CHAPTER 9 Nursing patients with disorders of the nervous system
Introduction
Many nurses may have contact with patients suffering from neurological disorders. Community nursing staff are increasingly involved in the care of patients recovering at home following acute neurosurgical interventions or with long-term neurological conditions. The National Service Framework for Long-Term (Neurological) Conditions (Department of Health 2005) has highlighted the need for quality care of patients with neurological conditions, regardless of the setting in which they are cared for (Box 9.1 outlines the experiences of living with a lifelong illness).
Anatomy and physiology
The nervous system has two main divisions; the central nervous system (CNS), comprising the brain and spinal cord, and the peripheral nervous system (PNS), consisting of the cranial and spinal nerves. The PNS has two functional parts, the somatic (sensory and motor) and autonomic divisions (pp. 308–311).
Basic tissue structure
Myelin protects and electrically insulates nerve fibres from one another and speeds up nerve impulse transmission. Myelinated nerve impulses are transmitted by saltatory conduction, whereby the impulse jumps from one node of Ranvier to the next (Figure 9.1). Impulses in myelinated nerves are therefore transmitted very much faster than in unmyelinated nerves and require much less energy.
Neurones
Neurones are the structural and functional unit of the nervous system. They are capable of conducting impulses throughout the nervous system and to other excitable tissues, including muscles and glands. The structure of a typical multipolar neurone is shown in Figure 9.1.
The nerve impulse
At rest, the neurone has an unequal distribution of potassium and sodium ions on either side of the plasma membrane, which are necessary to maintain the chemical difference that produces an electrical difference: the inside of the cell is negatively charged in relation to the outside. This has been measured at −70 mV and is termed the resting membrane potential. This state is maintained by exchange of ions between the intracellular and extracellular fluids. A property of all neurones is their ability to produce an impulse when a stimulus is sufficient to initiate certain electrical and chemical changes within the cell membrane. These positive–negative changes occur in rapid succession, spreading to the end of the axon. (See Further reading, e.g. Marieb & Hoehn 2007.)
Generally, the larger the diameter of the axon, the quicker the nerve impulse travels, but the alternative device of saltatory conduction is found in myelinated neurones (Figure 9.2).
The central nervous system
The CNS consists of the brain and spinal cord.
The brain
The cerebrum
The cerebrum forms the bulk of the brain. The outer surface, the cortex (grey matter) consists of neuronal cell bodies. The surface area of the cerebral cortex is increased by folding into a series of grooves (sulci) and ridges (gyri). The deeper grooves are termed fissures and some form landmarks, e.g. the longitudinal fissure which almost splits the brain into left and right hemispheres (Figure 9.3).
The cerebral cortex is responsible for three main functions:
Certain areas of the cerebral cortex have been identified as being responsible for specific functions, and can be mapped (Figure 9.4).
Relative size
The thumbs, fingers, lips, tongue and vocal cords are more sensitive than the trunk, due to the greater number of receptors found in them. The homunculus (Figure 9.5) illustrates how the various parts of the body are represented in the corresponding motor and sensory areas of the cerebral hemispheres, i.e. representation is proportional not to the relative size of the body parts, but to each part’s complexity of movement or the extent of its sensory innervation.
Cerebellum
The cerebellum is located below the posterior part of the cerebrum and is separated from it by a fold of dura mater (p. 305). It consists of two hemispheres separated by a narrow strip called the vermis. The cortex of the cerebellum consists of grey matter, folded to increase its surface area. The interior comprises white matter presented in a branching configuration termed the arbor vitae (tree of life). Links to the rest of the brain and spinal cord allow the cerebellum to receive sensory information and thereby to maintain equilibrium and modify voluntary movement, making it smooth and coordinated.
Pituitary gland
The pituitary gland is situated at the base of the brain in a depression or fossa in the sphenoid bone called the ‘sella turcica’. It is attached to the brain via a stalk which is continuous with the hypothalamus, and communication is by means of nerve fibres and blood vessels (see Ch. 5, Part 1).
