CHAPTER 10. Anatomy and Physiology Related to Infusion Therapy
Lynn C. Hadaway, MEd, RN, BC, CRNI®
Integument and Connective Tissue, 139
Neurological System, 144
The Thoracic Cavity, 147
Peripheral Vascular System, 157
Systemic Blood Flow, 166
Mechanisms of Defense, 172
Summary, 175
Knowledge of the anatomy and physiology of the skin, peripheral vasculature, and cardiopulmonary and neurological systems is crucial for the safe administration of any parenteral therapy. Many factors related to these body functions can encumber or increase the success of infusions. Conversely, parenteral therapy administration can have a detrimental effect on the anatomy and physiological function of all body systems. Our goal of this chapter is to consider all pertinent factors in an effort to manage the risk of parenteral therapy and ensure a positive outcome for the patient.
When an IV device is inserted, the skin is the first organ affected. Skin serves several functions: it is a mechanical barrier against microorganisms and radiation, provides sensory and temperature regulation, and helps maintain fluid and electrolyte balance. Breaking this natural barrier increases the risk of infection. The use of antiseptic solutions, stabilization devices, and dressing materials can affect the skin’s natural composition of bacteria, oils, and sweat. Factors such as age, chronic disease, and the environment can produce changes in the skin, making entry through the skin a difficult process.
Vascular anatomy is of primary importance when locating and cannulating veins or arteries. Both the insertion site and the location of the catheter tip are important considerations. Extreme variations in pH, osmolarity, volume, and rate can alter the risk of complications if consideration is not given to the size of the vessel lumen and the amount and type of blood flow. Fluid volume status and general cardiopulmonary condition can limit the volume and rate of infusion and lead to a negative patient outcome when not monitored closely.
The neurological system affects all other systems and can influence the success or failure of any parenteral therapy. The close proximity of peripheral veins and nerves leads to risk of nerve damage with routine venipuncture. The sensory receptors in the skin, the innervation of the walls of veins and arteries, the systemic responses evoked by emotion and pain, and the use of the epidural space for infusions necessitate knowledge of the central nervous system.
Finally, variation in personal technique used for insertion, infusion, injection, and withdrawal is another vital component affecting anatomy and physiology. The impact of differences in catheter types and components and solution composition can be evaluated and monitored more easily than the nuances of individual technique. A thorough working knowledge of the anatomy and physiology of multiple body systems, combined with a carefully crafted expertise, is necessary to ensure positive patient outcomes.
INTEGUMENT AND CONNECTIVE TISSUE
The integument, or skin, is a highly specialized organ that protects the body from its environment. It ranges in thickness from 1.5 to 4.0 millimeters (mm), with the thickest skin appearing on the palms of the hands and plantar aspect of the feet (Standring, 2005). Skin functions as a protective barrier between the body and environmental elements such as microbial invasion and mechanical, chemical, and ultraviolet radiation damage. Skin also synthesizes vitamin D, cytokines, and other growth factors; is under the influence of hormones; and assists with control of body temperature (Standring, 2005).
Skin is divided into two layers: the epidermis and the dermis. Lying immediately under the dermis is the hypodermis, which is composed of adipose tissue. This layer cushions and protects the underlying structures and acts as insulation for the body (Figure 10-1).
![]() |
FIGURE 10-1 Anatomy of the skin. A, Thick skin, found on surfaces of the palms and soles of the feet. B, Thin skin, found on most surface areas of the body. (From Thibodeau GA, Patton KT: Anatomy & physiology, ed 6, St Louis, 2007, Mosby.) |
Loose connective tissue lies under the skin and is composed of several types of fibers and cells. Its primary functions include providing structure and defense. Superficial veins for venipuncture lie in this layer of loose tissue.
STRUCTURE AND FUNCTION
Epidermis
The epidermis is composed of five layers of squamous cells (Figure 10-2). From deepest to most superficial, the basal layer is closest to the dermis and contains interlocking ridges to maintain the integrity of the two structures. The prickle cell layer (stratum spinosum) contains Langerhans cells and a few lymphocytes. The granular layer (stratum granulosum) provides the principle barrier to prevent penetration by gases or liquids. The clear layer is only present in hairless skin of the palm and soles of the feet. The cornified layer (stratum corneum) is the most superficial layer with depths ranging from a few cells in thin skin to more than 50 cells in normal skin (Standring, 2005). These layers represent different stages of the maturation process. Individual cells are constantly maturing, moving upward to the surface and being replaced by new cells from the base. Loss of skin cells is only perceptible when it is excessive, such as peeling or scaling associated with certain skin disorders. Stressful agents such as prolonged exposure to sunlight, habitual pressure, and abrasion cause thickening of the cornified layer and can be measured by ultrasound (Matsumura and Anathaswamy, 2002 and Lopez et al., 2004). This may explain difficult venipunctures in some patients, especially those who are manual laborers or others whose lifestyle involves excessive exposure to sunlight.
![]() |
FIGURE 10-2 Layers and features of the epidermis. (From Kierszenbaum A: Histology and cell biology: an introduction to pathology, ed 2, St Louis, 2007, Mosby.) |
Keratins, a large group of filament proteins in all epithelial cells, change as the cells mature and move upward through the layers. These proteins are a group of more than 50 distinct gene sequences in humans, and mutations can be the cause of several skin disorders (McLean, 2003).
Three other types of cells can be found in the epidermis: Langerhans cells, melanocytes, and Merkel cells. Langerhans cells are found in an extensive network throughout the epidermis. Their function is to detect antigens, react to inflammatory signals from the other keratinocytes, and then migrate to the nearest lymph nodes. Migration of Langerhans cells to lymph nodes has been demonstrated after mechanical tape stripping and exposure to antigens, but no such migration was seen after stripping and exposure to nonantigenic solutions. There is a growing body of information linking the loss of the skin’s barrier function to the development of atopic dermatitis and allergies (Callard and Harper, 2007).
