Pronephroi
Non-functioning, main structures degenerate by day 25 but ducts remain to be utilised by mesonephroi.
Mesonephroi
Degeneration occurs at 10 weeks gestation but some tubules and ducts remain.
In the male:
Tubules → efferent ductules of the testes.
Ducts → epididymis, ductus deferens and ejaculatory duct.
In the female:
Ducts mostly disappear.
Tubules retained near uterus and broad ligament.
Metanephroi
As the nephrons continue to grow during foetal life the tubules continue to elongate, forming the proximal tubule, the loop of Henle (LOH) and the distal tubule. In week 10 the ends of distal tubules connect and join to form the collecting tubule or duct. The metanephroi produces urine from weeks 11−13 onwards.
Weeks 10−32
Glomerular apparatus, proximal and distal tubules are found within the cortex
Collecting ducts and LOH grow down into the medulla. Length increases after birth which increases kidney size.
Numbers of nephrons increase during foetal life until birth when full complement in place.
Postnatal glomeruli also increase in size, so rates of excretion increase progressively.
The first source is the common ileac artery, but as the embryo grows flow is derived from new branches off the aorta. Ultimately, all previous blood supply routes degenerate as the renal arteries become the key supply route.
Once blood is delivered to the kidney the renal arteries progressively subdivide delivering blood into the glomerular capsule via the afferent arteriole where simple filtration takes place.
Blood flows away from the glomeruli via the efferent arterioles which become the peritubular capillaries surrounding the tubules in the cortex, so allowing selective reabsorption, tubular secretion. Ultimately, the peritubular capillaries drain into the renal vein and blood flows away from the kidney. All these vessels have thin, semi-permeable walls to allow movement of solutes and electrolytes to and from the blood and nephron.
Anatomy and Physiology of the Kidney
The normal mature kidneys are paired structures located at the rear of the abdominal cavity in the retroperitoneal cavity. Each kidney receives blood from the renal arteries and drains into the renal veins. The kidneys have several functions and regulatory roles. They are essential in maintaining homeostatic balance with the regulation of electrolytes, maintenance of acid base balance and management of blood pressure by maintaining the salt and water balance.
The functional unit of the kidney is the nephron and this contains the glomeruli which are modified capillaries and serve as a filter of blood, removing waste, which is excreted in urine (Figure 6.1). The rate of filtration can be measured (Table 6.2).
Table 6.2 Glomerular filtration rate
Age | Rate |
Preterm (28 weeks gestation) | 10 ml/min/1.73 m2 |
Term | 20 ml/min/1.73 m2 |
Adult | 80−120 ml/min/1.73 m2 |
The kidneys also have a regulatory function and conserve water, glucose and amino acids by selective reabsorption according to need. The kidneys also produce a range of hormones and enzymes (e.g. calcitriol, erythropoietin and renin).
At the point of birth the kidney is relatively immature and conserves water poorly. This is an important consideration when administering drugs to neonates and young children (European Medicines Agency 2004). The immature developmental factors are significant in increasing the risk of developing AKI in children under 1 year. A shorter loop of Henle and reduction in functional ability compared to adults have a number of effects:
- Reduction in ability to concentrate urine.
- Inability to excrete sodium.
- Alteration of water reabsorption.
- Altered excretion of hydrogen ions.
- Reduced reabsorption of bicarbonate ions.
These factors make water balance and acid base balance control in metabolic acidosis more difficult. The reduced ability to control blood flow and cardiac output also causes potential damage in the younger child. The kidneys initially receive approximately 15% of cardiac output, but once mature this increases to 25%. Renal blood flow (RBF) is autoregulated and determined by the renal perfusion pressure (RPP) divided by the renal vascular resistance (RVR). This in turn is affected by the afferent and efferent arterioles in the glomeruli being constricted and dilated. Autoregulation allows the blood flow to the kidney to be constant even with large fluctuations of RPP. In infants the mean arterial pressure (MAP) is lower than in maturity, therefore the RBF is lower, making autoregulation effective in a much narrower range of MAP. Immature kidneys cannot cope with even small changes in blood pressure. This is compounded in the neonatal period by the increased levels of renin in this population, thought to be a mechanism to prevent excess sodium loss as the kidneys grow and the subsequent increase in GFR that take place. One of the effects of elevated renin levels is a decrease in cortical blood flow, thus further reducing the kidney’s ability to compensate for small reductions in blood pressure.
Renal Pathology (Table 6.3)
Insults to the kidney will have a number of effects which, if not reversed, may lead to acute tubular necrosis (ATN). Once ATN has developed, improvement to renal perfusion will not enhance urine output until the ATN has resolved, and this may take from days to weeks. The processes of ATN include vasoconstriction, desquamation of cells from the renal tubule leading to cast formation and impediment to flow in the tubule. This in turn may generate back-leakage of glomerular filtrate out of the renal tubule. Neutrophils will also adhere to ischaemic endothelial tissue, which in turn causes damage and further inflammation.
