Chapter 29 Assessment of the Respiratory System
Health Promotion and Maintenance
1. Encourage all people to use masks and adequate ventilation when engaging in activities that expose them to inhalation irritants and toxins.
2. Teach patients and family members about what to expect during tests and procedures to assess respiratory function and respiratory disease.
3. Assess the patient’s endurance in performing ADLs.
4. Use concepts of anatomy and appropriate psychomotor skills to apply respiratory assessment techniques correctly.
5. Perform a clinical respiratory assessment, including health history, genetic risk, physical assessment, and psychosocial assessment.
6. Distinguish between normal and abnormal (adventitious) breath sounds.
7. Explain the respiratory changes associated with aging.
8. Calculate the pack-year smoking history for the patient who smokes or who has ever smoked cigarettes.
9. Interpret arterial blood gas values to assess the patient’s respiratory status.
10. Explain nursing care needs for the patient after bronchoscopy or open lung biopsy.
http://evolve.elsevier.com/Iggy/
Animation: Patterns of Respiration
Animation: Percussion Tones Throughout the Chest
Animation: Pulmonary Circulation
Answer Key for NCLEX Examination Challenges and Decision-Making Challenges
Audio Clip: Bronchial Breath Sounds
Audio Clip: Bronchovesicular Breath Sounds
Audio Clip: High- and Low-Pitched Crackles
Audio Clip: High- and Low-Pitched Wheezes
Audio Clip: Pleural Friction Rub
Audio Clip: Vesicular Breath Sounds
Review Questions for the NCLEX® Examination
Video Clip: Diaphragmatic Excursion
Video Clip: Inspection of the Nose
Video Clip: Percussion: Anterior Thorax
Video Clip: Respiratory Excursion
All cells need oxygen (O2) to live and perform their specific jobs. Oxygen intake depends on the respiratory system (Fig. 29-1). The respiratory system is important for oxygenation and tissue perfusion because the source of the oxygen for all body cells is the air we breathe. This system includes the upper airways, lungs, lower airways, and alveolar air sacs. Air with oxygen enters the nose and mouth and moves through the airways or respiratory tubes (trachea, bronchi, bronchioles) and into the air sacs (alveoli) of the lungs. Once in the air sacs, the oxygen from the air moves into the blood so that it can be carried to all tissues and organs. Carbon dioxide (CO2), the waste gas created in the tissues, moves from the blood into the lungs so it can be exhaled. All systems depend on adequate oxygen intake for tissue perfusion with oxygen. Any respiratory problem affects total body health and well-being.
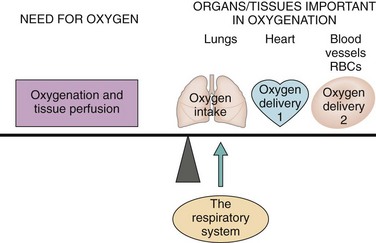
FIG. 29-1 Role of the respiratory system in oxygenation and tissue perfusion. RBCs, Red blood cells.
Lung and breathing problems are common and are the fifth leading cause of death in North America (McCance et al., 2010). Some respiratory problems are chronic, and the patient has physical and lifestyle limitations. Many acute health problems, medical therapies, and surgeries adversely affect respiratory function temporarily or permanently.
Anatomy and Physiology Review
The purposes of breathing are (1) to provide oxygen for tissue perfusion so that cells have enough oxygen to metabolize and generate energy and (2) to remove carbon dioxide, the waste product of metabolism. The respiratory system also influences acid-base balance, speech, sense of smell, fluid balance, and temperature control. The lungs are also an excretory organ because they can also break down some toxins and eliminate them from the body during exhalation.
Upper Respiratory Tract
The upper airways consist of the nose, the sinuses, the pharynx, and the larynx (Fig. 29-2).
Nose and Sinuses
The nose is the organ of smell, with receptors from cranial nerve I (olfactory) located in the upper areas. This organ is rigid and contains two passages separated by the septum. The upper nose is composed of bone; the lower two thirds is composed of cartilage, allowing some movement. The septum and interior walls of the nasal cavity are lined with mucous membranes that have a rich blood supply. The anterior nares (external openings into the nasal cavities) are lined with skin and hair, which help keep particles or organisms from entering the lungs. The posterior nares are openings from the nasal cavity into the throat.