Melanocytes contain melanin, the pigment that imparts color to the skin. Variation in color depends on the number and composition of melanocytes and on genetic, hormonal, and environmental factors. Melanin protects the skin from sun damage and is a scavenger for free radicals that can also cause cellular damage (Standring, 2005). Little is known about the physiology of Merkel cells, yet they are located close to sensory nerve endings in the epidermis and create and release numerous peptides (Boulais and Misery, 2007).
Dermis
The dermis, the thickest layer of the skin, is composed of dense connective tissue with varying amounts of elastin fibers, collagen fibers, blood, and lymphatic vessels. Collagen is the largest component of the dermis and provides mechanical or tensile strength. Sebaceous glands, sweat glands and ducts, hair follicles, and arrector pili muscles surrounding hair follicles are also located in the dermis (see Figure 10-1).
The dermis is divided into the papillary and reticular layers. The papillary layer is immediately under the epidermis and serves to anchor the epidermis mechanically and support it metabolically. Fingerlike projections, known as rete ridges, extend into the base of the epidermis to mate with the rete pegs in the epidermis and enhance the bond between the two layers. The reticular layer is the deepest and is characterized by dense, irregular, connective tissue. This type of connective tissue is formed by strong collagen fiber bundles arranged in a multilayered network, providing the ability to stretch. Elastin fibers are usually thinner and have almost perfect recoil.
The hair bulb, responsible for generating the growth of hair, is located in the dermis and is surrounded by the hair follicle. Arrector pili muscles extend at an angle from the follicle into the dermis. When these muscles are stimulated by cold or emotion, the hair shaft is pulled upward and the skin appears dimpled. Sebaceous glands are located in the angle between the muscle and follicle and the muscular action expresses sebum, the secretion from the sebaceous glands. Sebum passes through a duct, into the hair follicle, and onto the skin surface. The function of sebum, composed of glycerides, cholesterol, and wax esters, is to lubricate and protect the hair and skin and contribute to body odor.
Sweat glands and ducts arise from the dermis and communicate with the skin surface. The greatest number is located on the face and hands with the fewest number on the surfaces of the extremities. These glands secrete a clear, odorless, hypotonic fluid containing urea, lactate, immunoglobulin, and other proteins as well as ions such as sodium, chloride, calcium, and bicarbonate. Secretion is stimulated by temperature elevation and emotional stimulation, with as much as 10 L of sweat produced daily (Standring, 2005).
Superficial fascia or hypodermis
Continuing downward to the next layer, the superficial fascia is a layer of loose connective tissue (see Figure 10-1). This layer contains the same collagen and elastin fibers as the dermis. Adipose tissue or fat cells are located in this layer, where they serve as energy stores and thermal insulation. A large variety of defensive cells similar to those found in the blood are found in this tissue. Among all these important components lie the superficial veins used for peripheral venipuncture.
Blood vessels, lymphatics, and nerves
The skin does not have a great metabolic demand, yet the amount of blood flow far exceeds nutritional requirements and is equal to approximately 5% of cardiac output (Standring, 2005). Blood is supplied to the skin by a direct cutaneous network of vessels arising from the muscles and superficial fascia (Figure 10-3). Arterioles supply sweat and sebaceous glands and hair follicles; capillaries loop upward to the base of the epidermis through the rete ridges; and venules pass back through the dermis to join small veins in the superficial fascia. The skin is also served by a large number of small lymphatic vessels beginning in the papillary dermis. There are numerous anastomoses at all levels that are responsible for moving lymphocytes, Langerhans cells, and macrophages to lymph nodes (Standring, 2005).
![]() |
FIGURE 10-3 Vascular supply to the skin. (From Mathes SJ, Hentz VR: Plastic surgery, ed 2, Philadelphia, 2006, Saunders.) |
Cutaneous circulation serves as a thermoregulator. Blood flow to the skin is 10 times greater than its nutritional requirements. In response to the need for heat loss or conservation, cutaneous blood flow can be increased or decreased by 20 times the normal capacity. Cold conditions can quickly cause vasoconstriction, making venipuncture difficult, yet heat application rapidly causes vasodilation (Standring, 2005).
The extensive nerve supply in the skin acts as a sensory organ and regulator of response to mechanical, thermal, painful, or noxious stimuli. Sensory receptors in the form of free nerve endings provide needed information about the external environment. Encapsulated mechanoreceptors are found in the deep dermal layer and respond to weight, pressure, and vibration.
AGE-RELATED CHANGES
The normal physiological process of aging can have a distinct effect on the appearance and performance of the skin. An environmental source of skin changes is habitual sun exposure, called photoaging, and is considered to be preventable damage (Matsumura and Anathaswamy, 2002).
Changes in the appearance of skin attributed to age include wrinkling, dryness, and looseness. These effects can be found in all layers and cells of the skin (Table 10-1). Such changes indicate that the skin has a continually decreasing capability to respond to forces and strain, becoming more rigid and inflexible. The common clinical picture of these problems as related to parenteral therapy includes bleeding from venipuncture sites, shearing of skin layers when tape or dressing materials are removed, and excessive skin dryness with the use of alcohol and other antiseptic agents.