Table 6.3 Summary of diseases associated with types of renal failure
Type | Cause |
Prerenal | Hypovolaemia from any cause, including sepsis, cardiac failure, renal vein or artery thrombosis, persistent foetal circulation, cardiac bypass and dehydration including D&V. |
Intrinsic | Toxins, therapeutic agents, tumour lysis syndrome, hypoxia and birth asphyxia, haemolytic uraemic syndrome (HUS), glomerulonephritis, infections, massive rhabdomyolysis (e.g. following massive crush injury or electric shock). |
Postrenal (obstructive) | Urethral, ureteric obstruction, including blocked urinary catheter, kidney stones, tumours. |
AKI can be broken down into three broad categories: pre-renal, intrinsic renal and post renal (obstructive) uropathies.
Prerenal
Prerenal injury is caused by hypoperfusion from reduction in intravascular volume and/or hypoxia. In children this is usually associated with maldistribution of fluid associated with septic shock. However it can also be due to cardiac failure or shock from acute dehydration (Shaheen et al. 2007). If the hypoperfusion is short-lived and the kidneys are physiologically normal, restoration of intravascular volume will lead to speedy reversal of AKI. However, if it persists, a number of mechanisms come into play to avoid further intrinsic renal damage. Initially, dilation of renal arterioles takes place in an attempt to maintain glomerular blood flow. Prostaglandin generation is also associated with hypoperfusion; vasodilatory effects are designed to increase renal perfusion. There is some suggestion that these processes are impeded or reversed with the use of a number of targeted drug therapies used in animal models, but their clinical use has not yet been fully evaluated (Knoderer et al. 2008; Kohda et al. 1998; Landoni et al. 2007; Marthur et al. 1999).
Intrinsic
While there are many diseases that can cause intrinsic renal injury, only the causes likely to lead to treatment in PICU are discussed in this chapter with the notable exception of haemolytic uraemic syndrome (HUS), which can vary in severity, and extreme forms of which can lead to admission to PICU.
The intrarenal category includes all physiological events directly affecting kidney tissue structure and function. This may be the end-point of prerenal failure, particularly with hypoxic or ischaemic injury. Intrarenal injury is characterised by cell damage from ischaemia after hypoperfusion or direct damage of nephronic cells from drugs, toxins or swelling. Whatever the cause, the outcome is cell death and apoptosis. Cell debris then obstructs the tubule, further compounding the damage to the tubule. If the process continues and is severe, acute tubular necrosis (ATN) will develop. This can be entirely reversible depending on the length and type of injury. The child in PICU is particularly at risk because of the need to use nephrotoxic agents in life-threatening conditions in association with multiorgan failure.
Postrenal (Obstructive)
Anything that reduces or obstructs flow from the urine-collecting duct to the exit of the bladder can cause AKI. Varying damage is caused by back-pressure on the kidneys. Postrenal AKI is rare in PICU, but should not be ruled out, primarily because it can be caused by urethral obstruction from posterior urethral values. Ureteric obstructions can include kidney stones or tumours. Always ensure a urinary catheter is not blocked by flushing it with 1 ml/kg 0.9% sodium chloride.
Although there are three classic classifications of renal failure in PICU, the causes can be attributed to a number of factors rather than one discrete event. The aim of treatment is to prevent further damage and treat complications of AKI. AKI in the PICU population accounts for about 2.5% of admissions (Shaheen et al. 2007).
Signs of Acute Kidney Injury and Useful Tests in Children
A good history may reveal that the child has suffered from bloody diarrhoea, proteinuria or another disease, making the child seriously unwell. Examples of the underlying causes include meningococcal sepsis, diarrhoea and vomiting, thermal injury, malaria, Weil’s disease/leptospirosis, tumour lysis, HUS or traumas such as crush injuries, electrocution and birth asphyxia. To date there are no specific biomarkers for AKI, but a number of physiologically indicators. Table 6.4 lists indicators that may be useful. Urea and creatinine are variable markers with a huge normal range, depending on age, disease process and level of physical activity. Severe renal impairment may be present with normal creatinine in children with little muscle bulk. Urea may be low in fluid overload or where liver failure is present.
Table 6.4 Indications of AKI
Newborn | Children | |
Urine output | <1 ml/kg/hr >4 ml/kg/hr without diuretics | <0.5 ml/kg/hr >7 ml/kg/hr without diuretics |
Urea | >10 mmol/l | >20 mmol/l |
Creatinine | >50 | >90 micromol/l |
Metabolic acidosis | Usually in conjunction with other signs of renal insufficiency | |
Hb/platelets | Low Hb and platelet count with raised urea and creatinine, suspect HUS |
It is essential to catheterise the patient when an AKI is suspected because of the need to assess urine output and effects of treatment. Urinary biochemistry may be useful in interpreting the type of AKI (Table 6.5). However, this cannot be used once diuretics have been initiated and acute fluid resuscitation has taken place.
Table 6.5 Indications of types of AKI from urinalysis
Other tests, such as urinalysis for blood protein, casts and MC&S (microscopy, culture and sensitivities) are also required.
Acute Treatment (Table 6.6)
Treatment priorities are:
- Restoration of circulating blood volume.