Diencephalon
Three bilaterally symmetrical structures comprise the diencephalon:
The hypothalamus
is situated below the thalamus and forms the walls and floor of the third ventricle. It controls the output of the hormones from the pituitary gland. Other functions include the regulation of hunger, thirst and body temperature (see Chs 20, 21, 22).
Brain stem
The medulla
Table 9.1 Outline of the cranial nerves
Cranial Nerve Name And Number | Type | Functions |
---|---|---|
Olfactory (I) | Sensory | Smell (olfaction) |
Optic (II) | Sensory | Vision |
Oculomotor (III) | Motor Sensory | Controls four of the extrinsic (external) muscles that move the eyeball, and the muscle that raises the upper eyelid Some fibres control the iris muscle that constricts the pupil, and the ciliary muscle, which changes lens shape Proprioception |
Trochlear (IV) | Motor Sensory | Controls the external muscle that moves the eyeball down and outwards Proprioception |
Trigeminal (V) (three branches: ophthalmic, maxillary, mandibular) | Motor Sensory | Motor to the muscles of chewing (mastication) Sensory to the face, mouth, teeth and the nose |
Abducens (VI) | Motor Sensory | Controls the extrinsic (external) muscle that moves the eyeball outwards Proprioception |
Facial (VII) | Motor Sensory | Controls the facial, scalp and some neck muscles – facial expression. Autonomic fibres to the lacrimal (tear), nasal and some salivary glands – lacrimation and salivation Also controls the tiny stapedius muscle in the middle ear Taste |
Vestibulocochlear (VIII) | Sensory | Hearing (audition) and balance |
Glossopharyngeal (IX) | Motor Sensory | Controls the pharyngeal muscles involved in swallowing. Autonomic fibres to some salivary glands – salivation Taste Carotid sinus – regulation of blood pressure Proprioception |
Vagus (X) | Motor Sensory | Supplies external ear, heart, larynx, trachea, bronchi, lungs, pharynx, oesophagus, stomach, small intestine and proximal part of large intestine, the liver, gallbladder and pancreas – swallowing, digestive secretions and movement, etc. Taste and other sensory inputs from structures innervated |
Accessory (XI) | Motor Sensory | Controls muscles of the neck and shoulders – head movement, shoulder shrugging. The pharynx, soft palate and larynx – swallowing Proprioception |
Hypoglossal (XII) | Motor Sensory | Tongue movements during speech and swallowing Proprioception |
(adapted from Bowie & Woodward 2003)
The pons
The pons is a bridge between the medulla and the midbrain. It comprises fibres that connect with the cerebellum and fibres that link between the spinal cord and the brain. It contains the nuclei of cranial nerves V to VIII inclusive (see Table 9.1, p. 309). Other important nuclei also exert an influence on respiration.
The meninges
Dura mater
is a double layer of dense fibrous tissue. It is separated from the arachnoid mater by the subdural space. The outer layer adheres closely to the underside of the cranial bones, whilst the inner, meningeal layer is much thinner. The spinal dura mater has only one layer, which corresponds to the meningeal layer of the cranium. The two layers of the dura mater separate at several locations and these spaces contain the venous sinuses, e.g. the falx cerebri and the tentorium cerebelli. The former forms an incomplete division dipping down between the two cerebral hemispheres (Figure 9.6). The tentorium cerebelli forms a division between the occipital lobes of the cerebrum and the cerebellum.
The ventricular system
The ventricular system of the brain comprises four fluid-filled irregular cavities (ventricles) interconnected by narrow pathways (Figure 9.7) and connected with the central canal of the spinal cord and the subarachnoid space. There are two lateral ventricles, one in each cerebral hemisphere, one ventricle (the third) located in the diencephalic region and the fourth located in the medulla.
Blood supply and drainage
The greater part of the brain is supplied with blood by the arteries branching from the circulus arteriosus (circle of Willis), a ring of blood vessels located at the base of the brain (Figure 9.8).