Location | Changes |
---|---|
Epidermis | Flattening of projections between the two layers; widening space between keratinocytes; slower maturation of keratinocytes; decreased thickness of layer |
Dermis | Decreased thickness of layer; thicker and calcified elastin fibers, sometimes with complete fragmentation; decrease in total amount of collagen; reduction in vascular network around hair follicles and glands; decreased number of blood vessels and shorter capillary loops; decreased amount of mucopolysaccharides, resulting in changes in turgor; decreased number of nerve endings; decreased number of sweat glands and sweat production |
Cells | Decreased number of melanocytes, resulting in decreased protection from ultraviolet light; decreased number of mast cells and reduction in histamine release |
Photoaging from exposure to the sun differs with the length of exposure, gender, and individual differences in the skin. However, the same problems are seen as those that occur with the normal aging process.
ROLE IN INFECTION PREVENTION
Normal resident flora on human skin includes four bacterial groups and a fungal group. Coryneforms and staphylococci are the major bacterial groups, with Micrococcus and Acinetobacter as the minor bacterial group. Acinetobacter species can easily be found in the antecubital fossa. Malassezia is the primary fungus found on skin. Microorganisms are found in pilosebaceous ducts and on the skin surface in microcolonies. These colonies are larger in men than in women and are found in different quantities depending on the type of skin. For instance, the dry skin of the forearm may have only 100 organisms per colony, whereas the shoulder area may have colonies containing 10 5 organisms (Matsumura and Anathaswamy, 2002). Factors affecting the growth of skin microorganisms include the amount and type of nutrients such as lipids, adherence properties of the flora, and humidity at the skin surface. Presence of water at the skin surface leads to rapid microbial growth in short periods. The three most important factors in infection prevention are immunocompetence, nutritional status, and the maintenance of an intact integument. Therefore careful attention must be given to the condition of the skin under dressings, the patient’s response to various antiseptic solutions and dressing materials, and the number of venous, arterial, or epidural cannulations with the necessary break in the skin.
The skin’s primary function in the prevention of infection is as a mechanical barrier to the invasion of pathogenic organisms. Chemical barriers include sebaceous secretions that have antibacterial and antifungal fatty acids; the role of circulating immunoglobulins, cellular immunity, and delayed hypersensitivity; and the acidic pH of the skin (usually between 3 and 5) (Matsumura and Anathaswamy, 2002). The inflammatory process and subsequent infections are influenced by these factors.
WOUND HEALING
Because initiating any parenteral therapy requires breaking the skin, it is appropriate to consider the process for the healing of that wound. The anatomy of skin has been presented from the outer surface inward. However, to understand the process of restoring the dermis and epidermis after injury, it is necessary to discuss the repair of the dermis and the regeneration of the epidermis.
Immediately after any wound is sustained, the initial phase is one of inflammation in response to stimulation of platelets and mast cells (Figure 10-4). Bleeding is controlled by activation of the complex clotting cascade and the activation of platelets. The permeability of local blood vessels increases and causes plasma proteins to leak into the wound, forming an extravascular clot. Neutrophils, which control bacteria, and monocytes enter the wound. Monocytes become macrophages and have a phagocytic action; in addition, they release chemicals necessary for granulation.
![]() |
FIGURE 10-4 Normal response of skin to incision. (From Standring S, editor: Gray’s anatomy, ed 39, London, 2005, Churchill Livingstone.) |
The second phase, proliferation or granulation, is characterized by the formation of a large number of capillaries through the process of angiogenesis. These are embedded in a thick matrix of fibronectin, a glycoprotein that acts like glue. Macrophages are present in this stage and act to debride the wound. Collagen is synthesized, which adds more strength to the tissue.
Within a few hours after injury, the epidermis begins to regenerate new epithelial cells. Keratinocytes use a “leapfrog” action, moving over each other to reach the bed of the wound. They then stop moving and begin to divide, forming new epidermal cells. This process seals and protects the healing wound and severs the connection between the underlying clot and wound surface.
Two factors may inhibit the healing process at this point. The presence of anti-inflammatory steroids prevents macrophages from entering the wound, thus slowing the formation of granulation tissue. A lack of ascorbic acid (vitamin C) can interfere with the production of collagen.
The final stage of dermal repair is remodeling. Granulation tissue, containing a large number of cells and blood vessels, is gradually replaced with scar tissue, which contains fewer cells and vessels. Fibronectin is removed and replaced with randomly crosslinked collagen fibers. Over time these fibers become more organized, and the tensile strength of the new tissue improves, although it may not regain its original tensile strength (Standring, 2005).
Healing of wounds is delayed when there is excessive or prolonged bleeding during the initial injury. Red blood cells and fibrin must be removed before the repair begins. Lack of oxygen in the tissue causes vasoconstriction and decreases the deposit of cells necessary for the inflammatory process. Improper nutrition and underlying diseases such as diabetes also slow wound healing.
NEUROLOGICAL SYSTEM
The human nervous system acts as an information loop containing billions of neurons and hundreds of thousands of synaptic connections. When changes in the environment are picked up by the sensory organs of the body, information is fed back to the controlling organ, or brain, which coordinates this with numerous other pieces of information, decides what response to make, and then communicates that response back to the body.
The system can be studied in many ways. Distinct functional divisions of the nervous system include the following: (1) the sensory ability to transmit information from tactile, visual, and auditory receptors; (2) the motor functions, controlling all skeletal and smooth muscles; and (3) the autonomic system, which controls glands and smooth muscles. Anatomic divisions are the central system, composed of the brain and spinal cord, and the peripheral system, composed of 12 cranial and 31 spinal nerves.
INFORMATION PROCESSING
The nervous system processes enormous amounts of information in a manner that produces correct responses. While much sensory information (such as how clothing contacts the body) is unimportant, significant information is channeled and processed to produce the desired response.