- Treatment of electrolyte disturbances.
- Nutrition.
- Prevention of complications.
Table 6.6 Treatment for electrolyte disturbance
Restoration of Circulating Volume
The judicious use of fluid restriction with accurate fluid balance in renal failure will prove useful. Very careful reassessment of fluid status should be made after 5 or 10 ml/kg boluses of fluid have been given. Ideally, the fluid that most closely resembles that being lost should be used. However in practice, at least initially, the use of 0.9% sodium chloride or 4.5% human albumin solutions are used. After initial restoration of circulating blood volume with fluid boluses it may be necessary to restrict the child to infusing a calculation based on the child’s urine output plus insensible losses to avoid fluid overload. This is impractical in the ITU because of the use of inotropes, sedation and the need for nutrition. In these cases the early use of renal replacement therapy (RRT) is essential as there is evidence that fluid overload is a significant factor in mortality in children treated with RRT (Goldstein et al. 2004). It should be remembered that in AKI symptoms can be due to acute intravascular water loss as well as fluid overload and the child’s inability to handle solutes and maintain acid base balance. Therefore, fluid balance and fluid resuscitation will always need to be considered as first-line treatment. However, strict assessment of fluid balance, where practical, including accurate weighing for fluid trends, should take place. It is also essential to consider insensible loss carefully. The insensible loss can be calculated as 330 ml/m2/day or 10 ml/kg/day. All forms of insensible loss should be taken into account, including increases from raised temperature, thermal injury, phototherapy and chylothorax.
Nutrition in AKI is essential to hasten recovery, prevent catabolism and reduce the need to control metabolic abnormalities. Suitable nutrition may avoid the need for RRT. However, in PICU where RRT is instigated for fluid balance or metabolic control, space for nutrition can be generated with ease. This is particularly important when dealing with a critically ill child who has a large calorific need. Nutrition should be managed with a dietician to maximise calories without additional protein. Where specialist renal feeds are not available, combinations of nutritional supplements and feed fortifiers will have to be handmade. These are often strange combinations which are complicated to make up.
Prevention of Complications
AKI has the potential to cause anticoagulation with platelet dysfunction, hypertension, fluid overload, gastrointestinal bleeding, immunocompromise and reduced ability to handle drugs and toxins. In addition, children who require intensive care will usually need multiple invasive lines and catheters. The need to adhere to strict infection control measures is essential. Full blood count, platelets, coagulation and biochemistry, including phosphate, magnesium, calcium and liver function tests, must be checked urgently if AKI is suspected or known to be involved. Frequency of repeated samples depends on the patient’s response, but it is essential to check levels when potentially dangerous or in assessing response to treatment, rather than hope for the best. Most blood gas machines now measure corrected calcium, sodium and potassium on small sample sizes, which can be very useful.
One of the effects of AKI, particularly with fluid overload, is hypertension and potentially a hypertensive crisis. Hypertension should be treated with nifedipine then labetalol, at a rate starting at 0.5 mg/kg/hr, up to a maximum 3 mg/kg/hr (Barry et al. 2010). Sodium nitroprusside (SNP) can then be used. SNP is a very potent vasodilator and will potentially lower blood pressure very quickly. It does, however, have a short half-life so once switched off, blood pressure (BP) will recover. It is essential to protect SNP from the light and change it regularly as it degrades into cyanide. The SNP infusion rate is 0.5mcg/kg/hr with a maximum of 8 mcg/kg/hr (Barry et al. 2010). Furosemide should only be used if the patient is not intravascularly fluid-depleted. It is essential to reduce the pressure at a controlled rate to avoid organ damage and prevent permanent neurological disability.
Because of the varying length of hypertension before treatment in PICU, BP should be reduced by 25% in the first 8 hours and then reduced to normal over the next 16–48 hours, that is to say the aim is to return blood pressure to expected values relative to age over a 48-hour period using a two-stage approach (Barry et al. 2010).
Renal Protection Measures
Low-dose dopamine (2–5 mcg/kg/min) has been demonstrated to dilate renal arteries and increase renal blood flow in animal studies. For some years low-dose dopamine was standard treatment in the PICU to generate this renal perfusion effect; however, it has largely been abandoned because of lack of evidence of improved renal function in clinical practice.
The use of furosemide infusions has gained some ground as an avoidance strategy for RRT and as a way of increasing urine output to stop re-instigation of RRT. Again there is no evidence to support this; anecdotally, the ability to control fluid balance may be useful in the avoidance of RRT. However, it is essential to remember that furosemide will work only when there is cortical blood flow. As it is also nephrotoxic its use should not continue if it does not have an effect.
Drug Metabolism and Excretion
The kidneys are responsible for the elimination of many drugs and their metabolites. This is through the process of glomerular filtration of drugs and active renal secretion. As glomerular filtration rate (GFR) drops in renal failure, drug removal becomes less efficient. Normal GFR is 120 ml/min per 1.73 m2. There are various calculations for estimating GFR; however they are difficult to interpret when diuretics and fluid resuscitation have been administered.
