A thickening of the ectoderm forms the neural plate.
Complete closure of the neural tube takes about 4–6 days. During the early part of the neurulation stage the neural tube is fused except for a small posterior neuropore and a larger anterior neuropore. The anterior neuropore will eventually subdivide into three vesicles representing the forebrain, midbrain and hindbrain. The anterior neuropore closes by day 24. The posterior neuropore closes by day 26.
Ectodermal cells from the margins of the neural tube detach to form dorsal clusters of cells called neural crest cells. These migrate peripherally to become sensory ganglia cranial and spinal nerves, etc.
During the three-vesicle stage, representing the forebrain (prosencephalon) midbrain (mesencephalon) and hindbrain (rhombencephalon), the three-part brain is C-shaped.
By week 5 the forebrain gives rise to the paired telencephalic vesicles which will eventually become the cerebral hemispheres and the diencephalon from which develop the optic vesicles. The midbrain remains tubular and undivided. The rhombencephalon (hindbrain) subdivides into the metencephalon and a more caudal myelencephalon.
By week 6, the cellular growth in a restricted area has resulted in the formation of the cephalic flexure, the pontine flexure and the cervical flexure. The metencephalon will form the pons and the myelencephalon will eventually form the medulla oblongata
By weeks 5 and 6 all the cranial nerves (except the optic and the olfactory) are recognisable.
The lateral walls of the spinal cord thicken leaving a longitudinal groove which extends the full length of the spinal cord and into the midbrain. The divided grey matter forms dorsal and ventral plates. These plates signal the location of sensory and motor neurones and will eventually result in the sophisticated network of afferent and efferent neurones which will receive, transmit and respond to stimuli.
The ventricular system is established early from the division of the telencephalon.
The ventricles are well-defined, C-shaped structures. A rich capillary network develops on the roof of the midbrain and hindbrain which will become the choroid plexus and will secrete the cerebral spinal fluid which will bathe the CNS.
Rapid growth in restricted space forces the developing cerebral hemispheres to shroud and cover the midbrain. Initially, the cerebral cortex is smooth.
Neurones start to myelinate. After 18 weeks neurones no longer divide. Surface area landmarks of the brain are increasingly identifiable. Neurones for nociception located in dorsal root ganglion.
Rapid growth of cerebral hemispheres causes convolution of the cortex and form sulci and gyri increasing the surface area.
Rapid increase in neurone cytoplasm gives rise to increase in cell size.
Rapid myelination occurs and will continue into the postnatal period.
The Biology Behind the Nervous System
The nervous system is responsible for an individual’s ability to react to their environment as well as respond to internal triggers. It is a network of complex structures which can receive, emit and transmit electrochemical signals between tissues, organs and systems. The nervous system functions as a unified whole but for convenience is divided into the central nervous system (CNS) and peripheral nervous system (PNS).
The CNS comprises the brain and the spinal cord encased and protected by the skull and spine. The PNS consists of nerve tissue outside the brain and spinal cord. These are nerve bodies/ganglia not entirely encased in the skull and spine and include the 12 pairs of cranial nerves and the 31 pairs of spinal nerves. Some of the cranial nerves are highly specialised and critical to the function of the senses of taste, sight, hearing and smell. The PNS is differentiated into afferent and efferent pathways.
Neural transduction and transmission is complex, but put simply means that ascending nerves carry information impulses to the brain and the descending nerves carry information and impulses from the brain to muscle tissue and glands and so effect the nervous system’s response. Affecters and effecters are specialist neurones and the reader is advised to refer to an anatomy and physiology text such as those listed in the reference section to further augment their understanding.
The nervous system is divided into branches representative of their function, each complementary and working synergistically with the CNS and PNS. The autonomic nervous system is also subdivided into sections; the nerves of the sympathetic and parasympathetic have a primary role in regulating the individual’s internal homeostasis.
The autonomic nervous system exerts control mainly by involuntary action, which in health does not impact on the conscious awareness of the individual.
Components of the Nervous System
The nervous system comprises two basic types of cell: the neurones (Figure 13.1) and the supporting cells such as the neuroglial cells. Neurones vary in size from micrometres to about a 1 metre (i.e. the length of the spine in a young adult) and their shape and complexity differ with function and location. Generally speaking, the neurones do not have direct access to good capillary networks and receive nutrients such as glucose more indirectly. Neurone axons may be covered in a fatty sheath which acts as an insulator. Gaps in this covering allow ions to flow at these intermittent junctions rather than along its full length. This is thought to increase velocity.