Information travels from one neuron to the next through two types of synapses (Figure 10-5). Chemical synapses, the type found in most human central nervous system tissue, secrete a neurotransmitter. There are more than 40 neurotransmitters but the most common ones are acetylcholine, norepinephrine, epinephrine, histamine, γ-aminobutyric acid, glycine, serotonin, and glutamate (Guyton and Hall, 2006). Electrical synapses conduct electricity through open fluid channels between nerve cells. Chemical synapses send signals in only one direction, from the presynaptic neuron to the postsynaptic neuron, but electrical synapses transmit in either direction.
![]() |
FIGURE 10-5 Structure of a typical neuron. (From Standring S, editor: Gray’s anatomy, ed 39, London, 2005, Churchill Livingstone.) |
Chemical neurotransmitters can be divided into two groups. Small-molecule, rapidly acting transmitters are released within a millisecond or less, producing the acute responses of the nervous system. Neuropeptides are slow-acting transmitters, such as hormones, that act in the hypothalamus and pituitary glands, the gastrointestinal tract, or brain. Examples of neuropeptides are vasopressin, oxytocin, insulin, glucagon, angiotensin II, bradykinin, and substance P (Guyton and Hall, 2006).
Synaptic transmission is affected by special factors. Fatigue can occur when the firing rate is very rapid at first, and then becomes progressively slower over the next few seconds. Serum pH and low oxygen levels also produce changes. Acidosis and hypoxia decrease synaptic transmission, while alkalosis increases the excitability of neurons (Guyton and Hall, 2006).
FUNCTIONAL DIVISIONS
Sensory receptors
There are five types of sensory receptors (mechanoreceptors, thermoreceptors, nociceptors, electromagnetic receptors, and chemoreceptors), with corresponding stimuli for each (Table 10-2). Except for electromagnetic receptors, all have an effect on parenteral therapy. Many types of stimulation, such as heat, light, cold, pressure, and sound, must be processed appropriately. All these variations in modalities of sensation are transmitted along different afferent fibers, ending at a specific point in the central nervous system. This is called the labeled line principle (Guyton and Hall, 2006), which means that pain receptors transmit a painful response whether it is caused by puncture, crushing, or electricity. The sensation of touch is transmitted along a single path, no matter how the stimulation occurs.
Type of receptor | Sensation | Effect of parenteral therapy |
---|---|---|
Mechanoreceptor | Skin tactile sensibilities | Palpation for veins and arteries; application of antiseptic solutions and dressings; removal of tape and dressings |
Deep tissue sensibilities | Puncture of vein or artery; tight or constricting dressing | |
Arterial pressure control through baroreceptor or pressure receptor system in all large arteries | Excessive infusion of intravenous solution, increasing circulating blood volume, and activating pressure receptors | |
Hearing | None | |
Equilibrium | None | |
Thermoreceptor | Cold, warmth | Application of heat or cold to treat phlebitis, infiltration, or extravasation |
Nociceptor | Pain | Puncture of vein or artery for insertion of any catheter; puncture of lumbar area for epidural catheter insertion; removal of dressings; infusion of irritating medications subcutaneously; application of extreme heat or cold |
Electromagnetic receptor | Vision | None |
Chemoreceptor | Decreased arterial pressure stimulates receptors in aorta and carotid arteries to respond to low oxygen levels and increased carbon dioxide levels | Inadequate amount of solution infused, resulting in decreased circulating blood volume |
Osmotic changes in blood | Infusion of extremely hypertonic or hypotonic solutions | |
Taste | Dysgeusia, or alteration in taste, produced by many IV medications | |
Smell | None |
The sensation of pain can have a profound impact on parenteral therapy. Pain can be felt quickly or slowly. Fast pain is felt within 0.1 second after stimuli, but slow pain takes seconds to be felt and may increase over minutes (Guyton and Hall, 2006). Pain receptors in skin, subcutaneous tissue, and vessel walls are free nerve endings. Fast pain is associated with skin punctures, cuts, or electrical shock. Slow pain is more intense, occurring over a prolonged period, and is usually associated with tissue destruction that can occur with catheter complications such as thrombophlebitis, infiltration and extravasation. Mechanical, thermal, and chemical stimuli can activate pain receptors. Fast pain is evoked primarily by mechanical and thermal means, whereas slow pain is associated with all three stimuli. Histamine, bradykinin, potassium ions, serotonin, acetylcholine, and proteolytic enzymes can stimulate pain receptors. Bradykinin and potassium ions appear to be responsible for producing pain following tissue damage. Pain from ischemia is probably related to the formation of large amounts of lactic acid produced from anaerobic metabolism (Guyton and Hall, 2006). Phlebitis, infiltration, and extravasation injuries produce the tissue damage involving these chemicals.
Motor function
Sensory information comes from all areas of the nervous system into the spinal cord, brain stem, and cerebrum where it produces muscular functions transmitted to the body along efferent nerve fibers. Specific areas of the cerebral cortex and brain stem control motor functions. The pyramidal tract (or corticospinal tract) is the principal pathway for the transmission of impulses from the motor cortex through the brain stem and down the spinal cord. Other pathways for impulses include the mesencephalon (midbrain) and the cerebellum. The term extrapyramidal motor system has been used clinically to describe those motor functions outside the pyramidal system. Because there are many functions and pathways included in this term, it is difficult to use it for physiological purposes. However, the term extrapyramidal side effects is used to describe uncoordinated motor movements of the neck, jaw, and extremities that are associated with the administration of some types of medications.
Autonomic nervous system
The autonomic nervous system regulates the body’s internal organs and controls such functions as glandular secretions, arterial blood pressure, sweating, and body temperature. Perhaps the most astonishing factor about this system is the speed with which changes occur. The heart rate can double within 3 to 5 seconds, the blood pressure can drop enough to cause fainting within 4 to 5 seconds, and sweating can occur within seconds (Guyton and Hall, 2006). The two major divisions of the autonomic nervous system are the sympathetic and parasympathetic, both of which send signals to specific organs (Figure 10-6). There is also a system of visceral or enteric reflexes that receives subconscious sensory signals from the viscera, sending back a reflex response to control that specific organ. The vagus nerves (cranial nerve X) contain about 75% of all parasympathetic nerve fibers, extending to the thoracic and abdominal areas of the body.