The spinal cord
The spinal cord is an oval cylinder that lies within the spinal cavity of the vertebral column. In adults, it is approximately 45 cm long and extends from the medulla to the first or second lumbar vertebrae. Beyond this, the spinal nerves from the lumbar and sacral segments of the cord form the cauda equina, or ‘horse’s tail’. The lower part of the cord is attached to the coccyx by the filum terminale and is tapered in shape (Figure 9.9).
The cord is segmented into five parts or regions, each corresponding to the specific vertebrae:
Two enlargements of the spinal cord are seen: (i) from C4 to T1 containing the nerve supply for the upper limbs; and (ii) from L2 to S3 which supplies innervation to the lower limbs. Paired spinal nerves, part of the PNS, are attached by two short roots to the cord (see pp. 308, 309).
The structure of the spinal cord is illustrated in cross-section in Figure 9.10.
The spinal pathways are described as:
Sensory pathways
Spinothalamic pathways
are the lateral and anterior spinothalamic tracts. The first order neurone in both pathways connects the receptor with the spinal cord where it synapses with the second order neurone in the posterior grey horn. The pathway crosses over to the opposite side of the cord and ascends in either the lateral or anterior spinothalamic tract to the thalamus. The second order neurone synapses with the third order neurone, which then continues, terminating in the sensory cortex. Between them the spinothalamic tracts are responsible for conveying information about pain (see Ch. 19) and temperature, light touch and pressure.
Motor pathways
The peripheral nervous system
The PNS has two functional parts:
The cranial nerves
The 12 pairs of cranial nerves pass from their origin, principally within the brain stem, out via small openings in the skull to innervate structures around the head and neck, and beyond (Table 9.1). Cranial nerves were formerly described as either motor or sensory, or mixed nerves; however, most motor nerves are now considered to be mixed, but with a dominance of motor or sensory fibres. (See Further reading, e.g. Tortora & Derrickson 2009.)
Spinal nerves
Each spinal nerve has an anterior (motor nerve fibres) and a posterior root (sensory) (see Figure 9.10). The posterior root can be distinguished by its root ganglion, a cluster of nerve cell bodies.
Shortly after leaving the intervertebral foramina, both roots join together to form a mixed nerve. From here the spinal nerves continue to form a complex network all over the body, carrying motor signals to effectors such as the skeletal muscles and conveying sensory information such as touch to the CNS for interpretation. (See Further reading, e.g. Tortora & Derrickson 2009.)
The autonomic nervous system
The ANS comprises the nerves carrying motor impulses to the internal organs; thus it is exclusively peripheral and motor. Its activity is influenced by many factors, including sensory information from the internal organs, numerous peptides and hormones, and signals from higher control centres such as the hypothalamus. It is described as having two divisions, the sympathetic and parasympathetic, each imposing different effects (Figures 9.11, 9.12). (See Further reading, e.g. Tortora & Derrickson 2009.)
Neurological investigations
Patients may undergo a range of neurological investigations depending on their condition. Pre-procedure preparation and post-procedure care is identified in Table 9.2. The specific investigations performed for each condition are detailed throughout the chapter.
Head injury and raised intracranial pressure
In 2006/2007 almost 156 000 people were admitted to hospital in England as a result of head injury, the majority of whom were young males. These figures have risen significantly since the implementation of the NICE head injury guidelines in 2003, which were updated in 2007 (Goodacre 2008). The majority of head injuries are mild (Glasgow coma scale [GCS] 13–15) but moderate (GCS 9–12) or severe injuries (GCS 3–8) are more likely to result in morbidity and mortality (see Ch. 28).
Falls (24–43%) and assaults (30–50%) are the most common causes of mild head injury in the UK, followed by road accidents (25%), although these account for a far greater proportion of moderate to severe head injuries. Alcohol may be implicated in up to 65% of all adult head injuries (NICE 2007a).
