Chapter 29. Caring for children with cardiovascular problems
Barbara V. Novak
LEARNING OUTCOMES
• Critically review the embryonic and fetal development of the cardiovascular system.
• Demonstrate understanding of the specific anatomical and physiological features of the cardiovascular system.
• Detail the nomenclature of congenital cardiovascular disease in childhood.
• Outline the nature of acquired cardiovascular problems in childhood.
• Outline the assessment of a child with suspected cardiovascular problem.
• Detail the strategy for evidence-based nursing care of a child with cardiovascular problems.
Introduction
Some of the most complex childhood health problems are related to cardiovascular defects and anomalies. The cause of these defects and anomalies are not always known, although a range of contributing factors such as folate deficiencies, intrauterine infection and chromosomal abnormalities may play decisive roles. In general, congenital cardiovascular defects and anomalies occur in 8–10% of children born alive (Hoffman, 2003 and Sommer et al., 2008). Given the complexities involved in the development of the embryonic cardiovascular system it is not surprising that they occur this frequently. Many of the congenital cardiovascular defects and anomalies can be diagnosed in utero before birth. Other problems may be detected within the first few months of birth, usually due to the child manifesting persistent central and peripheral cyanosis often accompanied by respiratory difficulties. A small number of defects and anomalies may not be detected until later in childhood or during adult life. Irrespective of when the symptoms appear in most instances the clinical management of congenital cardiovascular problems will require specialist medical, surgical and nursing interventions.
Improved health care and better infection control are known to have reduced the incidence of acquired cardiovascular disease such as bacterial endocarditis in childhood. Nevertheless, viral myocarditis, cardiomyopathies and secondary cardiovascular disease caused by metabolic problems can arise in children and will require specific, at times life saving, evidence-based therapeutic interventions.
The normal cardiovascular system
The normal circulatory system can be divided into two functional compartments: the systemic circulation, which is supported by the left ventricle; and the pulmonary circulation, which is supported by the right ventricle. The right and the left ventricles work in relative harmony. This ensures that the systemic circulation transports oxygenated blood and nutrients from the left ventricle through the aorta to the systemic arteries and capillaries. The pulmonary circulation receives its deoxygenated blood from the right ventricle. This blood is then pumped through the pulmonary artery to lungs. Here a complex capillary bed surrounds the alveoli and facilitates gaseous exchange across the capillary-alveolar membranes. This mechanism ensures that oxygenated blood is transported to the left atrium by way of pulmonary venules, which anastomose giving rise to four pulmonary veins which commonly attach to the posterior wall of the left atrium. The oxygenated blood flows from the left atrium to the left ventricle which ejects it into the aorta ensuring that the metabolic needs of all organs and tissue are met.
The development of the cardiovascular system
The developed cardiovascular system is an adaptive but relatively efficient transport network supported by a rhythmically pulsatile four-chambered heart. Its role is to transport blood within the closed circuit of semi-compliant vessels, delivering nutrients and metabolic substrates from the placenta to the embry/fetus and removing fetus metabolic waste products back to the placenta. The anatomical developments and corresponding functional adaptations are dependent on the existence of a vast range of factors including the appropriate expressions of the human genome which masterminds the embryonic development and contributes to the establishment of the primitive cardiovascular system.
Scenario

Christopher, a third-year child branch student nurse, reported to his seminar colleagues that a number of children had been admitted to his ward with a history of respiratory difficulties and cyanosis. The evidence drawn from the physical assessments suggests that these children are suffering from congenital cyanotic cardiac anomalies. The severity of the respiratory difficulties and cyanosis means that these children require specialist hospitalised care. Christopher asked that the seminar should focus on the normal embryonic development of the heart and then critically examine the anatomy and physiology of the cardiovascular system in children.
The origins and development of the embryonic and fetal heart
The heart and its corresponding blood vessels develop from the splanchnopleuric mesoderm (embryonic tissue adjacent to the endoderm) in the cardiogenic region. The underlying endoderm contributes to this development by signalling to the primitive angioblastic cords to converge and form a pair of lateral endocardial tubes, which are then gradually fused and reshaped as the embryo grows and folds. This gradual, but carefully orchestrated, fusion of these tubes shapes the single primitive heart tube, which is then remodelled by a sequential process of septation and folding that transforms the single lumen into the four chambers of the definitive heart. The initial chambers are primitive, and require considerable development.