Changes to electrolytes, body water and uraemia will increase the effects of centrally acting drugs because of changes to the blood–brain barrier and to the distribution and amount of body water and therefore drug distribution.
There are further points to consider in renal failure especially when RRT is involved, these are detailed in Table 6.7.
Table 6.7 Characteristics of drugs
Drug factor | Implication for the child with impaired renal function |
Molecular weight | Molecular weight refers to the size of the drug molecule and will theoretically affect a drug’s removal. Most drugs, however, are small enough to fit through the semi-permeable membrane which forms the capillaries in the Bowman’s capsule. Once through into the tubule some drug may be reabsorbed along with water back into the peritubular capillaries. Eventually, the unabsorbed drug will pass into the collecting duct and be carried to the bladder. In RRT all non-protein and fat-bound drugs will be filtered with increasing efficiency as treatment rate goes up. Adding dialysate flow to CVVH will increase the efficiency of drug removal. |
Protein binding | Protein binding is one of the most important factors as drugs that are protein-bound (e.g. albumin) will not be filtered with the glomerular filtrate. Once unbound, the free drug may be removed. This is potentially highly significant in children who are critically ill as at least 50% of them will be hypoalbuminaemic and 25% will be severely hypoalbuminaemic. In addition, acidosis affects protein binding; this may lead to more unbound drug being available while having unchanged drug levels on drug assay. |
Water solubility | Water solubility is the ability of a drug to dissolve in water. Drugs that are highly water soluble will be likely to be removed by glomerular filtration. |
Volume of distribution | Volume of distribution depends on where the drug is likely to disperse through the body. Drugs with a high volume of distribution will be less available for glomerular filtration. Volume of distribution is often changed by fluid overload in renal failure. |
Renal excretion is the active removal of a drug and its metabolites by cells sensitive to drugs in the tubule. Many drugs have metabolites that are removed by the kidneys after initial metabolism in the liver.
Practical Drug Dosing
Ideally, known nephrotoxic agents should be avoided; however, this may be impossible in PICU. Dose or dose interval may have to be changed. Generally, loading doses should not be changed. As GFR improves or worsens, changes in dosage will have to be reassessed. When drug assays can be performed they should be done to aid decision-making on dosing and effects. Drugs with short half-lives, inotropes and inodilators should not have changes made to prescription as they are usually not affected by renal function.
Aminoglycosides, gentamicin, amikacin and tobramycin should be used with extreme caution in AKI in PICU. They are nephrotoxic and ototoxic and these risks are increased in renal failure. The effects are caused by reduction of intravascular volume, use of diuretics and hypokalaemia/magnesia, particularly in neonates. To reduce clinical incidents many units prescribe individual dosage of these agents rather than a course. This allows for individual consideration of the infant’s needs.
For gentamicin dosing Barry et al. (2010) and Rees et al. (2007) suggest the drug levels be monitored frequently but that the daily dosing schedule be replaced by a divided dosing regimen where 2.5–3.5 mg/kg be given when there is renal impairment. This should be reviewed if the child’s infection was not responding to management. Vancomycin will be removed by CVVH/DF, which is more efficient at drug removal than other forms of RRT, especially when using high-volume treatments. Vancomycin trough levels should be performed to determine dose schedule. Liposomal amphotericin B is considerably less nephrotoxic than conventional amphotericin and therefore dose adjustment is usually not necessary. Cephalosporin and penicillin will also need dose-adjustment.
Morphine and midazolam metabolites are removed by active renal secretion. Reduction in dose by up to 50% will usually be appropriate with full assessment of effects and sedation scoring. Some centres routinely change regimes from morphine to fentanyl once RRT is instigated.
The electrolyte content of drugs also needs to be considered in AKI (e.g. drugs with high sodium content such as Gaviscon and 4.5% human albumin solution).
Drug dosing in AKI and RRT is a highly complex, imprecise area. The advice of a specialist pharmacist should always be sought as well as up-to-date literature (BNFc 2011–12; Patzer 2008)
Long-Term Follow-Up
Long-term follow-up from AKI has until now been restricted to children with primary renal injury. It was thought that those who survived multi-organ failure would recover normal renal function. Some studies (Kist-van Holthe et al. 2002; Polito et al. 1998; Slack et al. 2005) suggest a need for long-term follow-up in those children.
Specific Renal Diseases That May Present in PICU
Haemolytic Uraemic Syndrome (HUS)
The syndrome is characterised by haemolytic anaemia with raised urea and creatinine, and low platelet count. Verotoxins are usually released after a diarrhoeal illness and cause a number of problems within the circulation. The toxins attack the endothelial cells in the kidney and brain; in addition, the breakdown of red cells and platelet aggregation partially or totally occlude blood supply in the kidneys. Usually, though not exclusively, this is associated with exposure to E. coli 0157, but can have a number of other causes. In most cases bloody diarrhoea is the first sign of illness although occasionally no diarrhoea is present and HUS may be the underlying pathology. While there is no specific treatment for HUS other than supportive management, RRT is often necessary to manage severe electrolyte disturbance and fluid imbalance. Occasionally, children develop severe cerebral irritation and cerebral bleeds, and this often necessitates intensive care for neurological protection and airway management. In these patients the use of plasma exchange has been found to be useful, possibly because of the removal of the toxin, but the process is not well understood. The use of all extracorporeal techniques in acute HUS can be challenging because of the tendency of circuits to clot owing to the large clumps of red cells and platelets occluding the circuit filters.