The turbinates are three bones that protrude into the nasal cavities from the internal portion of the nose (see Fig. 29-2). Turbinates increase the total surface area for filtering, heating, and humidifying inspired air before it passes into the nasopharynx. Inspired air entering the nose is first filtered in the nares. Particles not filtered out in the nares are trapped in the mucous layer. These particles are moved by cilia (hairlike projections) to the throat, where they are either swallowed or expectorated. Inspired air is humidified by the mucous membrane and is warmed by heat from the vascular network.
The paranasal sinuses are air-filled cavities within the bones that surround the nasal passages (Fig. 29-3). Lined with ciliated membrane, the purposes of the sinuses are to provide resonance during speech and to decrease the weight of the skull.
Pharynx
The pharynx, or throat, is a passageway for both the respiratory and digestive tracts. It is located behind the oral and nasal cavities. The throat is divided into the nasopharynx, the oropharynx, and the laryngopharynx (see Fig. 29-2).
The oropharynx is located behind the mouth, below the nasopharynx. It extends from the soft palate to the base of the tongue and is used for breathing and swallowing. The palatine tonsils, which are part of the immune system, are located on the side borders of the oropharynx. They guard the body against invading organisms.
The laryngopharynx is the area located behind the larynx, extending from the base of the tongue to the esophagus. It is the critical dividing point where foods and fluids are separated from air. At this point, the passageway divides into the larynx and the esophagus.
Larynx
The larynx is the “voice box” and is located above the trachea, just below the throat at the base of the tongue. It is controlled by the recurrent laryngeal nerves. The larynx is composed of several cartilages (Fig. 29-4). The thyroid cartilage is the largest and is commonly called the “Adam’s apple.” The cricoid cartilage, which contains the vocal cords, lies below the thyroid cartilage. The cricothyroid membrane is located below the level of the vocal cords and joins the thyroid and cricoid cartilages. This site is used in an emergency for access to the lower airways. In this procedure, called a cricothyroidotomy, an opening is made between the thyroid and cricoid cartilage and results in a tracheostomy. The two arytenoid cartilages work with the thyroid cartilage in vocal cord movement.
Inside the larynx are two pairs of vocal cords: the false vocal cords and the true vocal cords. The glottis is the opening between the true vocal cords (Fig. 29-5). The epiglottis is a leaf-shaped, elastic structure that is attached along one edge to the top of the larynx. Its hingelike action prevents food from entering the trachea (aspiration) by closing over the glottis during swallowing. The epiglottis opens during breathing and coughing.
Lower Respiratory Tract
Airways
The lower airways are the trachea; two mainstem bronchi; lobar, segmental, and subsegmental bronchi; bronchioles; alveolar ducts; and alveoli (Fig. 29-6). The lower respiratory tract (tracheobronchial tree) is an inverted treelike structure consisting of muscle, cartilage, and elastic tissues. This system of branching tubes, which decrease in size from the trachea to the respiratory bronchioles, allows gases to move to and from the lungs. Gas exchange takes place in the lung tissue between the alveoli and the lung capillaries, not in the airways.
The trachea (windpipe) is located in front of the esophagus. It begins at the lower edge of the cricoid cartilage of the larynx and extends to the level of the fourth or fifth thoracic vertebra. The trachea branches into the right and left mainstem bronchi at a junction called the carina. The trachea contains 6 to 10 C-shaped rings of cartilage. The open portion of the C is the back portion of the trachea and contains smooth muscle that is shared with the esophagus.
The mainstem bronchi, or primary bronchi, begin at the carina. The bronchus is similar in structure to the trachea. The right bronchus is slightly wider, shorter, and more vertical than the left bronchus. Because of these differences, the right bronchus can be accidentally intubated when an endotracheal tube is passed. Also, when a foreign object is aspirated from the throat, it usually enters the right bronchus.
The mainstem bronchi branch into the secondary (lobar) bronchi that enter each of the five lobes of the two lungs. Each lobar bronchus branches into progressively smaller divisions. The cartilage of these lobar bronchi is ring-shaped and resists collapse. The bronchi are lined with a ciliated, mucus-secreting membrane. The cilia move mucus up and away from the lower airway to the trachea, where the mucus is either spit out or swallowed.
The bronchioles branch from the secondary bronchi and divide into smaller and smaller tubes, which are the terminal and respiratory bronchioles (Fig. 29-7). These tubes have a small diameter, have no cartilage, and depend entirely on the elastic recoil of the lung to remain open.