Activity
Activity


Critically reflect on the embryonic developments of the heart and the blood vessels and consider how such developments contribute to the shaping of the fetal circulation.
The shaping of the embryonic cardiovascular system
The primitive heart tube consists of endothelium which is shaped by a series of definitive expansions, shallow ridges and crevices (sulci). By the 22nd day a thick mass of splanchnopleuric mesoderm surrounds this heart tube, differentiating into the loosely organised myocardium and cardiac jelly, a layer of thick acellular matrix synthesised by the developing myocardium (Larsen 2008). The visceral pericardium lies externally to the myocardium. It evolves from mesothelial cells, which are derived independently from the splanchnopleuric mesoderm. These cells are believed to migrate onto the surface of the developing heart from the regions of the septum transversum or the sinus venosus.
Approximately, from the 23rd day, the single heart tube begins to elongate, simultaneously loop and fold. This displaces the bulbus cordis to the right and adjusts the position of the primitive ventricle to the left, allowing the primitive atrium to move in an upward direction. The complex cardiac folding is believed to be completed by the 28th day of embryonic life. According to Larsen (2008), this highly organised looping of the primitive heart tube may be intrinsically motivated and possibly influenced by the state of hydration of the cardiac jelly, active migration and development of the primitive myocytes (cardiac muscle cells), and local haemodynamic forces initiated by the embryo’s circulating blood. This early cardiac morphogenesis includes the formation of the coronary vascular plexus, which later forms the coronary vessels.
The mesoderm, mesodermal growth factors and other factors expressed in the developing myocardium influence tissue differentiation and regulate the course of atrial and ventricular development. The cardiac neural crest contributes to the formation of the primitive ventricular outflow tracts. The internal septation of the heart, and the formation of the heart valves, are attributed to the development of the mesenchymal tissue at the atrioventricular and proximal outflow regions of the heart. The structural developments in the heart and blood vessels may play a role in regulating the functions of the cardiovascular system. For instance, marginal increases in blood pressure may contribute to the development of the embryonic myocardium and the smooth muscle of the blood vessels.
The primitive heart begins to contract on approximately the 22nd day (Larsen 2008) and blood begins to circulate through the primitive vessels within the next 2 days. The first blood cells found in the embryo’s circulation are formed in the yolk sac, although embryonic and later fetal haematopoiesis occurs in the liver, spleen, thymus and, of course, eventually the bone marrow. The sustained rhythmic myocardial contractility may, in part, be dependent on myocardial hypertrophy, which is a basic adaptive response of the cardiac myocytes to an increasing workload. Polin & Fox (2004) suggest that myotropin (which may play an important part in the pathogenesis of myocardial hypertrophy) could act as an important primary modulator responsible for enhancing myocyte protein synthesis and myocyte growth and differentiation.
The developing atria
The right atrium is remodelled during the 4th and 5th weeks of gestation. This initiates the gradual incorporation of the enlarged right sinus horn and the development of the venae cavae. A single pulmonary vein sprouts from the posterior wall of the left atrium and then divides to form the right and left pulmonary veins, which grow in the direction of the primitive lungs, where they join the smaller pulmonary veins which develop around the primitive bronchial tissue. The right and left pulmonary veins are eventually reconstructed forming, in most instances, four pulmonary veins.
The anatomical divisions between the atria and the common atrioventricular canal separate the systemic from the pulmonary circulation. This separation also depends on the gradual fusion of the septum primum and the septum secundum. The septum primum establishes a firm interatrial muscle and the septum secundum contributes to the development of the foramen ovale, or oval window. Throughout the embryonic and early fetal life, all septal structures form large openings that allow blood to flow in a specific direction. The foramen ovale is one of the most important of these openings because it permits shunting of blood from the right atrium to the left atrium. This mechanism allows the blood to bypass the right ventricle, the pulmonary artery and its tributaries. The foramen ovale normally closes soon after birth, partly because of the abrupt cessation of fetal circulation at birth, with the first breath and the eventual dilatation of the pulmonary vessels. These mechanisms reverse the pressure difference between the atria and push the flexible membranous part of the septum against the less conforming muscular septum thus closing the foramen ovale and blocking any shunting of blood from the right to the left atrium.