Figure 13.1 Structure of a neurone.
From Tortora, G. J. and Derrickson, B. H. (2009) Principles of Anatomy and Physiology, 12th edn. Reproduced with permission from John Wiley and Sons, Inc.
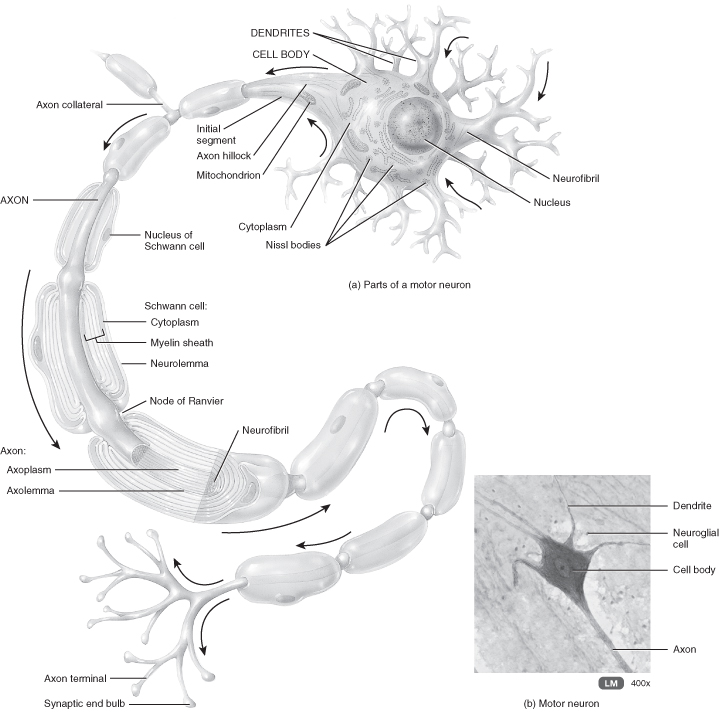
The covering of the axons take place according to a developmental timetable and may be incomplete at birth. The interpretation of this knowledge led to some postulating that infants could not feel pain as their nervous system was immature. It is now accepted that the absence of a myelin sheath does not impede the transmission of stimuli, which will then be perceived and experienced as pain. Foetuses and preterm infants from 24 weeks of gestation have a fully functional nociceptor system (Lee et al. 2005; Wolf 1999).
Neurones are categorised according to the number of branches they have. Unipolar have one process, bipolar two and multipolar several. Generally, sensory neurones transmitting information to the CNS are unipolar and motor neurones transmitting information away from the CNS are multipolar.
Developmental Aspects of the Central Nervous System
Neurones in the CNS do not regenerate. More neurones than are required are initially formed and some continue to divide following birth, with clear implications for early oxygenation and nutrition. If these neurones are not used and become part of a functional system they will die and this has possible implications for neonatal and infant experiences. Axons have limited capacity to repair, but successful function following injury depends on the type of injury and location of lesion. The formation of scar tissue, which is an end product of repair, can impede transmission. The olfactory neurones in the nose are unique in that they continue to divide and are replaced throughout life.
How Nervous Tissue Works
Neurones are not passive; they generate and respond to electrochemical impulses by selectively changing the electrical charge over their membranes. All body cells are polarised, with the inside of the cell more negatively charged than the outside. This difference is due to the composition of the intracellular fluid compared to the composition of the extracellular fluid. There is more potassium inside the cells than outside and more sodium outside the cells; this difference is maintained by active transport on the surface of the cell membrane. Understanding this at its most basic level means that when this equilibrium is disturbed above a threshold the neurone depolarises and the signal transmitted along the axon. Most sensory nerve fibres are unimodal in that they respond to one type of stimulus (e.g. sound waves or light intensity). The afferent nerve fibres of the PNS are different in several respects; they are polymodal and have the ability to respond to mechanical, chemical or thermal reception stimuli. Once this information is transported to the CNS at the level of the spinal cord it means a variety of stimuli can be responded to and there is some ability to discern between degrees of intensity as they have complex multi-transduction channels which can distinguish between different forms of stimulation and between intensities (McCance and Huether 2006). The spinal cord is a long, thin, tubular bundle of nerve tissue and support cells which extends from the medulla oblongata to the lumbar vertebrae. It is the pathway for information connecting the brain to the peripheral nervous system. In cross-section the cord consists of white matter tracts containing sensory and motor neurons and a grey, butterfly-shaped central region made up of nerve cell bodies. The spinal cord is bathed in cerebrospinal fluid.
