section, Pulmonary Hypertension in Chapter 8). Some patients with significant elevation in PVR benefit from administration of neuromuscular blockers with sedation (see Chapter 5) and controlled mechanical ventilation for 24 to 48 hours posttransplant.
Treatment of right ventricular failure is largely supportive and includes judicious fluid administration to maximize right ventricular preload, and inotropic support to improve contractility. In rare instances, right heart failure may be so severe that extracorporeal membrane oxygenation (ECMO) is required to support the circulation (see Chapter 7).1 Hemodynamic monitoring and serial echocardiograms will be used to monitor right ventricular function, particularly in response to therapy and during weaning of therapy.
Acute Allograft Dysfunction
Acute allograft dysfunction poses a significant clinical problem that accounts for approximately 30% of early posttransplant deaths. The causes include ischemia-reperfusion injury, problems with preservation, prolonged ischemic time, and unrecognized or underappreciated donor heart dysfunction.7 Allograft dysfunction may be detected even during the operative procedure with intraoperative transesophageal echocardiography (TEE).
A 12-lead electrocardiogram (ECG) is performed shortly after arrival from the operating room and daily after transplantation, because ECG changes may signify early rejection or graft dysfunction. Dobutamine and milrinone infusions are usually adequate to support lesser degrees of allograft dysfunction. On rare occasions, the recipient requires use of a ventricular assist device or ECMO until heart function improves (see Chapter 7).
Arrhythmias
The incidence of tachyarrhythmias after pediatric heart transplantation is about 15%, similar to that reported after adult cardiac transplantation.5 Most tachyarrhythmias resolve after a relatively brief period of medical treatment (see Arrhythmias in Chapter 8), and recurrence is uncommon.19 Although ectopic atrial tachycardia is more common in children than adults, it appears to be well tolerated in the younger age group.5 Atrial flutter tends to be associated with cardiac rejection, and atrial fibrillation is associated with a poor clinical outcome.5
Vasodilatory Hypotension
Vasodilatory hypotension can result from several factors after cardiac transplantation. Loss of vascular tone after heart transplant can be caused by the use of drugs, including angiotensin-converting-enzyme (ACE) inhibitors, amiodarone, and inodilators (e.g., milrinone). Vasodilation can also be caused by a systemic inflammatory response from inflammatory cytokine activation following cardiopulmonary bypass.7 In addition, vasodilation can result from a baroreflex-mediated depletion of arginine vasopressin (AVP). Treatment of vasodilatory hypotension requires the use of vasopressors (catecholamines).
Renal Dysfunction and Hypertension
Urine output, central venous pressure, and serum blood urea nitrogen (BUN) and creatinine are monitored closely after transplantation. Renal dysfunction can result from decreased renal perfusion associated with prolonged aortic cross-clamp time, as well as from thromboemboli or perioperative hypotension. The introduction of calcineurin inhibitors for immunosuppression (see Table 17-2) can suppress renal function further.
Hypertension is common during the early and late postoperative periods, and is often a side effect of medications. Pediatric patients typically require antihypertensive therapy after heart transplantation. Calcium channel antagonists, angiotensin-converting enzyme inhibitors, and diuretics are all acceptable first-line treatments in children with hypertension after cardiac transplantation.27 Individual patient blood pressure profiles and evaluation of drug tolerance and responsiveness are needed to determine optimal drug choice and dosing.
Posttransplant Immunosuppression
The survival of the transplanted heart relies heavily on immunosuppressive therapy. Ideally the goal of immunosuppression is to manipulate the immune response to promote tolerance to the foreign antigens, without rendering the recipient vulnerable to infection.1
Induction immunosuppression is started in the perioperative period. Many institutions begin administration of calcineurin inhibitors, such as cyclosporine or tacrolimus, before the start of the transplant surgery. High-dose corticosteroids are given intraoperatively and continued for 48 hours; they are then either discontinued or tapered to a low-dose maintenance regimen.1
Additional immunosuppressive medications may be given postoperatively in the form of polyclonal antibodies such as antithymocyte globulin (ATG). The maintenance immunosuppressive regimen uses the “triple therapy” approach: a calcineurin inhibitor (cyclosporine or tacrolimus), mycophenolate mofetil (MMF), and corticosteroids (see Tables 17-1 and 17-2).
Rejection
Most pediatric heart transplant recipients experience at least one rejection episode during their lives, with a majority of these occurring during the first 3 months after transplantation.7 Clinical evaluation of rejection is important but can be misleading, especially in the pediatric population, because the inflammatory response associated with an infection can mimic the presentation of rejection.1 Clinical signs of rejection can include vague signs such as fatigue, irritability, gastrointestinal problems (e.g., vomiting, diarrhea, or poor feeding), or the development of specific cardiovascular signs such as heart failure, low cardiac output, or arrhythmias.
Cellular rejection, often referred to as T-cell-mediated rejection, is most common and is characterized by an infiltration of the heart by lymphocytes. The treatment options include optimizing current immunosuppressive therapy plus the administration of high-dose corticosteroids for mild cases of rejection and antithymocyte globulin (ATG) or muromonab CD-3 (OKT3) for severe cases of rejection (see Table 17-1).