Strict barrier nursing is essential when dealing with cases of HUS because of the ease of transfer to staff and relatives. There are well-documented cases of fatalities in health care workers. In HUS blood film and stool culture will be helpful.
Nephrotic Syndrome
This occurs when the glomeruli become permeable to albumin. If unchecked the oncotic pressure can drop to a point where intravascular fluid is lost from the circulation. Acute treatment usually involves infusion of 20% albumin followed by furosemide. Occasionally, intravascular losses will cause very significant pulmonary oedema and ventilation may be required. It is then usually necessary to perform fluid removal with haemofiltration.
Hepatorenal Syndrome
This is renal failure secondary to hepatic failure. It should be considered when children with liver failure show signs of kidney insufficiency. Children may well be hypovolaemic despite the appearance of ascites. It is essential to replace intravascular volume if the central venous pressure (CVP) is low; however, CVP must not be raised above 10 mmHg because of the risk of further liver damage.
Tumour lysis Syndrome (TLS)
Tumour lysis occurs when tumours that are sensitive to chemotherapy are treated causing dead cells and cell debris to be released into the blood. Uric acid and calcium phosphate potentially precipitate in the renal tubules causing AKI. Large amounts of potassium will also be released, causing potentially life-threatening hyperkalaemia. Acute RRT may be necessary to treat symptoms in TLS. It may be avoided with aggressive fluid management, diuresis and possibly the use of rasburicase and allopurinol (Ahn et al. 2011; Sood et al. 2007).
Transplant
Kidney transplant patients are not routinely admitted to PICU unless undergoing a more complex procedure, including liver and kidney transplant or have comorbidity that requires intensive monitoring or ventilation. General principles will be discussed.
It is vital to ensure good communication between teams caring for these children, with coordination of ward rounds to include all the senior medical staff.
Immunosuppression is one of the most important aspects of immediate post-transplant care to avoid acute rejection of the transplanted kidney. The therapy causes significant immunocompromise and strict adherence to reverse-barrier nursing protocols is essential. Where practical the child should be nursed in a cubicle or at the very least away from other infectious children. Combinations of monoclonal antibodies that inhibit CD50, calcineurin inhibitors and corticosteroids are used to achieve immunosuppression and local protocols should be strictly adhered to, including the use of drug levels to assess dose effectiveness. Some of these agents (e.g. cyclosporine) are cytotoxic and the usual precautions should be taken. Tacrolimus and cyclosporine both interact with many drugs causing increased or decreased effect and nephrotoxicity. Of particular note in the PICU are: amphotericin, vancomycin, aminoglycosides, fluconazole, erythromycin, chloramphenicol, rifampicin, nifedipine, cimetidine, phenytoin, phenobarbitone and carbamazepine (Rees et al. 2007). This is not an exhaustive list and before administering any new agents to children on anti-rejection therapy the current British National Formulary for Children (BNFc) should be consulted.
Important postoperative factors include the need to be scrupulous about fluid balance and monitoring the function of kidney graft with the fluid allowance, including a calculation for urine replacement initially millilitre for millilitre to avoid dehydration. Other considerations are the risk of urine leakage from the ureteric anastomosis site. Urine leakage may be detected by an improvement in biochemistry without adequate urine output, although pain and swelling can also be a clue. The leak can be confirmed with ultrasound and will require surgical intervention to rectify it. Blood clots can restrict and occlude the transplanted kidney’s blood supply. Most centres overcome this with the use of heparin or aspirin and this can lead to bleeding problems postoperatively. Often, kidneys are transplanted from much older donors; consequently, a higher blood pressure than physiological normal for the child will be needed to ‘drive’ the new kidney.
Renal Replacement Therapies
Renal replacement therapy (RRT) has in important role in PICU.
Acronyms
Continuous veno-venous haemofiltration (CVVH) is a substitute for haemodialysis. There are different types and they all come under the general term renal replacement therapy. There has been an increase in the use of CVVH, CVVHD (continuous veno-venous haemodialysis) and CVVHDF (continuous veno-venous haemodiafiltration) in the last decade, with the availability of automated machines and paediatric tubing, filters and software. However, peritoneal dialysis (PD) still has a place. Choice of therapy should be based on advantages and disadvantages (Table 6.8), as well as experience with the setup, what the continuing treatment options are and the availability of each method.