Δ (delta) and C fibres are regarded as first-order neurones for the detection of acute pain. The transmission of stimuli from these fibres can be transmitted up the spinothalamic tract for interpretation by the third- and higher-order neurones in the cerebral cortex, reticular and limbic areas of the brain, a little like a hierarchy of people passing on important messages to the management team. Understanding the essentials of this puts into perspective the value of the gate theory of pain first proposed by Melzack and Wall (1965).
Once neurones have fired there is a period of readjustment, called the refractory period, during which the neurone cannot fire again. In some cases (e.g. following an inflammatory response) cells can be regarded as hypopolarised and be more excitable than normal, as it takes less to trigger a signal. This hyperalgesia state has direct implications for the transmissions of signals which go on to be perceived as pain and for the management of it, as this state can prove to be very resistant to treatment.
Neurones do not physically connect, although they are in close proximity. The gaps between them are called synapses, with impulses being transmitted across the gaps by electrochemical messengers (Figure 13.2). Transmission occurs by chemical neurotransmitters, which are synthesised and stored by the neurones and released on instruction into the synaptic cleft. These chemicals pass across the synapse to reach a postsynaptic membrane and, depending on the level of excitation, have the potential to transmit the signal onwards.
Figure 13.2 A synapse in action.
From Tortora, G.J. and Derrickson, B.H. (2009) Principles of Anatomy and Physiology, 12th edn. Reproduced with permission from John Wiley and Sons, Inc.
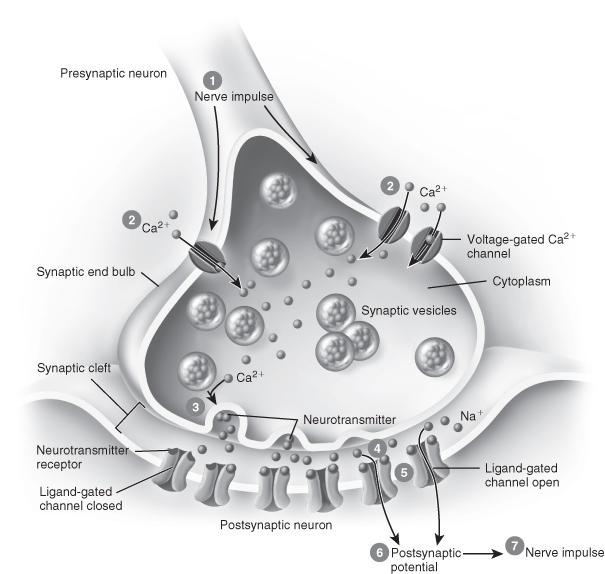
Neurotransmitters
Substances which function as neurotransmitters continue to be identified. Some are chains of amino acids, some are recognised as hormones, and it is apparent that some have several roles, which vary according to location. For example, norepinephrine can be both excitatory and inhibitory. Some have direct pain implications such as the neuropeptides, the endorphins and the encephalins, which are widely distributed throughout the CNS and the PNS. The increasing understanding of what happens in the synapse will potentially result in more selective analgesics which will be capable of targeting and blocking the passage of stimuli. This will result in suppressing aversive sensations without impeding function; for example, in a limb, pain will be removed but the reception of other information such as the position and temperature of the limb will remain.
The importance of the biochemistry of the synapse is apparent every day in theatre and in ICU as this is the site of action which allows neuromuscular blockade; this permits a state of relaxation to allow intubation and ventilation, overcoming the normal body defence mechanism of cough and gag.
Chemically-induced paralysis takes place at the neuromuscular junction, which is the point where the motor neurones extending from the spinal cord make contact with the muscle fibres. Present, at that point, are the potential transmitters which, if triggered, will result in a muscle contraction. Neuromuscular blocking drugs (Table 13.2) target this action and prevent the transmission of electrical impulses in a number of ways. They can bind with the receptor sites of the neurotransmitters so blocking the normal pathway, or they can compete with the neurotransmitters and bind with the site while not causing a muscle contraction.