![]() |
FIGURE 10-6 Autonomic nervous system. (From Standring S, editor: Gray’s anatomy, ed 39, London, 2005, Churchill Livingstone.) |
Synaptic transmission involves primarily acetylcholine (known as cholinergic fibers) and norepinephrine (known as adrenergic fibers). Virtually all parasympathetic nerve endings secrete acetylcholine, and almost all sympathetic nerves secrete norepinephrine.
Sympathetic stimulation may cause excitation in some organs but has an inhibitory effect on others. The same can be said for the parasympathetic system. Also, the two systems may work in a reciprocal arrangement, with one causing excitation and the other producing inhibition. For example, sympathetic stimulation increases the rate and force of heart contraction, and parasympathetic stimulation has the opposite effect. Systemic blood vessels, especially in the skin of extremities, are constricted by sympathetic stimulation, thus increasing the difficulty of peripheral venipuncture. Parasympathetic stimulation has no effect on blood vessels except for those in the face that react in the form of a blush (Guyton and Hall, 2006). Table 10-3 provides a list of autonomic effects on various body organs.
Organ | Effect of sympathetic stimulation | Effect of parasympathetic stimulation |
---|---|---|
Eye | ||
Pupil | Dilated | Constricted |
Ciliary muscle | Slight relaxation (far vision) | Constricted (near vision) |
Glands | ||
Nasal Lacrimal Parotid Submandibular Gastric Pancreatic | Vasoconstriction and slight secretion | Stimulation of copious secretions (containing many enzymes for enzyme-secreting glands) |
Sweat | Copious sweating (cholinergic) | Sweating on palms of hands |
Apocrine | Thick, odoriferous secretion | None |
Vasculature | ||
Blood vessels | Most often constricted | Most often little or no effect |
Heart | ||
Muscle | Increased rate Increased force of contraction | Slowed rate Decreased force of contraction (especially of atria) |
Coronaries | Dilated (beta 2-adrenergic); constricted (alpha-adrenergic) | Dilated |
Lungs | ||
Bronchi | Dilated | Constricted |
Blood vessels | Mildly constricted | Dilated |
Gut | ||
Lumen | Decreased peristalsis and tone | Increased peristalsis and tone |
Sphincter | Increased tone (most times) | Relaxed (most times) |
Liver | Glucose released | Slight glycogen synthesis |
Gallbladder and bile ducts | Relaxed | Contracted |
Kidney | Decreased output and renin secretion | None |
Bladder/Genitourinary System | ||
Detrusor | Relaxed (slight) | Contracted |
Trigone | Contracted | Relaxed |
Penis | Ejaculation | Erection |
Systemic Arterioles | ||
Abdominal viscera | Constricted | None |
Muscle | Constricted (alpha-adrenergic) | None |
Dilated (beta 2-adrenergic) | ||
Dilated (cholinergic) | ||
Skin | Constricted | None |
Blood | ||
Coagulation | Increased | None |
Glucose | Increased | None |
Lipids | Increased | None |
Other | ||
Basal metabolism | Increased up to 100% | None |
Adrenal medullary secretion | Increased | None |
Mental activity | Increased | None |
Piloerector muscles | Contracted | None |
Skeletal muscle | Increased glycogenolysis | None |
Increased strength | ||
Fat cells | Lipolysis | None |
Numerous intravenous medications act on the autonomic nervous system. Adrenergic or sympathomimetic drugs stimulate organs that respond to the sympathetic system and include epinephrine, methoxamine, phenylephrine, isoproterenol, and albuterol. Other drugs block adrenergic activity, including reserpine, phentolamine, propranolol, and metoprolol. Parasympathomimetic drugs include pilocarpine and methacholine, while atropine and scopolamine block cholinergic activity.
ANATOMIC DIVISIONS
Central nervous system
The spinal cord extends from the medulla oblongata and occupies about two thirds of the length of the vertebral column. In most adults, the cord terminates between the first and second lumbar vertebrae. The cord is enclosed within three protective layers. The pia mater is the most proximal to the cord, the arachnoid mater is next, and the dura mater is the most distal. Between the pia and arachnoid mater is the subarachnoid space, which contains cerebrospinal fluid. The subdural space lies between the arachnoid and dura mater and does not contain fluid. The subdural space below the level of the cord can be used for the epidural infusion of medication, such as that used for pain management.
Peripheral nervous system
Twelve pairs of cranial nerves and 31 pairs of spinal nerves compose this system. Cranial nerves originate at the base of the brain and have four functions: motor, somatic sensory, special senses, and parasympathetic.
Of primary importance for parenteral therapy is the vagus nerve, which has the longest course of any cranial nerve. It descends behind the internal jugular vein, crosses the subclavian artery, and enters the thorax where it passes behind the brachiocephalic vein and superior vena cava (Figure 10-7). Numerous branches extend to the pharynx, larynx, heart, lungs, esophagus, stomach, liver, pancreas, spleen, small intestines, and kidneys. Six branches of the vagus nerve innervate the heart. Venipuncture of the internal jugular vein can result in damage to the vagus nerve (Nakayama et al, 1994).
