Fig. 7.1
The 2016 World Health Organization classification of tumors of the central nervous system
Because there are many different kinds of brain tumors, the number of children diagnosed with each particular type is small (Fig. 7.2). Advances in successfully treating each subset of tumor have been a direct result of children being enrolled in clinical trials. The majority of those trials are part of the Children’s Oncology Group (COG). Such trials accurately evaluate treatments and recommend standard best treatments for each tumor type. In addition to clinical treatments, COG also conducts biological research focused on identifying possible causes of CNS tumors (Khatua and Jalali 2005). Neurosurgical and technological advances in diagnostic capabilities, radiation techniques, and the use of chemotherapy have radically improved the prognosis for children with CNS tumors over the past few decades. Many CNS tumors carry a greater than 75% chance of long-term survival. Unfortunately, for those malignant tumors that have been refractory to treatment, there still has been minimal progress in identifying effective therapies (Parsons et al. 2016).


Fig. 7.2
The percentage of tumor histologies for the four pediatric age subgroups in the SEER database between 1973 and 2008. The “other astro” category includes diffuse, anaplastic, unique, and not otherwise specified (NOS) astrocytomas. The “other brain” category includes choroid plexus tumors, germ cell tumors, nerve sheath tumors, craniopharyngiomas, hemangiomas, and chordomas (Used with permission from Patel et al. 2014)
7.2 Etiology
Despite the research to date, the cause of pediatric central nervous system tumors remains unknown, though age, gender, hereditary, and environmental factors may be involved (Fig. 7.3). Some CNS tumors have been associated with phakomatoses or hereditary syndromes in children. Examples of tumors with hereditary causes include tuberous sclerosis, which may have tubers within their ventricular system or astrocytomas called subependymal giant cell astrocytomas (SEGAs). Hemangioblastomas are common in children who have von Hippel–Lindau syndrome. Optic nerve gliomas and other CNS astrocytomas can be associated with neurofibromatosis type 1 (also known as von Recklinghausen’s disease). Schwannomas, meningiomas, and spinal cord ependymomas can be associated with neurofibromatosis type 2. Cowden’s disease, Gorlin syndrome (also known as nevoid basal cell syndrome), Turcot syndrome, and Li–Fraumeni syndrome can each be associated with medulloblastomas and other CNS tumors (Kun et al. 2011).


Fig. 7.3
Average annual age-adjusted incidence rates of all primary brain and CNS tumors by region of the United States (0–14 years) (N = 16,044) (CBTRUS 2007–2011)
Radiation exposure has been linked to children with pediatric brain tumors. Children who received cranial irradiation in either low or moderate doses have had a higher incidence of brain tumors (Vinchon et al. 2011). While there has also been discussion regarding the use of cellular phones and high-power electrical wires being associated with CNS tumors, to date there has been no scientific research to confirm this (Kieran et al. 2015).
Recent advances in molecular biology and cytogenetics have begun to identify possible sites of oncogenesis. Mutations in tumor suppressor genes can lead to unregulated and rapid cellular division, and mutations in oncogenes lead to the conversion of normal cells into cancer cells (Ruccione 2011). Alterations in chromosome 17 have been associated with medulloblastoma and astrocytoma, and loss of chromosome 10 has been associated with glioblastoma. Clinical research in pediatric CNS tumors on the cooperative group level is focusing on biology, not only to identify causes but also as a step to develop new treatment strategies (Gajjar et al. 2015).
7.3 Nervous System Anatomy
It is essential to have an understanding of normal anatomy to understand the diagnosis and treatment of CNS tumors. The brain sits inside a solid calvarium, the bony structure that is a fixed volume once the sutures are fused. The spinal cord sits inside the hollow vertebrae of the spine. It is the brain and spinal cord that make up the central nervous system. The brain and spinal cord communicate with the arms, legs, and other organs through the peripheral nervous system (PNS). Control of blood pressure, breathing, and hormonal function is carried out primarily in the brainstem by the autonomic nervous system (ANS) (Hickey 2014).