Division and shaping of the common atrioventricular canal by the septum intermedium (the middle segment of the intraventricular septum) into the right and left atrioventricular canals ensures that the right atrioventricular canal aligns the right atrium with the right ventricle and the left atrioventricular canal aligns the left atrium with the left ventricle. The division and reshaping of the common atrioventricular canal initiates the development of the primitive left ventricle, which establishes a firm contact with the proximal portion of the truncus arteriosus, a major vessel, which ultimately divides to form the future aorta and the main pulmonary artery. The four main cardiac valves begin to form in the 5th week of gestation, almost immediately following the division of the atrioventricular canal, and the septation of the truncus arteriosus, and is initially supported by growth and shaping of ventricular muscle from below. However, proliferating cells, which surround these orifices eventually, form the distinctive mitral and tricuspid valves.
The developing ventricles
The right and the left ventricles are composite structures. According to Larsen (2008) the right ventricle is derived predominantly from the inferior aspects of the bulbus cordis and the right aspect of the conus cordis. The left ventricle develops mainly from the primitive ventricle and the left wall of the conus cordis. Corresponding outflow tracts, namely, the main pulmonary artery and the aorta, complete the morphogenesis of the heart and its major vessels. The intraventricular septum is formed by the developing right and left ventricular walls opposing one another (Collins 2008). The growth of the muscular part of the septum halts just before its superior edge meets the inferior membranous surface of the septum intermedium in the middle segment of the intraventricular septum. This temporary arrest in growth is associated with a number of developmental benefits including ventricular enlargement and formation of the ventricular trabeculae (ridges in the myocardium), which shape the ventricular chambers and their respective outflow tracts so that the heart can function as a competent mechanical pump.
The truncus arteriosus, which serves as the main cardiac outflow pathway, is divided by a septum which shapes the distal aspects of the right and left outflow tracts for the right and left ventricles respectively. As the aorta separates from the main pulmonary artery three distinctive swellings (tubercules) evolve initiating the development of the semilunar valves, also known as the aortic and the pulmonary artery valves which direct the intracardiac blood flow (Larsen 2008).
The final stage in the intracardiac development involves the growth of the membranous interventricular septum, which is originally derived from the inferior endocardial cushion. As the membranous septum fuses with the muscular interventricular septum at about 8 weeks of gestation blood flow between the right and the left ventricles ceases. The aorta and the pulmonary artery ensures that both ventricles can now function autonomously with respect to their own outflow tracts. In instances where fusion between the membranous and muscular parts of the septum fails a septal or intra-ventricular septal defect (VSD) will persist. This is the most common type of congenital cardiac anomaly that occurs in children.
The development of the valves
The atrioventricular valves begin their development in the 5th week of gestation with the formation of cusps within the atrioventricular canals. Generally, the left atrioventricular valve has only two cusps, which form the bicuspid valve, also known as the mitral valve. In contrast, the right atrioventricular valve usually, but not always, develops three cusps, which shape the tricuspid valve. The free edges of these cusps attach to the sinew-like chordae tendineae, which insert into the ventricular papillary muscles. The design of these valves ensures that during diastole the cusps fold back allowing the blood to flow uninhibited from the atria to the ventricles. Significantly, during systole, the tight closure of these cusps prevents a backflow of blood from the atria into the ventricles.
Chamber and vessel concordance
Primitive blood vessels begin to appear in the splanchnopleuric mesoderm of the yolk sac on the 17th day. A similar aggregation of cells, also known as blood islands, appears in the embryonic disc on the 18th day. These cells initiate the formation of the embryo’s blood vessels which, according to Colins (2008), are initially surrounded by a fibronectin rich matrix which is later incorporated into the endothelial lamina. The early development and subsequent reshaping of the endocardial tubes allows a pair of dorsal aortas to attach to their cranial axis and then form the first pair of aortic arches. Four additional aortic arches develop during the 4th and 5th weeks, connecting the rudimentary aortic sac (also known as the truncus arteriosus) to the dorsal aortas, which fuse later to form the median dorsal aorta. This complex network of the aortic arches is eventually remodeled to establish arteries within the upper thorax, neck and head. The dorsal aorta gives rise to three distinctive sets of arterial vessels (Collins, 2008 and Larsen, 2008):
• The ventral arteries, which supply the gut
• The lateral arteries, which supply the retroperitoneal organs such as the kidneys and the gonads
• The intersegmental arteries, which supply, in part, the head, the neck and body walls.