Alveolar ducts with alveoli attached branch from the respiratory bronchioles and resemble a bunch of grapes. Alveolar sacs arise from these ducts. The alveolar sacs contain groups of alveoli, which are the basic units of gas exchange (see Fig. 29-7). A pair of healthy adult lungs has about 290 million alveoli, which are surrounded by lung capillaries. These numerous small alveoli share common walls, making a large surface area for gas exchange. In a healthy adult, this surface area is about the size of a tennis court. Acinus is a term for the structural unit consisting of a respiratory bronchiole, an alveolar duct, and an alveolar sac.
Lungs
The lungs are elastic, cone-shaped organs located in the pleural cavity in the chest. The apex (top) of each lung extends above the clavicle; the base (bottom) of each lung lies just above the diaphragm. The lungs are composed of millions of alveoli and their related ducts, bronchioles, and bronchi. The right lung, which is larger than the left, is divided into three lobes: upper, middle, and lower. The left lung, which is narrower and smaller than the right lung, is divided into only two lobes. About 60% to 65% of lung function occurs in the right lung. Any problem with the right lung interferes with oxygenation to a greater degree than a problem in the left lung.
The pleura is a continuous smooth membrane composed of two surfaces that totally enclose the lungs. The parietal pleura lines the inside of the chest cavity and the upper surface of the diaphragm. The visceral pleura covers the lung surfaces. These two surfaces are lubricated by a thin fluid produced by the cells lining the pleura. This fluid allows the surfaces to glide across each other smoothly and painlessly during breathing.
Blood flow in the lungs occurs through two separate systems: bronchial and pulmonary. The bronchial system carries the blood needed to meet the metabolic demands of the lungs. The bronchial arteries, which branch from the thoracic aorta, are part of the systemic circulation and do not participate in gas exchange.
The pulmonary circulation is a highly vascular capillary network. Oxygen-poor blood travels from the right ventricle of the heart into the pulmonary artery, which eventually branches into arterioles that form capillary networks. These capillaries are meshed around and through the alveoli—the site of gas exchange (see Fig. 29-7). Freshly oxygenated blood travels from the capillaries to the pulmonary veins and then to the left atrium. From the left atrium, oxygenated blood flows into the left ventricle, where it is pumped throughout the systemic circulation.
Accessory Muscles of Respiration
Breathing occurs through changes in the size of and pressure within the chest cavity. Contraction and relaxation of specific chest muscles (and the diaphragm) cause changes in the size and pressure of the chest cavity. Accessory muscles that help in this process include the scalene muscles, which lift the first two ribs; the sternocleidomastoid muscles, which raise the sternum; and the trapezius and pectoralis muscles, which fix the shoulders. At times, various back and abdominal muscles are used when the work of breathing is increased.
Oxygen Delivery and the Oxygen-Hemoglobin Dissociation Curve
Oxygen delivery to the tissues requires the binding of oxygen to hemoglobin in red blood cells. Each molecule of hemoglobin can bind four oxygen molecules, which fills or saturates all of its binding sites. In the healthy person, each red blood cell normally has hundreds of thousands of hemoglobin molecules. When blood passes through the lung alveoli, where oxygen concentration is the greatest, oxygen diffuses from the alveoli into red blood cells and binds to all those hemoglobin molecules. This oxygen-rich blood then goes to the left side of the heart and is pumped out into systemic circulation. In tissues away from the source of oxygen, hemoglobin unloads (dissociates) the oxygen molecules and delivers them to the tissues. Fig. 29-8 shows the oxygen-hemoglobin dissociation curve.
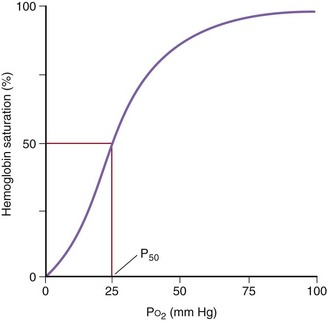
FIG. 29-8 The oxygen-hemoglobin dissociation curve. P50, the partial pressure of O2 at which hemoglobin is 50% saturated; PO2, oxygen partial pressure.