![]() |
FIGURE 10-7 Dissection that displays the heart, great vessels, and lungs. (From Standring S, editor: Gray’s anatomy, ed 39, London, 2005, Churchill Livingstone.) |
The phrenic nerve arises from the cervical area of the neck and descends into the thorax where it serves as the motor nerve to the diaphragm. The right phrenic nerve follows the right brachiocephalic vein and superior vena cava while the left phrenic nerve lies close to the left subclavian artery, left brachiocephalic vein, and aortic arch (see Figure 10-7). Numerous reports link right phrenic nerve damage to both left- and right-sided insertions of central venous catheters. Paralysis of the diaphragm results, although shoulder pain is the most frequent initial symptom (Rigg et al., 1997, Aggarwal et al., 2000, Takasaki and Arai, 2001, Reeves and Anderson, 2002 and Mandala et al., 2004).
The brachial plexus arises from cervical and thoracic spinal nerves, passing posteriorly to the clavicle in close proximity to the subclavian artery and vein. There are two large branches of this plexus: the supraclavicular and infraclavicular (Figure 10-8). These branches bifurcate into smaller nerves that serve the muscles and cutaneous areas of the shoulder, chest, and upper and lower arm. The close proximity of this group of nerves to the puncture sites of the subclavian vein makes damage to the nerves and ultimately the areas served by that nerve a real possibility.
![]() |
FIGURE 10-8 Brachial plexus and its skeletal relations. (From Jacob SW, Francone CA: Elements of anatomy and physiology, ed 2, Philadelphia, 1989, Saunders.) |
The median, ulnar, and radial nerves are all branches of the brachial plexus. The median nerve passes laterally to the brachial artery and then crosses the artery, descending medially into the antecubital fossa and then into the forearm and palm of the hand. Branches of the median nerve can be superficial in the volar aspect or palm side of the wrist (Figure 10-9). Veins in this area appear to be good for cannulation, but venipuncture is usually painful in this area because of the close proximity of the nerve.
![]() |
FIGURE 10-9 Nerves of the left upper extremity (palmar view). (From Standring S, editor: Gray’s anatomy, ed 39, London, 2005, Churchill Livingstone.) |
Multiple intersections between the cephalic vein and the sensory branches of the radial nerve were found when researchers dissected the forearm and wrists of 33 cadavers at the origin of the cephalic vein (Figure 10-10) (Vialle et al, 2001). The researchers suggest that to avoid nerve damage from venipuncture in the cephalic vein of the lower forearm, the insertion site should be at least 12 centimeters (cm) above the level of the styloid process at the distal end of the radius. Other reports of peripheral nerve damage resulting from venipuncture include the medial and lateral antebrachial cutaneous nerves in the antecubital fossa (Rayegani and Azadi, 2007), the posterior interosseous nerve branching from the radial nerve in the antecubital fossa (Ragoowansi, Kirkpatrick, Moss, 1999), the anterior interosseous nerve branching from the median nerve at the antecubital fossa (Zubairy, 2002 and Puhaindran and Wong, 2003), and radial nerve injury in the wrist (Stahl et al., 1996, So et al., 1999 and Boeson et al., 2000).
![]() |
FIGURE 10-10 Radial aspect of the distal forearm and wrist.PART A: 1. Tendon of extensor carpi radialis brevis. 2. Tendon of extensor carpi radialis longus. 3. Tendon of extensor pollicis longus. 4. First metacarpal. 5. Trapezium. 6. Tendon of extensor pollicis brevis. 7. Scaphoid. 8. Tendon of abductor pollicis longus. 9. Radial styloid.PART B: 1. Cephalic vein. 2. Radial nerve. 3. Radial artery. (From Lumley JSP: Surface anatomy, ed 4, London, 2008, Churchill Livingstone.) |
In the lower extremity, the sacral plexus arises from the sacral vertebrae and descends the leg in much the same manner as the brachial plexus in the arm (Figure 10-11). Of particular importance is the medial plantar nerve, which passes on the medial aspect of the foot near the ankle. The saphenous nerve passes close to the saphenous vein on the anterior aspect of the foot. When the foot of an infant is used for venipuncture, with subsequent immobilization of the joint, the nerves can be damaged if adequate padding and range-of-motion exercises are not used.
![]() |
FIGURE 10-11 Nerves of the anterior and posterior aspects of the lower extremity. (From Drake RL, Vogl W, Mitchell AMW: Gray’s anatomy for students, London, 2005, Churchill Livingstone.) |
THE THORACIC CAVITY
The borders and boundaries of the thorax help determine the optimal locations for catheter insertion and catheter tip placement. The presence of any catheter and the solutions infused can affect the physiology of the heart and lungs, in addition to the anatomy of the vessels.
BONY THORAX
A framework of bone and cartilage surrounds the vital respiratory and circulatory structures of the thorax. The vertebrae of the spinal column form the posterior aspect. On the anterior aspect, the clavicles lie horizontally, connecting with the sternum to create the upper border.
The first rib curves from the first thoracic vertebra to the upper border of the sternum. This junction is difficult to palpate because the clavicle joins the sternum immediately in front of and superior to it (Figure 10-12). The costoclavicular ligament connects the first rib with the lower border of the clavicle close to the sternum. Motion of the sternoclavicular joint is involved with all shoulder movement. The first rib moves with respiration.
![]() |
FIGURE 10-12 Anterior view of the thorax, root of neck, and axilla. (From Standring S, editor: Gray’s anatomy, ed 39, London, 2005, Churchill Livingstone.) |
Subclavian vein cannulation in the medial aspect or close to the junction of the clavicle and first rib can have a negative outcome. Known as the pinch-off syndrome, the scissorlike action of these bones can sever the catheter, causing a catheter emboli (Pittiruti et al., 2000, Sarzo et al., 2004, de Graaff et al., 2006, Surov et al., 2006 and Nuss et al., 2008). Positioning the patient in the Trendelenburg position with a rolled towel or sheet between the scapulas helps open the angle of these bones. When the patient is moved from this position, the angle is closed. The minimal clinical problem is compression of the catheter, causing difficult infusion. Irritation to the tunica intima of the vein could compound the problem, with the possibility of development of a thrombosis. Using a more lateral venipuncture location, at the axillary vein, and then advancing into the central system can eliminate this problem.