The largest region in the brain is called the supratentorial region which contains the cerebrum, basal ganglia, and the diencephalon. Symptoms of CNS tumors in this area can be generalized (from changes in intracranial pressure regulation) or focal (from tissue destruction or compressions from the tumor). Focal symptoms include seizures, memory difficulties, headaches, weakness or paralysis of arms and legs, speech abnormalities, personality changes, and visual loss or changes. Generalized symptoms include irritability, lethargy, early morning vomiting, headache, loss of appetite, and behavioral changes (Shiminski-Maher et al. 2014).
The cerebrum is divided into left and right cerebral hemispheres by the great longitudinal fissure and is connected by two bundles of fibers, called the corpus callosum and the anterior commissure. The corpus callosum and the anterior commissure transmit information between the two hemispheres. The cerebrum interprets sensations, controls body movements, and is essential for emotional awareness, memory, acquisition of language, reasoning, and other higher mental functions (Young et al. 2015). In general, motor function for one side of the body is controlled by the opposite cerebral hemisphere. For example, movement of the arms and legs on the right side of the body is controlled by the left cerebral hemisphere. By early school age, around 5 years, a hand preference is usually identified. A person’s speech center is located in the hemisphere opposite hand dominance. This is important when planning treatment for a tumor which is within or adjacent to the speech or motor cortex. In this situation, special preoperative testing may be needed, along with intraoperative monitoring to minimize damage to that area. Younger children have the ability to switch dominance after an injury to the dominant side has occurred or have mixed dominance in terms of speech or motor functioning (Shiminski-Maher et al. 2014).
The frontal lobes make up about 40% of the cerebral cortex and contain the following six functional areas: primary motor, premotor, supplementary motor, frontal eye field, prefrontal, and the Broca speech area. The primary motor cortex controls voluntary movements by sending neural impulses to produce movement in the contralateral side. A lesion on the primary motor cortex that is located in the region that controls facial movement would result in paralysis of the facial muscles on the opposite side. The premotor cortex is located anterior to the primary motor cortex and, when stimulated, produces slower generalized movements of the larger muscles on the contralateral side. A lesion in this area would result in the inability to perform skilled movements on the contralateral side. The supplementary motor area is located anterior and medially to the primary motor area and is responsible for motor planning. A lesion in this area will often result in the inability to perform purposeful movement known as motor apraxia. The frontal eye field is located anterior to the supplementary motor area and is responsible for conjugate eye movements to the contralateral side. A lesion here would result in paralysis of the contralateral gaze. The prefrontal cortex is located anterior to the frontal eye field and extends inferiorly, medially, and laterally making up approximately one-third of the cerebral cortex. It is responsible for social behavior and cognitive abilities. Bilateral lesions on the prefrontal cortex may result in personality changes and inability to concentrate or to solve problems. Broca area is located in the inferior frontal gyrus and is responsible for the motor control of speech. A lesion here would result in the inability of a person to express his or her thoughts, known as nonfluent aphasia (Young et al. 2015; Hickey and Kunusky 2014). (Figs. 7.4 and 7.5).