Humoral- or antibody-mediated rejection occurs when donor-specific antibodies attack the transplanted allograft. This type of rejection is B-cell mediated and is associated with a higher rate of graft loss, development of transplant vasculopathy, and decreased long-term survival.7 Treatment for humoral rejection includes the use of high-dose corticosteroids, ATG, cyclophosphamide, and plasmapheresis.
Although the most effective way to detect rejection is through an endomyocardial biopsy (see Fig. 8-72), studies are underway to develop less invasive methods for rejection surveillance. Biohumoral markers such as B-type natriuretic peptide (BNP) and vascular endothelial growth factors (VEGF) are currently being studied as markers for rejection. Elevated BNP levels at 1 year or more posttransplant are associated with worse graft survival in pediatric heart transplant patients.28
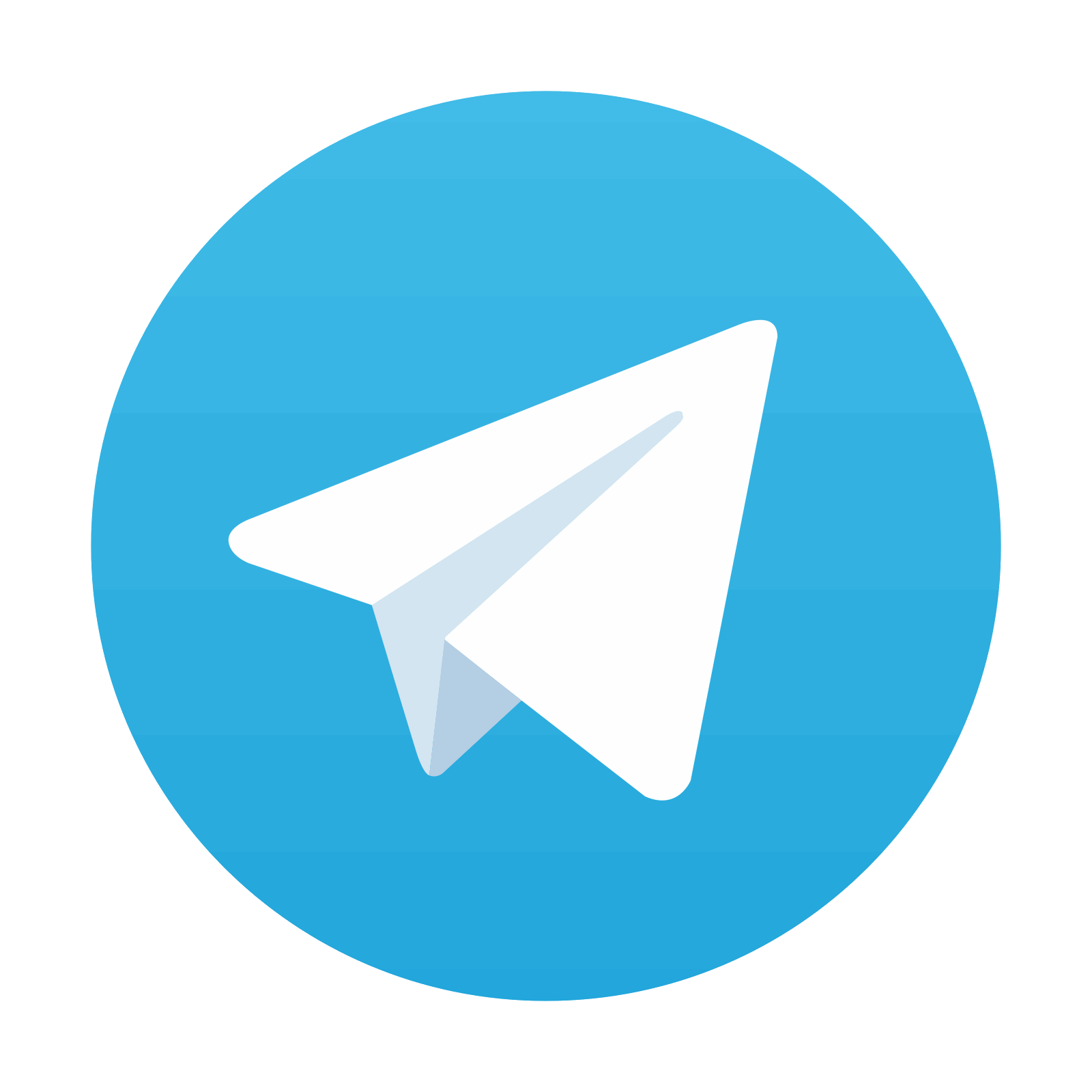
Stay updated, free articles. Join our Telegram channel

Full access? Get Clinical Tree
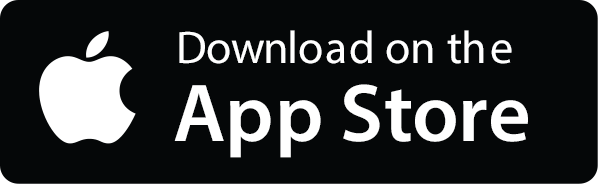
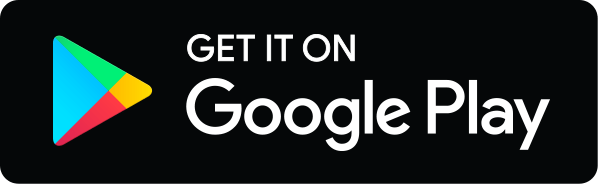