Table 6.8 Advantages and disadvantages of different therapies
Therapy | Advantages | Disadvantages |
Peritoneal dialysis | Circulatory stable No anticoagulation Can be used in infants <2 kg Technically easy Less resource-intensive | Potential respiratory compromise Infection Catheter placement Less efficient Moderate fluid volume control |
CVVH/CVVHD/ CVVHDF | Efficient Circulatory stable after initial connection Efficient fluid balance control ‘Evil humour removal’ | Access Clotting/bleeding problems Platelet consumption Electrolyte disturbance, e.g. Ca2+/Mg/PO4 More technical |
Intermittent haemodialysis | Drug removal Toxin removal Quick Very efficient removal of wastes | Access Can cause severe circulatory instability Water supply needed Dedicated operator |
Peritoneal Dialysis
PD is not suitable for every child as there are a number of contraindications (Table 6.9). However, it is still widely used as a renal replacement therapy for children outside the specialist renal units. PD may be used, for example, during the postoperative phase of a cardiac patient, where the placement of a PD catheter can be done under direct visualisation.
Table 6.9 Contraindication and requirements for PD
Contraindications | Essential requirements |
Diaphragmatic hernia Omphalocoele Gastroschisis Possibility of intra-abdominal catastrophe (necrotising enterocolitis in most circumstances) Recent abdominal surgery Multiple adhesions Peritonitis Presence of VP shunt | Peritoneal access Dialysis fluid A delivery system incorporating timer and measuring device Fluid warmer |
Peritoneal Catheter Access
The best form of access is obtained by either laparoscopic surgical or percutaneous insertion of a tunnelled double-cuffed catheter, with antibiotic cover. Ideally, these should not be used for 2 weeks; however, in the PICU the need for acute biochemical control will circumvent this. There are ‘blind’ insertion acute peritoneal dialysis catheters, which can be used in an emergency; however these do have an increased complication rate, in particular bowel perforation, leakage, mechanical obstruction and infection. As with all practical procedures experience reduces the risk of complications.
Dialysis Fluid and Kinetic Principles
In PD the peritoneum acts as the semi-permeable membrane, allowing diffusion, convection and osmosis to play a role. The dialysis fluids use glucose as the main osmotic agent. The higher the glucose concentration, the greater the fluid removal. However, due to absorption of glucose this can lead to hyperglycaemia, thus the glucose concentration should be as low as possible to achieve the required fluid removal.
The dialysate is buffered with bicarbonate or lactate. In general there has been a trend to using bicarbonate solutions in PICU, as they can be used in liver failure and lactic acidosis and reduce infusion pain. Because of bicarbonate’s instability with calcium all bicarbonate-based fluids need to mixed before use and usually have a 24-hour expiry. Observation for calcium precipitation should always take place.
The dialysate fluid can be used as an effective method of temperature control. The fluid is normally heated to 37°C; however, a lower temperature provides an extremely effective central cooling.
Tubing and Connections
These are straightforward and come in sterile packaging. They are simply a means of connecting the dialysis fluid to a burette then to the catheter via a three-way tap, with the third connection to a waste bag fitted with a measuring device. They must be managed aseptically at all times. Luer lock connecters are preferable as they are more secure.
Cycles Time and Fluid Volumes (Table 6.10)
Volumes: The starting fill volume should be 10–15 ml/kg, which can be increased to 30 ml/kg if no leaks occur. Maximum volumes of 50 ml/kg can be used. The larger the volume the more diffusion can occur and thus increased efficiency of the dialysis. However, the larger the volume the more likely it is that there will be respiratory and cardiovascular instability and exit site leakage.
Table 6.10 Cycles of PD
Cycle | Time (min) | Comment |
Fill | 10 | |
Dwell | 30−60 | Reduce cycle time if fluid removal is the main aim. |
Drain | 10 | Continue drain if fluid is draining. |
There are automated machines to simplify the process of cycling. However, these cannot perform fill volumes below 100 ml and so they are unsuitable for newborns. Neonates have a very varied response to dwell times. Paradoxically, the reverse of what is physiologically expected may sometimes be the case.
Cross-flow: In situations where large volumes in the abdomen cannot be tolerated it is possible to insert two PD catheters. One can be used to fill and the other to drain. The fill volumes are the same; however it is infused and drained continuously over 1 hour.
Additives to peritoneal dialysis fluid: PD fluid can be added to if necessary, in particular with heparin, to prevent fibrin deposits (be aware of systemic absorption especially in neonates), antibiotics to prevent or treat peritonitis and potassium to prevent hypokalaemia. PD is efficient at removing potassium, therefore close monitoring of levels is essential. All additions to bags must strictly comply with infection control measures because of the increased risk of peritonitis.
Peritonitis
Peritonitis is one of the most important complications of PD as it can be life-threatening. The standard symptoms of pain, fever and cloudy PD fluid do not have to be present. Examples of antimicrobials that can be added to the PD fluid are shown in Tables 6.11 and 6.12. Because of the changing nature of the problem, drug choice should adhere to local policy based on bacterial sensitivities. There should be case-specific discussion with the pharmacist and microbiologist.