Pathophysiology
Three intracranial components contribute to maintaining ICP:
The causes and presenting symptoms of raised ICP
A raised ICP can be due to intracerebral or extracerebral causes (Box 9.2).
A relationship exists between the volume of a lesion inside the head and ICP, represented by the pressure–volume curve (Figure 9.13).
During the initial rise in ICP, compensatory mechanisms come into play. The cerebral ventricular system can reduce the volume of CSF by displacing it into a distensible spinal dural sac. A reduction in cerebral blood volume also occurs as a result of autoregulation, the ability of blood vessels to constrict according to local conditions. This is represented by the flattened part of the curve. However, this is only a temporary measure and, as the volume of the expanding lesion increases, compensation is overcome and the steep part of the curve is entered. Then for every small increase in volume, the corresponding rise in ICP is dramatic. This process has four identifiable stages (Box 9.3).
Box 9.3 Information
Stages of volume–pressure relationship in raised intracranial pressure
Other factors which have an influence on this complex process include cerebral blood flow and cerebral oedema. (See Further reading, e.g. Woodward & Mestecky 2011.)
Herniation
The skull has two compartments:
Trans-tentorial herniation
Lateral trans-tentorial herniation occurs in the presence of an expanding lesion located close to the temporal lobe. The medial part of the temporal lobe (the uncus) is forced downwards and can subsequently develop into a central herniation (Figure 9.14).
Medical/surgical management
Common presenting symptoms
Investigations
In the head-injured patient, skull X-ray may reveal a fracture and computed tomography (CT) or magnetic resonance imaging (MRI) may demonstrate cerebral contusions or lacerations and/or an intracranial haematoma (Hickey 2002) (Box 9.4).
ICP monitoring
One of the most important diagnostic measures is the monitoring of ICP. This is an invasive technique involving direct measurement of ICP. A typical system comprises a fibreoptic transducer-tipped catheter, which can be placed in the lateral ventricle, subdural or extradural space. The level of ICP is then transmitted to a digital data display as a waveform. A pulsatile waveform will be demonstrated along with a pressure level indicating if the patient’s ICP is within normal limits (≤15 mmHg) (Figure 9.15).
Treatment
In the past, the treatment of head injury focused primarily on the interventions required to reduce ICP. However, greater emphasis is now placed on maintaining cerebral perfusion pressure (CPP) which is the pressure needed to perfuse the brain with blood, at more than 70 mmHg (Box 9.5). Those patients with a high ICP, low blood pressure and low CPP make a poorer recovery. The means by which this is achieved are varied according to circumstances.
Controlled ventilation
In the past it was recommended that the reduction of PaCO2 would reduce ICP. However, it also causes vasoconstriction, thus reducing cerebral blood volume. The resultant reduction in cerebral blood flow may itself cause ischaemic brain damage (Lindsay & Bone 2004) so patients are ventilated to keep PaCO2 within normal limits. Maintaining the blood pressure and CPP appear to be as important, if not more important, than lowering ICP.
Sedatives
If ICP fails to respond to standard measures then sedation, under carefully controlled conditions, may help by reducing cerebral metabolism and thus offering a degree of protection for the brain (Lindsay & Bone 2004).
Surgical intervention
Nursing management and health promotion: head injury
Head injury is preventable. This is an area in which nurses should exercise their health promotion skills, e.g. by emphasising the dangers associated with head injury and its detrimental effects when communicating with patients who have experience of minor head injury with good recovery, and with their families. This may include consideration of driver behaviour or unsafe work practices. The use of seatbelts for the driver and all car passengers has led to a reduction in head injuries, as has the use of protective headgear for motorcyclists and horse riders (Headway 2004). Because a common contributing factor in head injury is alcohol intake, where appropriate, the patient can be encouraged to consider personal lifestyle and the consumption of alcohol.