The arteries supplying the gastrointestinal tract are derived from remnants of vitelline arteries and the vitelline duct, which anastomose with the paired dorsal aortae. The dorsal aortae in turn connect to the umbilical arteries, which carry blood from the embryo, and later the fetus, to the placenta.
The primitive venous system consists of three major components:
• The cardinal veins, which drain the head, neck, body walls and limbs
• The vitelline veins, which initially drain the yolk sac
• The umbilical veins, which carry oxygenated blood from the placenta to the embryo/fetus.
All three sets of veins initially drain into the right and left sinus horns but their extensive modification shapes the distinctive systemic venous connection with the right atrium. The left sinus horn is eventually transformed into the coronary sinus and the oblique vein of the left atrium. The coronary sinus receives most of the venous blood returning from the coronary vascular bed. The vitelline venous system gives rise to the liver sinusoids and the portal system that transports venous blood from the intestinal tract to the liver. The vitelline system further subdivides within the liver establishing the ductus venosus, a small vessel that directs the embryonic/fetal blood from the umbilical vein directly into the inferior vena cava.
The complex arrangement of the heart is attained by the 55th day of gestation (Larsen 2008). The remaining period of gestation facilitates growth and relative maturation of the cardiovascular structures and corresponding haemodynamic adaptation. Myocardial cells proliferate in conjunction with the rapid accumulation of contractile proteins and metabolic substrates.
Activity

Read
•Chapter 22 (The circulatory system) in Hoffman (2003 pp 1745–1904).
•Chapter 21 (Blood vessels and circulation) in Martini and Nath (2009 pp 719–775).
• Critically review the anatomical and physiological characteristics of the fetal circulation.
• Give a reasoned account of the adaptations, which need to occur in the fetal circulation shortly after the birth of a child.
• Give a rationale for the differences between the fetal circulation and the assumed normal circulation of a 7-year-old child.
Developmental changes in the cardiovascular system
The normal position and shape of the heart in children
In children, the heart is a small, rounded, three-dimensional pyramid that lies in the mediastinal cavity more horizontally than in adults. The apex of the heart is normally higher and located in the fourth left intercostal space, extending only to the fifth intercostal space by 7 or 8 years of age. The base of the heart lies in an oblique position behind the sternum. It consists of the atria and their respective auricles (small appendages of each atrium). The two ventricles form the inferior aspect of the cardiac silhouette coming together to form the apex.
A mature heart weighs between 230 and 340 g, and this weight generally reflects the person’s body size. However, according to Gatzoulis (2008), this weight is achieved only between 17 and 20 years of age. In children, the normal heart is relatively small, the size varying with age and body surface area. The heart is suspended in a pericardial sac by its attachments to the aorta and the pulmonary artery. This leaves the apex relatively free and allows the ventricles to move the apex forward to strike against the left side of the chest wall during contraction (systole). This characteristic thrust is best heard as the apex beat on auscultation and is frequently referred to as the point of maximal intensity.
Activity

• Name the three anatomically distinctive layers of the heart.
• Give a reasoned account of the functions of these distinctive layers.
The pericardium
The fibrous pericardium encloses, and to some extent protects, the heart. It forms a continuum with the adventitia of the major veins and arteries that communicate with the heart. The serous pericardium is a double-layered membrane that consists of flat secretory epithelium, connective tissue and some adipose tissue, which is present in insignificant quantities in children. The two layers of the serous pericardium form a pericardial cavity, which holds a very small volume of serous fluid that acts as a lubricant preventing the occurrence of friction as the two membranes glide over each other during systole.
The myocardium
The myocardium, the contractile muscle of the heart, is composed of highly specialised small myocytes rich in specialised contractile proteins, mitochondria and sarcoplasmic reticulum. During embryonic and fetal life the myocardium develops as a result of a gradual increase in myocyte numbers and the intrinsically sustainable contractility. The relatively slow development of myofibrils (contractile elements) within the myocytes throughout fetal life, during infancy and childhood appears to contribute to a characteristic myocyte hypertrophy (i.e. an increase in the size of the myocytes). After birth, in children of all ages, the capacity for myocyte hyperplasia may be limited but myocyte hypertrophy will continue, under normal physiological conditions, up to 20 years of age.