Table 6.11 Example regimen in PD
MRSA status | Management |
MRSA negative | Load with tobramycin (16 mg/l) and cefuroxime (500 mg/l). Subsequent maintenance doses as prescribed examples provided below. Dwell time 6 hours. |
MRSA positive | Load vancomycin (500 mg/l) and cefuroxime (500 mg/l). Subsequent maintenance doses as prescribed. Dwell time 6 hours. |
Table 6.12 PD fluid additives and dose
Therapeutic agent | Suggested dosage |
Cefuroxime | 125 mg/l |
Vancomycin | 30 mg/l |
Ceftazidime | 125 mg/l |
Teicoplanin | 20 mg/l |
Cefazolin | 125 mg/l |
Tobramycin | 8 mg/l |
Heparin | Max. 500 units/l |
Potassium | Max. 5 mmol/l; standard 4 mmol/l |
Drug Levels Initially at 48 Hours
Total treatment duration is 2 weeks, except for Staphylococcus aureus and Pseudomonas which should be 3 weeks. If infection persists or recurs, catheter removal may be necessary.
Fungal peritonitis can occur with few signs and often necessitates catheter removal and fluconazole treatment.
Where prophylaxis is the aim, cefuroxime at 125 mg/l is often the first choice.
Catheter Leakage
This is more common in the acute PD catheters and it greatly increases the likelihood of infection.
- Use occlusive dressings if possible.
- Apply pressure dressing to site. Remember to weigh prior to application in neonates, to allow calculation of fluid balance.
- Purse string suture around catheter.
- Consider a new catheter if leakage continues.
(See Table 6.13.)
Table 6.13 Troubleshooting PD
Problem | Cause | Solution |
No/reduced flow on drain | Clamped or kinked lines or catheter | Unclamp or unkink lines. |
Fibrin blockage | Flush catheter with heparin and 0.9% sodium chloride never aspirate. Add heparin to bags. | |
Position of catheter obstructing drain | Reposition patient. Some positions can be counterintuitive. | |
Fluid may have fully drained straight into drain bag because drain line not clamped | Clamp drain line clamp. Perform one cycle with no dwell time, observing closely for signs of over-filling. | |
More fluid removal required | Increase strength of dialysis fluid | Use higher-strength glucose bag. Try mixing of two strengths rather than going straight to a higher strength. |
Decrease dwell times | Shorten the length of time the dialysate stays in the patient. This can increase the fluid removal but can also have an effect on solute removal. | |
Increase fill volumes | Increasing the amount of fluid going into the patient (max. 50 ml/kg) can sometimes increase fluid removal but should be done cautiously. This will also increase the solute removal. | |
Too much fluid being removed | Decrease the glucose concentration | Use a weaker strength bag if using pre-made. Try mixing two strengths. |
Lengthen the dwell time | Leaving the dialysate in the patient for longer will remove less fluid. | |
More clearance of waste and electrolytes required | Urea | Longer dwell times are required to remove more urea. |
Potassium | Shorter dwell times are required to remove more potassium. Continuous dialysis can cause hypokalaemia, requiring the addition of potassium to the PD fluid. | |
Sodium | Sodium should be lowered slowly to avoid any adverse effects. 1 mmol/hr is a safe reference to use. Very hypernatraemic patients should have additional sodium chloride added to the dialysate to avoid lowering levels too quickly. | |
Calcium | Pre-made solutions are available with different calcium concentrations (range 0−1.75 mmol/l). | |
Creatinine | Creatinine is not removed very efficiently during short-dwell peritoneal dialysis. It is, however, a useful indicator of kidney function and should be observed in the acute setting for any improvement. | |
Pain on infusion | Internal position of catheter | Tidal dialysis can be tried in order to keep a pool of fluid in the peritoneum to float the catheter. Change patient position. Reposition catheter (acute only). |
Intra-abdominal pressure | Reduce fill volume. Try cross-flow dialysis. | |
Air under diaphragm | Normally corrects over 30 minutes. Analgesia. Try cross-flow dialysis. | |
Pain on outflow | Internal position of catheter | As for pain on infusion. |
Breathlessness | Intra-abdominal pressure | Reduce fill volume. Cross-flow dialysis. |
PD fluid migrating into chest via a diaphragmatic hole | Drain fluid, stop PD and convert to an alternative therapy. |
Continuous Venous-Venous Filtration and Haemodialysis (CVVH and CVVHDF) (Figure 6.2 and Figure 6.3)
Kinetic Principles
Haemofiltration is the removal of plasma water by filtration of the blood. Blood runs through the filter which has fibres made of semi-permeable membranes.
Figure 6.2 Continuous venous-venous haemofiltration (CVVH).
Based on 2005 Prismaflex manual, with kind permission from HospalGambro.