Table 13.2 Muscle relaxants used in ITU
Source: BNF for Children 2011–12.
Drug | Examples | Actions |
Non-depolarising neuromuscular blocking drugs | Aminosteroid group: pancuronium rocuronium vecuronium Benzylisoquinolinium group: atracurium cisatracurium mivacurium. | Non-depolarising neuromuscular blocking drugs have a slow onset and can be classified by the length of time they maintain their action. There is a caveat of dosage. Short-acting: 15–30 minutes. Intermediate-acting: 30–40 minutes. Long-acting: 60–120 minutes. Although the child may be still and apparently sleeping the children’s nurse needs to remember that non-depolarising neuromuscular blocking drugs have no sedative or analgesic effects. These are powerful drugs and have complex side-effects. If used, the nurse should refer to the BNF for Children for guidance. |
Depolarising neuromuscular blocking drugs | Suxamethonium | This has the fastest onset of action of any of the neuromuscular blocking drugs. Its effect is brief so it is ideal for rapid-sequence induction for tracheal intubation. Most intubation policies give additional agents such as analgesia and atropine before an agent such as suxamethonium. |
NB: When muscle relaxants are used in an ICU setting it is good practice to discontinue them every 24 hours so that the level of sedation and analgesic can be assessed.
The use of paralysing agents in the intensive care environment is vital to maintain an airway, prohibit the normal cough and gag response an endotracheal tube would stimulate and ensure the child will tolerate the tube and not try to breathe against the ventilator. Unfortunately, they make the child very vulnerable and dependent on good nursing care and management. These children need one-to-one nursing and all ventilator pressure alarms set in case of accidental disconnection. In addition, all monitors which record the child’s vital signs need to be set within age-appropriate parameters in case of accidental extubation.
Biology of Pain and Sedation
The understanding of pain is incomplete and research continues to build on current knowledge. At present there is a consensus that there are two types of acute pain: fast and slow. The perception of fast pain occurs almost instantly. It is precisely located, unique in character and identified by sudden sharp, pricking sensations. In contrast, slow pain is of later onset, perhaps a second or so after the initial stimulus. The unpleasant situation builds and escalates in intensity, becoming burning, aching and/or throbbing. It is less easy to localise and in some cases may be referred (Jenkins et al. 2006). Relating the biology to the perception of pain is called nociception and depends on the intact functioning of the nervous system. The biology may be clear-cut but the individual’s experience of pain is less so. To counter pain experience and manage the clinical situation, pharmacological, cognitive strategies and holistic strategies should be employed in a multimodal approach.
The stimulus of acute pain is transmitted in two pathways: via a three-neurone system to the cerebral hemisphere on the opposite side of brain from the pain location and by a two-neurone system transmitting the stimulus to the cerebral hemisphere on the same side of the brain to the sensation (Waugh and Grant 2006). For pain to be experienced the following sequence has to take place:
- There has to be an event, a stimulus and a trigger strong enough to fire a receptor neurone. This stimulus will travel by neurone 1 to the spinal cord by the posterior route.
- Once in the spinal cord decussation takes place; this can be compared to a circuit, with some messages being transported via one route and other messages by another. At this level it can be seen why some impulses generate a crude reflex withdrawal and others are conveyed for more precise interpretation.
- These impulses are transmitted via the anteriorolateral spinothalamic tract to the thalamus and neurone 3, where processing at the perceptual level can begin (Marieb 2004).
Pain Processing
Neurone 3 conducts the stimulus to the higher brain centres of the limbic system and the cerebral cortex. Areas of the cerebral cortex correspond to parts of the body and these areas are probably responsible for the interpretation of what is perceived as pain. In an instant this is matched with previous experiences – the stored memories of pain. The pain stimulus is, in essence, made sense of and placed in context. There is also an understanding of what the individual’s pain experience might mean to an outside observer and further lightning-quick networking influences decision-making about how the individual should react. This is conscious processing and is complex. (For greater understanding refer to texts in anatomy and physiology.) For children, there are fewer memories and less experience on which to draw, resulting in fewer learned clues on how to react. These factors account for the wide variety of pain responses and behaviours seen in children.