Tissue oxygen delivery through dissociation or unloading from hemoglobin is based on tissues’ need for oxygen. The curve in Fig. 29-8 shows that the rate of this unloading changes depending on how much oxygen is already in the tissues. When blood perfuses tissues in which the oxygen levels are high, as indicated in the upper right-hand corner of the figure, hemoglobin binds oxygen very tightly and little oxygen is unloaded or dissociated from the hemoglobin into the tissues. This prevents oxygen delivery from being wasted by unloading it where it is not needed. When blood perfuses tissues in which oxygen levels are very low, as indicated in the lower left-hand corner of Fig. 29-8, hemoglobin binds oxygen less tightly and will rapidly and easily unload its remaining oxygen to provide these tissues with needed oxygen. So, how rapidly and easily hemoglobin dissociates oxygen to the tissues changes depending on oxygen need. The S-shaped curve indicates that it is harder for oxygen to dissociate from hemoglobin in tissues that are well-oxygenated and much easier in tissues that are “starving” for oxygen.
The curve in Fig. 29-8 indicates that, on average, 50% of hemoglobin molecules have completely dissociated their oxygen molecules when blood perfuses tissues that have an oxygen tension (concentration) of 26 mm Hg. This is considered a “normal” point at which 50% of hemoglobin molecules are no longer saturated with oxygen.
On the other hand, when tissues have less need of oxygen because they are metabolizing more slowly than usual, the oxygen-hemoglobin dissociation curve shifts to the left, which means that the hemoglobin dissociates less easily. The tissue oxygen tension level has to be lower for hemoglobin to unload oxygen. Tissue conditions that cause a shift to the left include decreased tissue temperatures, decreased carbon dioxide levels, decreased amounts of glucose breakdown products, and a higher tissue pH (alkalosis). This also is a tissue protective mechanism to prevent wasting oxygen delivery to tissues that are not using the oxygen they already have.
One clinical example of how these actions are helpful is one in which a person is having a myocardial infarction (heart attack). Blood flow to the area is reduced and the heart muscle is metabolizing under hypoxic conditions, which create more carbon dioxide in the tissue and acidosis. As a result, the hemoglobin that does reach this hypoxic tissue unloads more oxygen at a faster rate to prevent ischemia and cardiac muscle cell death. What if this person believes he or she is having indigestion, which can have similar symptoms of chest discomfort, and tries to correct the problem by taking in large amounts of bicarbonate-based antacids? This ingestion increases the pH in the blood and all tissues, shifting the oxygen-hemoglobin dissociation curve to the left. As a result, the hypoxic cardiac muscle cells receive even less oxygen and more of them die.
Respiratory Changes Associated With Aging
The respiratory changes that occur with aging are described in Chart 29-1. Many additional respiratory changes in older patients result from heredity and a lifetime of exposure to environmental pollutants (e.g., cigarette smoke, bacteria, industrial fumes and irritants).
Chart 29-1 Nursing Focus on the Older Adult
Changes in the Respiratory System Related to Aging
PHYSIOLOGIC CHANGE | NURSING INTERVENTIONS | RATIONALES |
---|---|---|
Alveoli | ||
Alveolar surface area decreases.Diffusion capacity decreases.Elastic recoil decreases.Bronchioles and alveolar ducts dilate.Ability to cough decreases.Airways close early. | Encourage vigorous pulmonary hygiene (i.e., encourage patient to turn, cough, and deep breathe), and to use incentive spirometry, especially if he or she is confined to bed or has had surgery. | Potential for mechanical or infectious respiratory complications is increased in these situations. |
Encourage upright position. | The upright position minimizes ventilation-perfusion mismatching. | |
Lungs | ||
Residual volume increases.Vital capacity decreases.Efficiency of oxygen and carbon dioxide exchange decreases.Elasticity decreases. | Include inspection, palpation, percussion, and auscultation in lung assessments. | Inspection, palpation, percussion, and auscultation are needed to detect normal age-related changes. |
Help patient actively maintain health and fitness. | Health and fitness help keep losses in respiratory functioning to a minimum. | |
Assess patient’s respirations for abnormal breathing patterns. | Periodic breathing patterns (e.g., Cheyne-Stokes) can occur. | |
Encourage frequent oral hygiene. | Oral hygiene aids in the removal of secretions. | |
Pharynx and Larynx | ||
Muscles atrophy.Vocal cords become slack.Laryngeal muscles lose elasticity, and airways lose cartilage. | Have face-to-face conversations with patient when possible. | Patient’s voice may be soft and difficult to understand. |
Pulmonary Vasculature | ||