Fig. 7.4
Principal gyri and sulci in the cerebrum (Used with permission from Wolters Kluwer Health)

Fig. 7.5
Functional areas of the cerebrum (Used with permission by Wolters Kluwer Health)
The temporal lobes are located on the sides of the brain and make up about 25% of the cerebral cortex. Each temporal lobe contains the following five functional areas: primary auditory receptive area, Wernicke’s area (also known as the auditory association area), interpretive area, amygdala, and hippocampus. The primary auditory receptive area is located in the transverse temporal gyrus and is responsible for emotions, speech, and memory. A lesion in this area would cause difficulty determining the direction or distance of sound especially in the contralateral ear. Wernicke’s area is located in the posterior part of the temporal gyrus, is larger in the dominant hemisphere, and is responsible for speech formation and comprehension. A lesion in this area would result in a person substituting meaningless words or phrases that is characterized as fluent aphasia. The lesion would also result in difficulty understanding written or spoken language characterized as receptive aphasia. The interpretive area is located where the temporal, parietal, and occipital lobes meet and is responsible for memory, olfaction, visual, auditory, and emotions. A lesion here would result in significant cognitive impairment. The amygdala is located near the dorsomedial tip of the temporal lobe and is responsible for controlling social behavior and emotions. Bilateral lesions here could result in behavioral changes such as a loss of fear. The hippocampus is located in the floor of the temporal horn of the lateral ventricle and is responsible for short-term memory and ability to learn. Lesions here could result in the loss of short-term memory and ability to learn (Young et al. 2015; Hickey and Kunusky 2014). Seizures are a common presenting symptom in tumors of the temporal lobe (Kun et al. 2011).
The parietal lobes are located posterior to the frontal lobes and make up about 20% of the cerebral cortex. The parietal lobes contain the following three functional areas: primary somatosensory area, secondary somatosensory area, and association area. The primary somatosensory area is located in the postcentral gyrus and part of the paracentral lobule and is responsible for interpreting contralateral tactile sensory information such as pinprick and temperature as well as position of body parts known as proprioception. A lesion in this area would result in a loss of tactile discrimination or proprioception on the contralateral side. The secondary somatosensory area is located in the cortex that extends from the parietal operculum into the posterior part of the insula and is also responsible for the interpretation of tactile sensation. The association area is located posterior to the somatosensory area and is responsible for stereognosis, awareness of body parts, and orderly motor movements. This area is also responsible for visual and auditory processing that is needed for language and cognition. A lesion in the superior region of this area can result in the denial of parts of the body on the contralateral side, also known as neglect syndrome. Lesions in the inferior region of this area can result in the inability to read, write, and perform simple math (Young et al. 2015). Tumors in the parietal lobes can result in seizures, weakness, uncontrolled movements, or sensory changes such as pain or tingling known as paresthesia in the extremities (Shiminski-Maher et al. 2014).
The occipital lobes are located posterior to the parietal lobes and make up about 15% of the cerebral cortex. The occipital lobes contain the primary visual cortex and the visual association area which are responsible for receiving and interpreting information received from the optic nerves, visual fixation, and involuntary smooth eye movements. The left occipital lobe receives information from the right eye, and the right occipital lobe receives information from the left eye (Shiminski-Mayer et al. 2014; Hickey and Kunusky 2014). A lesion in the primary visual cortex would result in loss of peripheral vision of the contralateral side known as a visual field cut. Bilateral lesions in the visual association area would result in loss of color vision and loss of spatial relationships (Young et al. 2015).
The basal ganglia is located deep within the cerebral hemispheres and is interconnected with the thalamus, cerebral hemispheres, and the midbrain. The basal ganglia help to control fine motor movements in the hands and lower extremities. A lesion involving the basal ganglia can result in weakness, abnormal movements, or spasticity (Young et al. 2015).
The diencephalon is divided into four regions: hypothalamus, thalamus, epithalamus, and subthalamus, and it is located in the middle cranial fossa below the cerebral hemispheres (Fig. 7.6). The hypothalamus is located in front of the third ventricle and behind the optic chiasm. It is responsible for many roles in the endocrine, reproductive, and autonomic systems including regulation of temperature, water balance, food intake, emotions, and circadian rhythms. The thalamus consists of a left and right thalami that are connected across the third ventricle by the interthalamic adhesions or massa intermedia in most brains. The thalamus is responsible for the reticular activating system (RAS), limbic system, and the sensory pathways (except smell). It is also responsible for pain awareness and the ability to focus one’s attention. The epithalamus is located behind the thalamus and contains the pineal body which secretes melatonin that helps to regulate circadian rhythms. The epithalamus also helps to regulate the intake of food and water. The subthalamus is located below the thalamus, and its responsibilities are similar to the basal ganglia. A lesion within the diencephalon could result in disturbances of behavior, vision, circadian rhythms, fever, and endocrine abnormalities such as diabetes insipidus (Young et al. 2015; Hickey and Kunusky 2014).