For the preterm and young infant there may be no previous experience and stored data on which to draw. There is significant debate as to the reception and perception of pain in the foetus (Fitzgerald 1995; Glover and Fisk 1996; Harrison 1996; James 1998; Lee et al. 2005). This debate could be extrapolated to include the extremely preterm infant (Slater et al. 2006). What can be accepted is that preterm and neonatal pain is physiologically disruptive and impacts on physiological stability as it occurs at a time when the infant is developmentally unprepared for the stimulus. Exposure to stimuli resulting in pain may promote a heightened peripheral sensitivity or dampen down the normal expected behavioural responses to pain indicative of altered development (Anand and Hall 2006). Neonatal nursing textbooks cover the topic extensively but it is astounding that, in some textbooks of neonatal medicine, pain is not found in the index. From a children’s nurse perspective, an additional worry is the fact that children vary in their ability to develop the concept of time and infants have no concept of time; accordingly, they do not have the ability to understand that the experience they are suffering is usually temporary and time-limited. Table 13.3 demonstrates the links between biology and practice.
Table 13.3 Linking biology to the clinical interpretation of a child’s pain
Aspect of pain | Implication for the child |
Pain threshold | The level at which the stimulus will exceed sensory tolerance and be perceived as pain. This seems not to vary much among individuals or in the same person over time. |
Perceptual dominance | The phenomenon which seeks to explain why an individual with several sites of pain focuses on the most extreme pain experience. |
Pain tolerance | The duration of time in which an individual will tolerate pain until prompted to seek relief. This varies considerably among individuals and within an individual over time. |
Radicular pain | The pain which arises from a major nerve and is felt along the distribution of the nerve. |
Referred pain | The pain perceived in an area removed from its point of origin. The point of origin is mainly visceral and is thought to occur as a result of the internal organs having few sensory nerves. |
Incidence pain | Occurs when pain is provoked or intensified by movement or activity. |
Pain Classifications
The terms nociceptive pain and non-nociceptive pain are an attempt to classify pain using biology as a basis and create a taxonomy aimed at understanding the condition usually from a mechanical perspective.
Nociceptive Pain or Non-Nociceptive Pain
Nociceptive pain originates from cell damage, resulting in the release of inflammatory mediators; these bathe and sensitise the nociceptors which activate the pain cascade (Butcher 2004). Nociceptive pain can be superficial, from the skin, subcutaneous tissue or mucous membranes and can be characterised by a well-localised, sharp, pricking and throbbing experience (Butcher 2004) or may be somatic, from the deeper tissues such as muscle tendons and joints. This is less well localised and is characterised by aching, gnawing and constant pain. Visceral pain results from affected organs, causing sensations of deep, dull, dragging spasms or squeezing, colicky pain and is frequently associated with vomiting and alterations in vital signs (Butcher 2004).
Non-nociceptive pain, such as neuropathic pain, can vary in nature and severity. This pain is often caused by over-sensitisation of the nerves and is frequently associated with numbness or paraesthesia (Butcher 2004). The symptoms of which the patient complains reflect the nerve fibre affected. Burning and itching indicate that the C fibres are the causative nerves, whereas colour and temperature changes are a result of activity of the B fibres (Butcher 2004).
Acute or Chronic Pain
Pain is further divided into chronic and acute pain in an attempt to define it according to a time frame. Chronic pain is usually defined as pain which persists for 3 months beyond the usual course of an acute disease or reasonable time for an injury to heal or is associated with a chronological pathological process which causes continuous pain, or pain which recurs at intervals for months or years. Prolonged pain is disabling and can interfere with physical functioning (Shaw 2006).
From a clinical perspective there can be problems with all the information summarised in previous sections. Nurses seek to interpret what information is elicited from the assessment of an individual but this is complicated when the child is cognitively impaired (Dowling 2004), pre-verbal or sedated. Signs, symptoms and a history of the event will assist, but the most effective pain assessment requires qualitative as well as quantitative analysis.