The nerves and vascular structures in the thoracic outlet can be compressed by changes in normal anatomy caused by posture, physical activity, chronic illness, and occupation. Pain, muscle weakness and atrophy, and sensory changes result from these thoracic outlet syndromes. Arterial compression causes pallor of the fingers, while venous compression leads to edema and cyanosis. Venous cannulation can increase the risk of thrombosis in these patients (Urschel and Kourlis, 2007).
The kidney-shaped opening formed by the first rib, sternum, and vertebra is the thoracic inlet. It measures about 5 cm from front to back and about 10 cm from side to side, and is angled downward toward the anterior side (Standring, 2005). This small opening must accommodate many important structures, including the subclavian arteries and veins, carotid arteries, internal jugular vein, thymus gland and its muscles and nerves, trachea and esophagus, part of the brachial nerve plexus and vagus nerve, and the upper part of the pleura and apex of the lungs. Venous catheters inserted, terminating, or passing through the veins in this area could lead to complications affecting all these structures.
The sternum lies in the center of the anterior chest and is divided into three sections (Figure 10-13). Moving from top to bottom, the first section or manubrium is broad and thick and narrows toward the lower side. This section can be used for intraosseous infusion during emergencies in adults (Koschel, 2005). The midsection or body is long, narrow, and thin. The xiphoid process is the smallest section, extending into the epigastric region.
![]() |
FIGURE 10-13 The sternum and costal cartilages, anterior aspect. (From Standring S, editor: Gray’s anatomy, ed 39, London, 2005, Churchill Livingstone.) |
There are 12 pairs of ribs encircling the thorax from the vertebrae to the sternum (see Figure 10-13). The upper seven pairs, known as true ribs, connect to the sternum by costal cartilages. Thoracic mobility and elasticity are directly related to the structure of this costal cartilage found between the ribs and sternum. The remaining five ribs are called false ribs, with the eighth, ninth, and tenth ribs attaching to the costal cartilage immediately above it. The eleventh and twelfth ribs are floating ribs without any attachment on the anterior side.
RESPIRATORY ANATOMY
The next layer of the thorax consists of the pleurae, or sacs, that cover the lungs. There are two layers of pleurae. The parietal pleura lines the internal surface of the ribs and costal cartilage, vertebrae, diaphragm, mediastinum, and lung apices. The visceral pleura adheres closely to all lung surfaces. Both pleurae easily slide over each other with the space between them called the pleural cavity. The elastic recoil of the lungs is opposed by the outward pull of the chest wall, thus creating negative pressure in this pleural space. Trauma, such as puncture during the insertion of a central venous catheter, results in air, fluid, blood, or lymph fluid accumulating in this space.
The mediastinum separates the lungs and can be divided into four parts (Figure 10-14). The superior mediastinum contains the internal thoracic arteries and veins; brachiocephalic veins and upper half of the superior vena cava; aortic arch; brachiocephalic, left common carotid, and subclavian arteries; left superior intercostal vein; vagus, cardiac, phrenic, and left recurrent laryngeal nerves; and the trachea, esophagus, thoracic duct, and superficial part of the cardiac plexus.
![]() |
FIGURE 10-14 The major divisions of the mediastinum. (From Standring S, editor: Gray’s anatomy, ed 39, London, 2005, Churchill Livingstone.) |
The anterior mediastinum extends between the sternum at the fourth costal cartilage to the pericardium, and contains loose connective tissue, ligaments, lymph nodes, and branches of the internal thoracic artery.
The middle mediastinum contains the heart, ascending aorta, lower half of the superior vena cava, azygos vein, tracheal bifurcations, and both main bronchi. The posterior mediastinum contains the pulmonary vessels, nerves, esophagus, thoracic duct, and lymph nodes.
The lungs sit on either side of the heart and are free inside the pleural cavity (Figure 10-15). Their only attachment is the heart, trachea, and pulmonary ligaments. In healthy adults, lungs are dark gray in color, feel spongy, and can float in water.
![]() |
FIGURE 10-15 The respiratory tract. (From Standring S, editor: Gray’s anatomy, ed 39, London, 2005, Churchill Livingstone.) |
The trachea is a flexible tube made of cartilage and muscle tissue and is about 10 to 11 cm long. It extends from the larynx at the sixth cervical vertebra to the fifth thoracic vertebra where it divides into the right and left bronchi (see Figure 10-15). The carina, or the point where the left and right bronchi divide, is an important marker for assessing the proper location of a central venous catheter tip (Stonelake and Bodenham, 2006 and Ryu et al., 2007). The bronchi continue to divide into bronchioles and then into alveoli, which are balloonlike structures where gas exchange takes place.
RESPIRATORY FUNCTION
The blood flows into the lungs through the pulmonary arteries, which carry deoxygenated blood. Pulmonary arteries and arterioles are short segments of vessels with large diameters. They have thin walls and are capable of distention, thus allowing for a large blood volume capacity. They follow the path of the bronchi, branching into smaller arterioles with a network of capillaries surrounding each alveolus. The venous side of this same pathway, carrying oxygenated blood, follows the path of bronchioles, and becomes larger as it returns to the pulmonary veins. These veins are short and their ability to distend is about the same as that of the veins in the systemic circulation.