Vascular resistance to blood flow through pulmonary vascular system increases.Pulmonary capillary blood volume decreases.Risk for hypoxia increases. | Assess patient’s level of consciousness and cognition. | Patient can become confused during acute respiratory conditions. |
Exercise Tolerance | ||
Body’s response to hypoxia and hypercarbia decreases. | Assess for subtle manifestations of hypoxia. | Early assessment helps prevent complications. |
Muscle Strength | ||
Respiratory muscle strength, especially the diaphragm and the intercostals, decreases. | Encourage pulmonary hygiene, and help patient actively maintain health and fitness. | Regular pulmonary hygiene and overall fitness help maintain maximal functioning of the respiratory system and prevent illness. |
Susceptibility to Infection | ||
Effectiveness of the cilia decreases.Immunoglobulin A decreases.Alveolar macrophages are altered. | Encourage pulmonary hygiene, and help patient actively maintain health and fitness. | Regular pulmonary hygiene and overall fitness help maintain maximal functioning of the respiratory system and prevent illness. |
Chest Wall | ||
Anteroposterior diameter increases.Thorax becomes shorter.Progressive kyphoscoliosis occurs.Chest wall compliance (elasticity) decreases.Mobility may decrease. | Discuss the normal changes of aging. | Patients may be anxious because they must work harder to breathe. |
Discuss the need for increased rest periods during exercise. | Older patients have less tolerance for exercise. | |
Osteoporosis is possible. | Encourage adequate calcium intake (especially during a woman’s premenopause phase). | Calcium intake helps prevent osteoporosis by building bone in younger patients. |
Respiratory disease is a major cause of illness and chronic disability in older patients. Although respiratory function normally declines with age, there is usually no problem keeping pace with the demands of ordinary activity. The sedentary older adult, however, often feels breathless during exercise (Touhy & Jett, 2010).
Assessment methods
Patient History
Obtaining accurate information from the patient is important for identifying the type and severity of breathing problems. Chart 29-2 lists questions to ask patients (based on Gordon’s Functional Health Patterns) to assess the impact of pulmonary function.
Respiratory Assessment
Using Gordon’s Functional Health Patterns
Activity-Exercise Pattern
• Do you feel you have sufficient energy to perform tasks or routines that are required of you?
• Do you feel you have sufficient energy to do what you would like to do?
• Do you exercise? How often? For how long each time? What type(s) of exercise do you perform?
• What activities do you perform in your spare time?
• What is your ability to perform these tasks?
Based on Gordon, M. (2011). Manual of nursing diagnosis (12th ed.). Boston: Jones & Bartlett.
Age, gender, and race can affect the physical and diagnostic findings related to breathing. Many of the diagnostic studies for respiratory disorders (e.g., pulmonary function tests) use these data for determining predicted normal values.
Women, especially smokers, have greater bronchial responsiveness (i.e., bronchial hyperreactivity) and larger airways than men. This factor increases the risk for a more rapid decline in lung function as a woman ages, especially in women who were or are smokers.
Compared with whites, black people and others with dark skin usually show a lower oxygen saturation (3% to 5% lower) as measured by pulse oximetry; this results from deeper coloration of the nail bed and does not reflect true oxygen status.
Explore the home, community, and workplace for environmental factors that could cause or worsen lung disease. Occupational lung diseases include pneumoconiosis, which results from the inhalation of dust (e.g., coal dust, stone dust, silicone dust); toxic lung injury; and hypersensitivity disease (e.g., hypersensitivity to latex). Work history includes the exact dates of employment and a brief job description. Exposure to industrial dusts of any type or to the chemicals found in smoke and fumes may cause breathing disorders. Bakers, coal miners, stone masons, cotton handlers, woodworkers, welders, potters, plastic and rubber manufacturers, printers, asbestos workers, farm workers, and steel foundry workers are at risk for breathing problems. Use this opportunity to teach patients about the use of masks and adequate ventilation to protect the respiratory system from inhalation irritants.
Ask about home conditions, such as the type of heat used (e.g., gas heater, wood-burning stove, fireplace, kerosene heater). Determine exposure to irritants (e.g., fumes, chemicals, animals, birds, air pollutants). Ask about hobbies such as painting, working with ceramics, building model airplanes, refinishing furniture, or woodworking, which may expose the patient to harmful chemicals. Teach patients to wear masks during these exposures and to ensure the area is well ventilated.
Ask patients about their respiratory history (Table 29-1), including smoking history, drug use, travel, and area of residence.
TABLE 29-1 IMPORTANT ASPECTS TO ASSESS IN A RESPIRATORY SYSTEM HISTORY