Immediate priorities
The immediate nursing aim is to prevent further damaging rises in ICP, and the first priority is to identify any alteration in respiratory function due to an inability to maintain an airway (Box 9.6). The nurse should be aware of the presence of other injuries, e.g. if the patient has been in a road traffic accident (see Chs 18, 27 for detail of assessment and interventions).
Box 9.6 Information
Respiratory care priorities in a patient with raised intracranial pressure
Assessment of neurological status
This is performed in order to:
Any deterioration in neurological status may be an early indication that ICP is rising further, thus increasing the likelihood of herniation (Mestecky 2007). Neurological assessment is important, as this may be the only indication that the patient’s condition is deteriorating and a standardised method of monitoring neurological status enhances this process. The gold standard method is the Glasgow Coma Scale (NICE 2007a) (Ch. 28).
The nurse, as the health care professional who normally spends most time with the patient, should learn to observe changes in the patient’s behaviour which may herald an impending change in neurological status, e.g. the patient who does not answer questions so readily or who is becoming agitated and restless (Box 9.7).
The expert neurosurgical nurse will be alert to the early warning signs that must be reported so that treatment can be carried out as soon as possible (p. 314).
Surgery
Pre-operative care
Urgent surgery (e.g. craniotomy) may be indicated on admission or in response to subsequent deterioration. The nurse may need to prepare the patient in a very short time and also provide adequate explanation and reassurance (see Ch. 26).
Postoperative care
The overall priorities for patients following neurosurgery are:
Table 9.3 outlines the main complications of neurosurgery.
Table 9.3 Complications of neurosurgery
Complications | Cause | Interventions |
---|---|---|
Altered conscious level | Increased ICP due to cerebral haemorrhage/oedema | Frequent assessment of neurological status |
Onset of seizures | Cerebral irritation | Observation of seizures. Appropriate interventions if they occur (see section on epilepsy) |
Limb weakness | Increased ICP due to cerebral haemorrhage/oedema | Frequent assessment of limb movements |
Speech problems | Increased ICP due to cerebral haemorrhage/oedema | Frequent assessment of verbal responses |
Respiratory problems | Increased ICP due to cerebral haemorrhage/oedema | Frequent assessment of respiratory status |
Loss of swallowing reflex | Increased ICP due to cerebral haemorrhage/oedema | Assessment of swallowing and keep nil by mouth |
Loss of corneal reflex | Increased ICP due to cerebral haemorrhage/oedema | Eye care. Keep eyes closed to protect cornea |
Periorbital oedema | Direct result of surgery | Observe for swollen/bruised periorbital tissues |
Subsequent considerations
All of the nursing interventions identified in Chapter 26 will apply. Only those interventions specific to the patient who is unconscious due to raised ICP are included here. The main aim of care remains that of preventing further rises in ICP.
The patient care outlined in the following sections uses the Roper et al (1980) framework of the activities of living model.
Communicating
Unpleasant stimuli are known to increase ICP, so the nurse should take a calm, reassuring approach. Relatives should be encouraged to talk to the patient and, although they may feel rather foolish at first, they will be encouraged if they see the nurse doing this. They should, however, be warned to avoid discussing potentially upsetting topics as the unconscious patient may still be able to hear (Hickey 2002) and this may cause their ICP to rise.