Signs and Symptoms of Pain
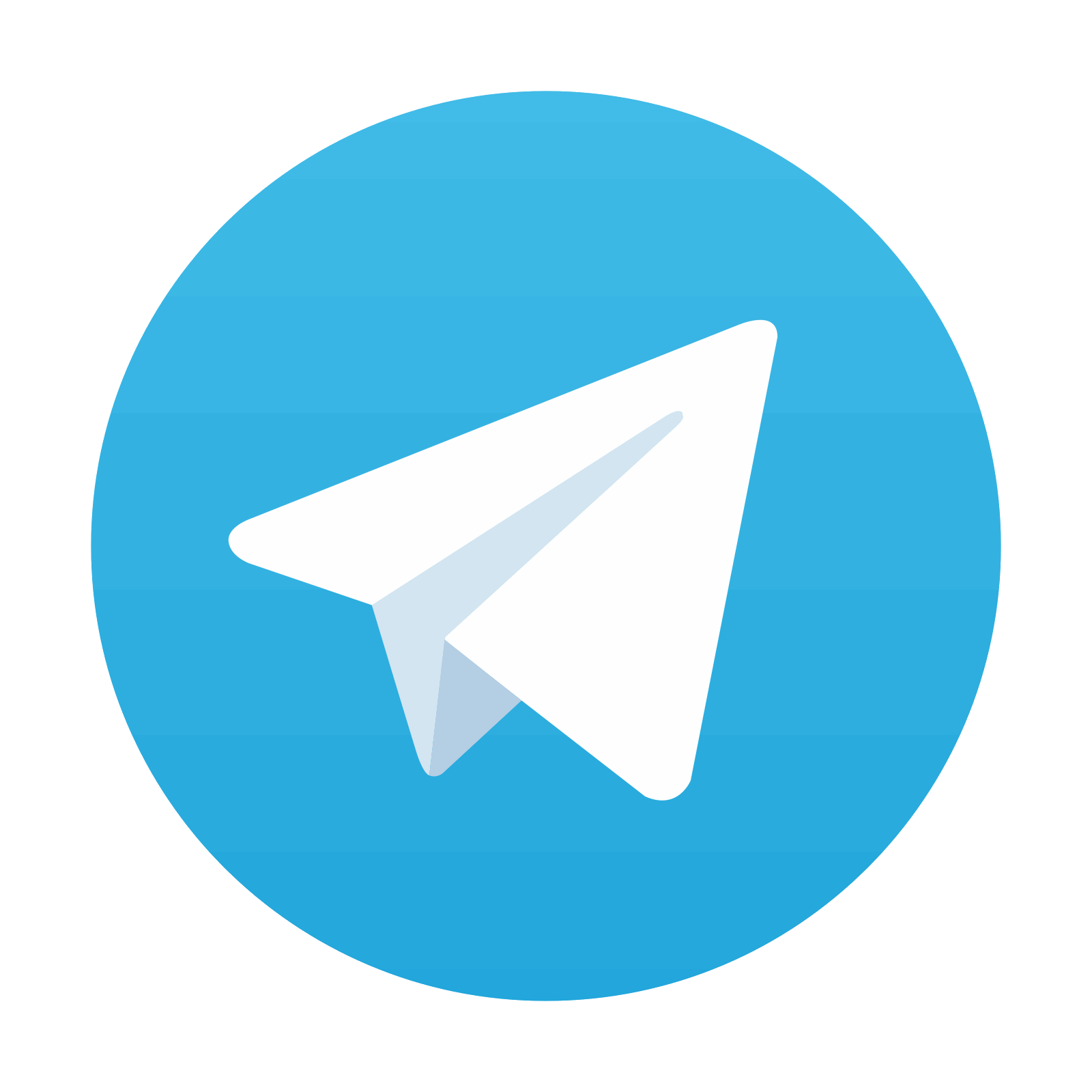
Stay updated, free articles. Join our Telegram channel

Full access? Get Clinical Tree
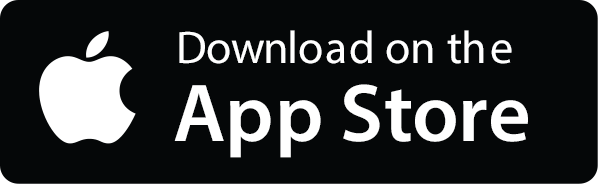
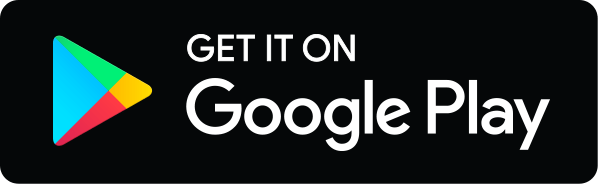