IV solutions contain many types of particulate matter. Undissolved drug particles, precipitate from incompatible drugs, rubber cores of vials or solution containers, glass particles from ampules, and plastic particles from administration sets make up this particulate matter. The most likely place for these particles to accumulate during intravenous infusion is the microcirculation of the lungs. The average diameter of pulmonary capillaries is about 5 micrometers (μm); the capillaries’ small diameter acts as a trap for infused particles and requires red blood cells to change shape in order to pass through them (Guyton and Hall, 2006).
Clinical outcomes associated with infusion of particulate matter include pulmonary granulomas found on autopsy, local tissue infarction, and other forms of pulmonary dysfunction. An animal study demonstrated that particulate matter in antibiotic infusions lead to greater compromise of capillary perfusion in ischemic tissue, not normal tissue. This suggests that critically ill patients could suffer greater compromise to pulmonary function if receiving infusions containing large amounts of particulate matter. Filtration is the recommended method for removing these harmful insoluble particles at present (Lehr et al., 2002, Kuramoto et al., 2006 and Yorioka et al., 2006).
< div class='tao-gold-member'>
Only gold members can continue reading. Log In or Register a > to continue
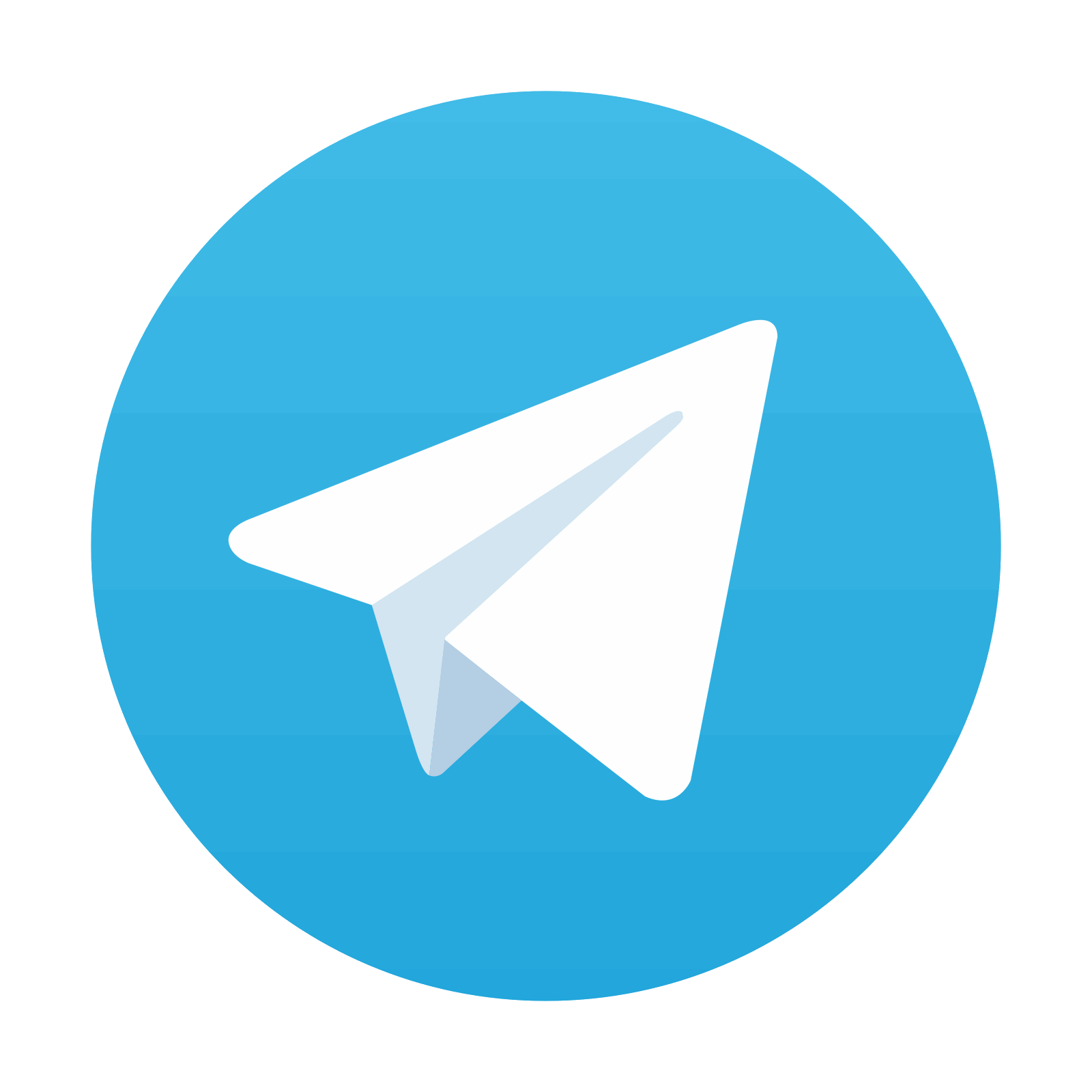
Stay updated, free articles. Join our Telegram channel

Full access? Get Clinical Tree
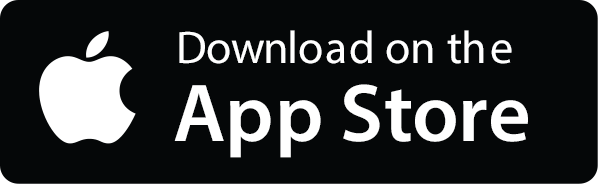
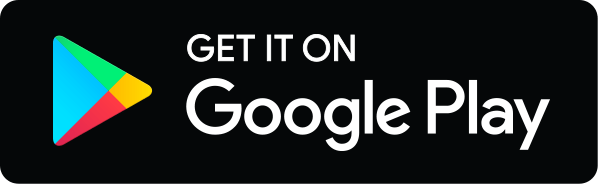