Fig. 7.6
Median view of right diencephalon and adjacent parts of the brainstem and cerebral hemisphere (Used with permission from Wolters Kluwer Health)
The posterior fossa or infratentorium contains the cerebellum, the brainstem, and the fourth ventricle. It is located below the occipital lobe and under a double layer of dura mater called the tentorium (Hickey and Kanusky 2014). Nearly half of all child and adolescent brain tumors originate in the posterior fossa (Kun et al. 2011). The cerebellum controls balance, coordination, the ability to judge distances, and cadence and rhythm of speech. A lesion in this area would result in problems with balance and/or lack of coordinated movements known as ataxia (Young et al. 2015). The brainstem, which contains cranial nerves, is the relay center responsible for transmitting and coordinating messages between the brain and other parts of the body. The brainstem is located behind the clivus and in front of the cerebellum and contains the midbrain, the pons, and the medulla oblongata. The brainstem is also continuous with the spinal cord distally. The midbrain is located at the top of the brainstem and contains cranial nerve nuclei responsible for processing vision and hearing. It also coordinates the sleep and wake cycles. The pons is directly below the midbrain and contains cranial nerve nuclei that control eye and facial movements and also serves as a link between the cerebrum and the cerebellum. The medulla is below the pons and contains the cranial nerves that control breathing, swallowing, heart rate, and blood pressure. The fourth ventricle is a fluid space that connects the upper fluid chambers to the spinal cord and subarachnoid space surrounding the brain and contains the vagal motor center that controls vomiting (Shiminski-Maher et al. 2014). A lesion here could result in impaired gag, swallowing dysfunction, and signs of hydrocephalus such as headache, vomiting, and lethargy (March and Hickey 2014; Cahill and Armstrong 2014).
The spinal cord contains the 31 pairs of spinal nerves that exit at various levels in the cervical, thoracic, lumbar, and sacral regions. The spinal cord sits inside the vertebral column, a series of bones that are stacked upon one another. Nerve impulses travel from the brain down the spinal cord and exit at various levels. They provide sensory and motor stimulation, which result in contraction of muscles in organs in the body as well as movement of the extremities (Hickey and Kanusky 2014).
Figure 7.7 illustrates normal brain anatomy.


Fig. 7.7
Normal brain anatomy (Used with permission from Barrow Neurological Institute. © Barrow Neurological Institute 2000. All Rights Reserved)
7.4 Presenting Symptoms
The diagnosis of a CNS tumor in a child is often difficult to establish. The presenting symptoms of a CNS tumor may be vague or similar to the symptoms of many common childhood illnesses. As there are only approximately 2500 children diagnosed with CNS tumors annually in the United States, the likelihood of a pediatrician seeing a CNS tumor in the practice in his/her career is remote. It is not unusual to get a history from the family of many trips to a health-care provider before the actual diagnosis is made. Many parents express a sense of frustration that they knew something was wrong with their child, and if they had not been persistent in expressing their concerns to the physician, the diagnosis may have been “even more delayed” (Wilne et al. 2010).
Signs and symptoms vary depending upon the rate of growth and location of the tumor. Table 7.1 summarizes symptoms based upon tumor location and tissue type. Tumors with an acute onset of symptoms tend to be more rapidly growing and may be described as aggressive, high grade, or histologically malignant (Watral 2014). Those with a long history of vague symptoms or those picked up incidentally tend to be slower growing, low grade, or histologically benign (Petriccione and Gilger 2011).