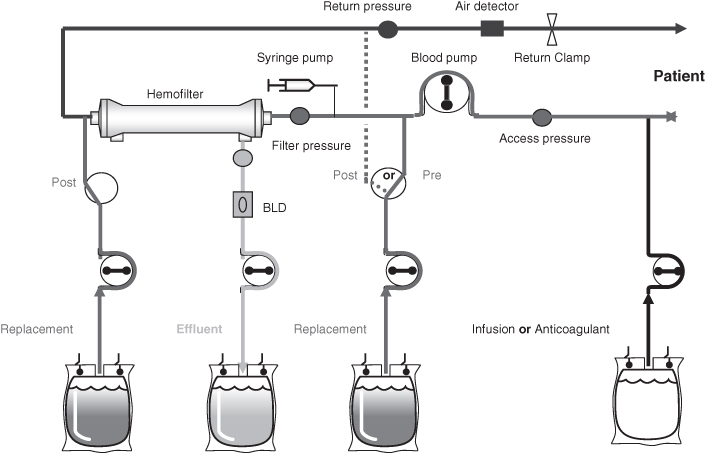
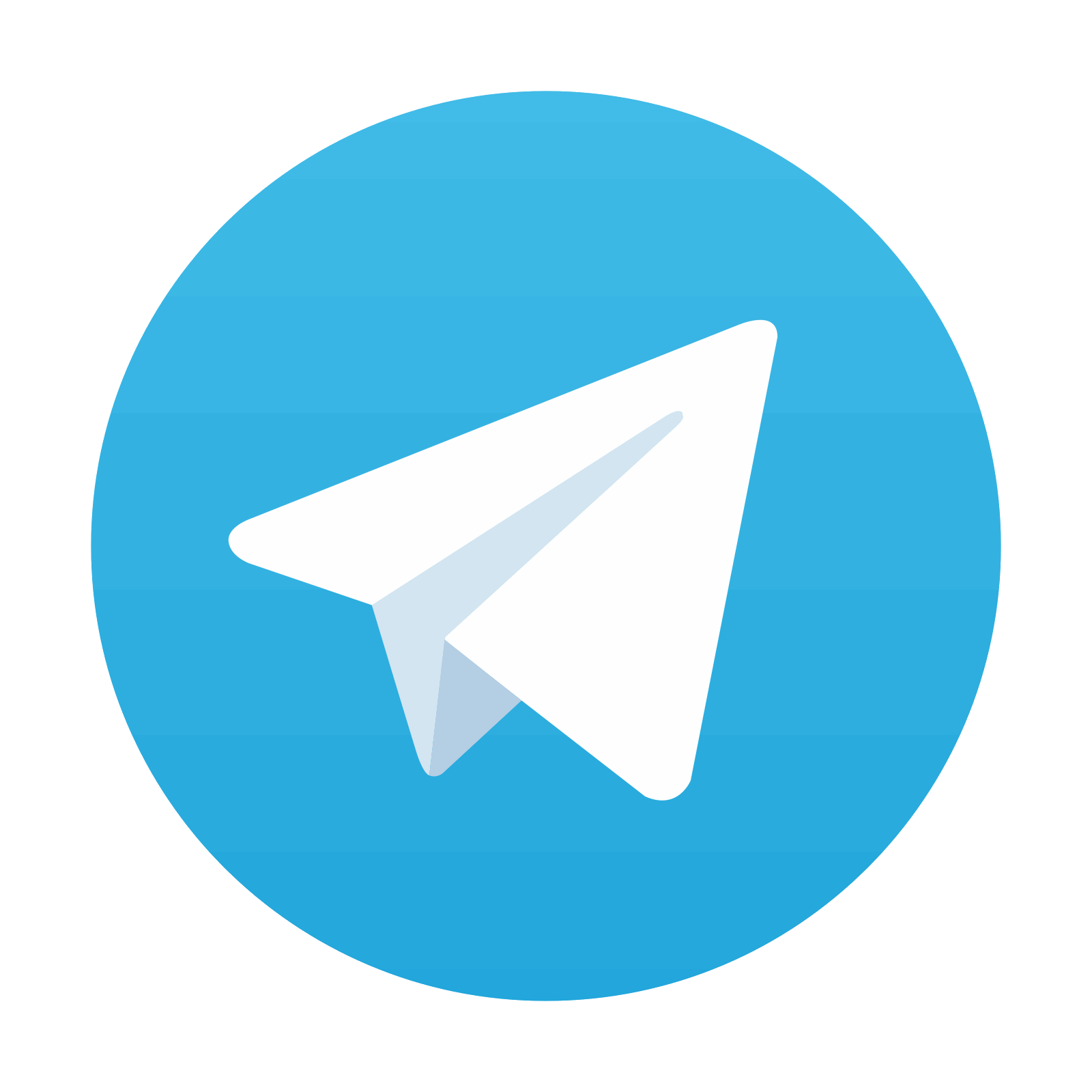
Stay updated, free articles. Join our Telegram channel

Full access? Get Clinical Tree
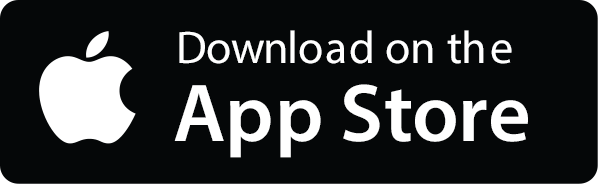
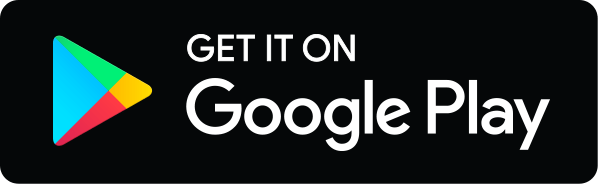