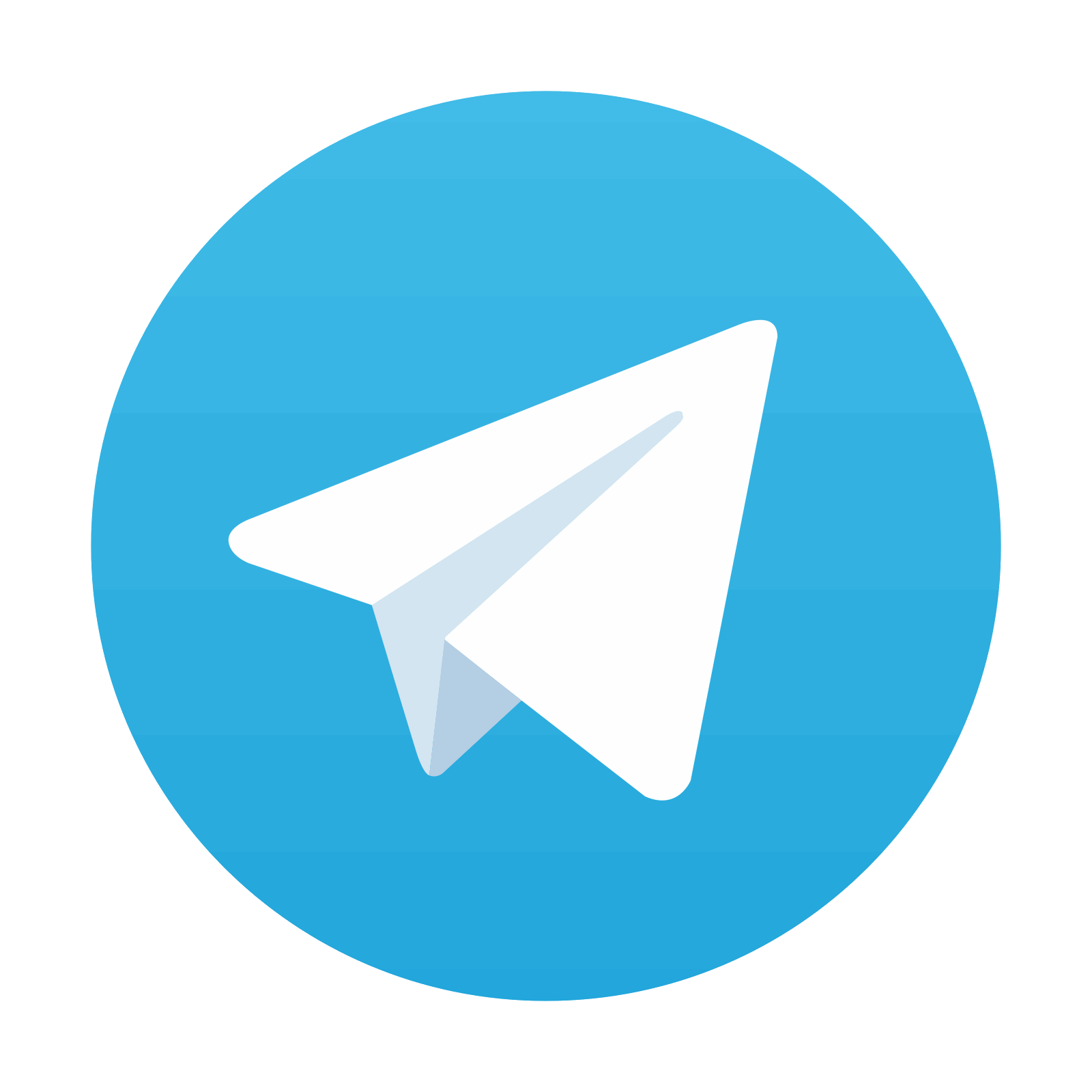
Stay updated, free articles. Join our Telegram channel

Full access? Get Clinical Tree
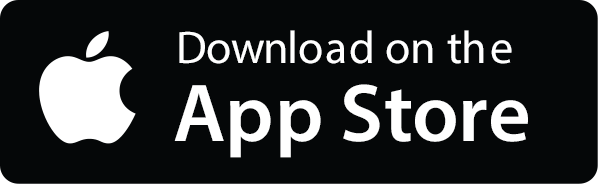
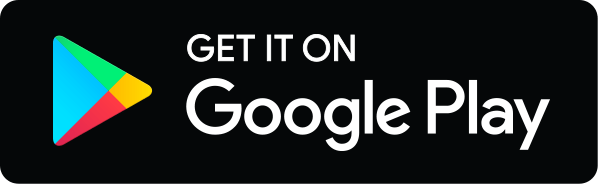