Table 7.1
Brain tumors: diagnosis based on location and symptoms
Part of the CNS | Symptoms of tumors | Types of tumors |
---|---|---|
Frontal lobes (cerebral hemispheres) | Problems with learning and concentration, changes in behavior, personality changes, seizures, weakness of an arm or leg on opposite side of tumor | Astrocytoma |
Glioma | ||
Rarely PNET or ependymoma | ||
Parietal lobes (cerebral hemispheres) | Seizures, difficulty processing information, language difficulties | Astrocytoma |
Glioma | ||
DNET | ||
Temporal lobes (cerebral hemispheres) | Atypical/partial complex seizures, behavior problems (aggressiveness, impulsiveness) | Astrocytoma |
Glioma | ||
DNET | ||
Occipital lobes (cerebral hemispheres) | Loss of peripheral vision | Astrocytoma |
Glioma | ||
Cerebellum (posterior fossa) including the fourth ventricle | Problems with balance, uncoordinated gait, increased intracranial pressure, nausea and vomiting | Astrocytoma |
Glioma | ||
Medulloblastoma | ||
Ependymoma | ||
Brainstem (posterior fossa) including the fourth ventricle | Increased intracranial pressure, headache, nausea and vomiting, cranial nerve problems including eye movement disruption, decreased hearing, facial asymmetry, breathing or swallowing difficulties, and problems with balance and strength | Astrocytoma |
Glioma | ||
Ependymoma | ||
Medulloblastoma (rare) | ||
Midbrain/thalamus | Altered level of consciousness, memory problems, weakness of arms or legs | Astrocytoma |
Glioma | ||
Diencephalon (hypothalamus, sella, pituitary) | Hormonal secretion disruption (decreased growth, diabetes insipidus, thyroid deficiency, puberty problems), memory and academic problems | Astrocytoma |
Gliomas | ||
Craniopharyngioma | ||
Germ cell | ||
Optic pathway | Visual changes: acuity or field cut | |
Ventricular system | Increased intracranial pressure, hydrocephalus, memory or academic problems, hormonal changes | Ependymoma |
Choroid plexus | ||
Astrocytoma | ||
Glioma | ||
Medulloblastoma | ||
Spinal cord | Back pain, scoliosis, weakness in arms or legs, bowel and bladder problems | Astrocytoma |
Ependymoma |
On physical examination, the child with a CNS tumor may have specific neurological deficits, which correlate with the tumor location. It is possible for a child to have a normal examination, for example, in a situation where the tumor is diagnosed incidentally because of a diagnostic test being performed for another reason (Shiminski-Maher et al. 2014).
7.5 Diagnosis: Tumors of the Infratentorium
Symptoms of infratentorial tumors are most commonly associated with increased intracranial pressure. Signs of increased intracranial pressure will be discussed in detail in the next section. Other signs associated with infratentorial tumors include ataxia, nystagmus, and cranial nerve problems. Cranial nerve deficits are indicative of brainstem involvement (Parsons et al. 2016).
7.5.1 Diagnosis: Tumors of the Supratentorium
Symptoms of supratentorial tumors include hemiparesis, hemisensory loss, seizures, visual field changes, personality changes, and intellectual problems. Midline tumors, such as those in the thalamus, hypothalamic, or pituitary region, are associated with increased intracranial pressure, visual changes, motor deficits, and seizures. Endocrine issues such as diabetes insipidus or precocious puberty may also exist (Petriccione and Gilger 2011).
7.5.2 Diagnosis: Tumors of the Spinal Cord
Spinal cord tumors usually present with scoliosis, back or leg pain (which often awakes the child from sleep), weakness or sensory changes in the arms or the legs, and/or bowel and bladder dysfunction. Occasionally, a brain tumor will metastasize to the spinal cord and produce similar symptoms to those mentioned above (Shiminski-Maher et al. 2014).