Sources of chemical energy for the myocardium
The chemical energy for the contraction of heart muscle is derived from the metabolism of carbohydrates and fatty acids. However, because the amount of oxygen required for fatty acid metabolism is 11% more than that required for carbohydrate metabolism, to produce an equivalent quantity of adenosine triphosphate, fatty acids are not as efficient as glucose in terms of providing metabolic fuel or energy. Nevertheless, the adult myocardium has a strong preference for fatty acids. This contrasts with the preferences of the fetal and neonatal myocardium for carbohydrates or glucose. This may be clinically significant in circumstances of hypoglycaemia, which could compromise myocardial contractility and so cardiovascular function in neonates and possibly young children (Gluckman & Heymann 1996). Booker (1998) reported significant postnatal increases in myocardial contractility possibly attributable to increases in contractile protein, the delivery of Ca 2+ to the myofilaments, enhanced sensitivity of troponin to Ca 2+ and reduced sensitivity to acidosis. The timing of the transition from the use of glucose to fatty acids as the dominant metabolic fuel is not known, although it is possible that this transition is a gradual process influenced by the child’s diet and maturation of the myocardium.
The endocardium
The endocardium is composed of a specialised endothelium and a layer of fibroelastic connective tissue that lines the inner surfaces of the heart including the four cardiac valves. Characteristically, the endocardium is thicker in the atria than the ventricles and this may have a functional significance yet to be determined.
Electrophysiology: functional features of the heart
The coordinated contraction of myocardium provides the mechanical energy for the transportation of blood throughout the entire vasculature. Both the electrodynamic controls of the heart and the force of myocardial contractility are the consequence of the sophisticated cellular mechanisms that bring about the rhythmic excitation–contraction coupling phenomena. In the fetus, the right ventricle ejects approximately 60% of blood while the left ventricle ejects only 40% of the total cardiac output (Hoffman 2003). As both ventricles pump blood against a similar vascular resistance, there is little difference in the thickness of the myocardium as the two ventricles develop. However, in some instances the right ventricular wall is slightly thicker than the left ventricular wall, and this persists for a considerable time after birth. Electrocardiographic (ECG) recordings reflect this phenomenon as a relative right ventricular dominance (Hoffman 2003 pp 1757–1762). The right ventricular hypertrophy is generally attributed to the workload of the right ventricle and associated physical development of the myocardium during fetal life. A clear distinction must, however, be made between the physiological right ventricular hypertrophy and a hypertrophy initiated by some form of pathophysiological change that may affect the competence of the entire cardiovascular and pulmonary systems in neonates and children.
Activity

• Give a reasoned explanation for the significant differences in the normal ranges in children’s heart beats identified in Table 29.1.
Age of the child | Normal range (beats per minute) | Average (beats per minute) |
---|---|---|
Newborn–6 months of age | 90–160 | 120 |
6–12 months of age | 80–140 | 110 |
1–3 years | 80–120 | 100 |
3–6 years | 75–115 | 90 |
6–9 years | 70–110 | 90 |
9–11 years | 70–105 | 85 |
11–14 years | 65–100 | 80 |
• Define bradycardia in the context of each of the age groups in Table 29.1.
• Define tachycardia in the context of each of the different age groups.
• In your view, is bradycardia or tachycardia more clinically significant in children; and why is this the case?
The faster heart rate in neonates and infants is largely attributed to the smaller size of the ventricular chambers, which are capable of ejecting only a small amount of blood, commonly referred to as the stroke volume, on each ventricular contraction. However, as the child’s metabolic demands are high, and these have to be met by an effective cardiac output (the quantity of blood pumped by the left ventricle per minute), the potential deficits created by the small stroke volume are corrected by the higher heart rate. As children grow older, their hearts – and especially the ventricular chambers – evolve, the stroke volume increases, and this contributes to the significant reduction of the heart rate. It is important, however, to appreciate that the normal heart rate and stroke volume in all children fluctuate with activity and crying.
The cardiac cycle
The cardiac cycle is defined as the time from the beginning of one heart beat to the beginning of the next. Each cardiac cycle is initiated and governed by an action potential generated within the sinoatrial node and conveyed rapidly to both atria. The fractional delay in the transfer of the impulse from the atria to the ventricles ensures that the atria contract before the ventricles, as a result of which the blood is pumped by the atria into the ventricles, increasing the ventricular volume, which in turn raises the stroke volume. The four phases of the cardiac cycle are commonly known as:
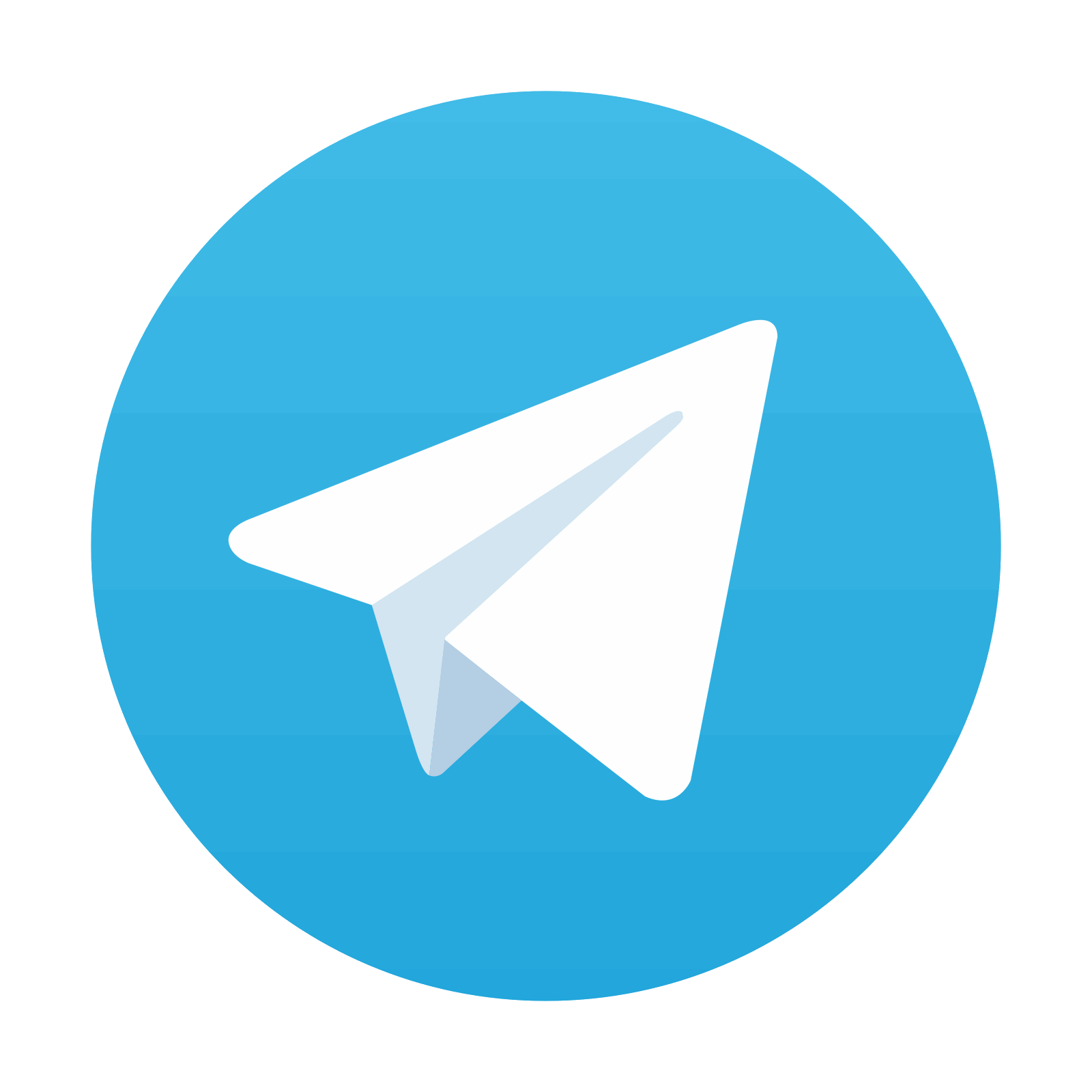
• contraction (phase I)
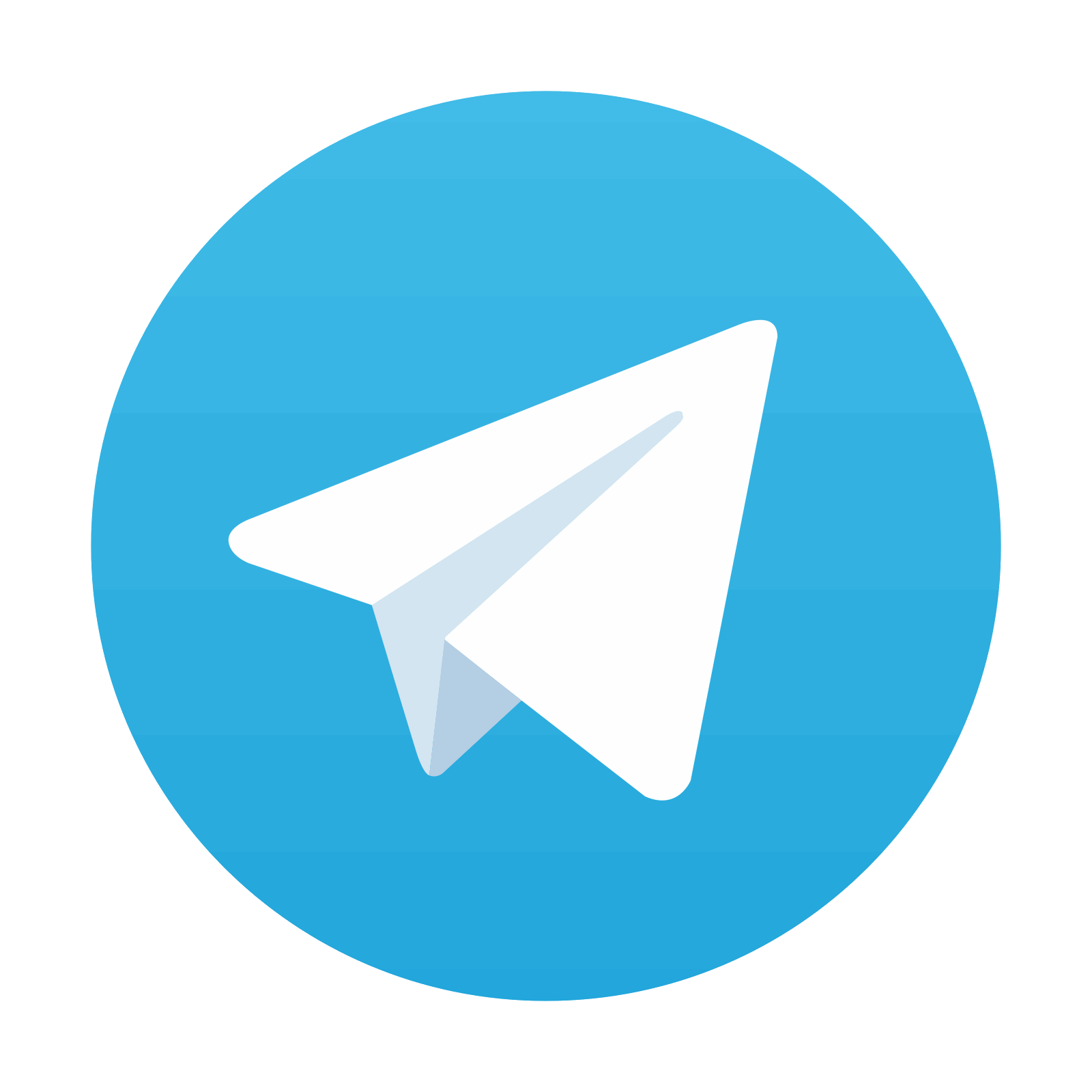
Stay updated, free articles. Join our Telegram channel

Full access? Get Clinical Tree
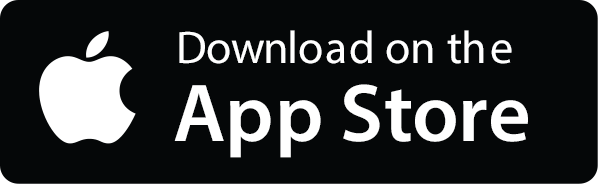
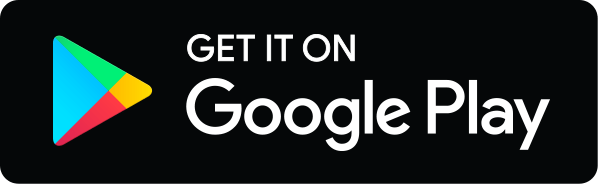