7.5.3 Diagnosis: Increased Intracranial Pressure and Hydrocephalus
Increased intracranial pressure occurs from the mass of the tumor occupying space within the brain. It can also occur when the tumor causes an obstruction in the flow of CSF, resulting in hydrocephalus. Symptoms of increased intracranial pressure include headache (that awakens from sleep), nausea, vomiting (which often temporarily relieves the nausea and headache), lethargy, double vision or other visual changes, gait instability, memory problems, and decline in academic functioning. Nausea is especially problematic, as the nausea centers are located near the medulla that may be compressed or infiltrated by a tumor. Infants whose sutures are open can increase their head circumference to compensate for the pressure (Petriccione and Gilger 2011). Infants and children have the ability to compensate for increased intracranial pressure, especially if the tumor is slower growing and pressure increases are subtle. In addition, these symptoms are typical of many childhood illnesses and, therefore, may go unnoticed (Watral 2014). Late signs of increased pressure are papilledema, vital sign changes, and severe altered level of consciousness (Cahill and Armstrong 2014).
7.5.4 Diagnosis: Diagnostic Tests
The diagnosis of a CNS tumor is confirmed with a radiographic study, which is usually ordered by the primary care provider in a child with symptoms suggestive of intracranial or intraspinal pathology. Several other diagnostic tests may also be performed prior to treatment. Obviously, the extent of testing will depend upon the clinical condition of the patient. In general, the more information available to the treating team, the more thorough the plan for maximal surgical removal of the tumor, followed by appropriate adjunctive therapy (Eche 2014).
Until the late 1980s, the computed tomography (CT) scan was the most frequently ordered imaging test. Magnetic resonance imaging (MRI) of the brain is now the gold standard neuroimaging for brain tumors (Watral 2014). Nurses are generally familiar with this diagnostic test, but not necessarily with how it works. A patient having an MRI scan is placed in a machine that contains a strong magnetic field. This magnetic field causes protons in the water molecules in the body to align parallel or antiparallel within the field, and this generates an image (Krishnamurthy et al. 2016).
MRI is a much more sensitive diagnostic test than the previously utilized computed tomography (CT) scans. Its sensitivity allows for detection of smaller tumors that may be missed with a CT scan. In addition, an MRI provides greater anatomic detail in multiple planes. The administration of a contrast agent (gadolinium diethylenetriaminepentaacetic acid: Gd-DTPA) allows for better visualization of enhancing tumors. Moreover, newer imaging sequences are continually being developed to assist in determining whether a tumor’s pathology is malignant or benign or to differentiate between recurrent tumor and treatment changes. In addition, specific sequences may allow the radiologist to predict specific tumor diagnosis. For example, diffusion tractography, a variant of diffusion-weighted MRI, allows tracing of functional tracts in the cerebral white matter, and the optic radiations can be traced through the occipital lobes. This allows planning a surgical route that avoids the optic radiations, limiting the risk for postoperative visual deficit. Figure 7.8 shows this technique (Hastings et al. 2012).


Fig. 7.8
Diffusion tractography of the right optic radiation in a 3-year-old girl with right occipital ependymoma. The right optic radiation (shown in blue) abuts the ring-enhancing ependymoma
MR angiography utilizes the magnetic field to display blood vessels within the brain. It is helpful in visualizing the blood supply to a tumor or in identifying vessels that may be compromised because of the tumor. It is a noninvasive way of looking at the blood supply within the CNS and has replaced the conventional angiography in many situations. Angiography is used in a small group of CNS lesions whose differential diagnosis includes tumor versus aneurysm/cavernoma or tumors where hemorrhage into the tumor cavity is suspected (Krishnamurthy et al. 2016).
Because the quality of the MRI images is dependent upon the patient lying motionless throughout the study, younger children and some older ones will require conscious sedation or anesthesia to perform the test. Sedation protocols vary with the institution, and larger pediatric centers may have blocks of time set aside with anesthesiologists present to efficiently utilize scanner time. If the child shows clinical signs of increased ICP or has hydrocephalus, it may be the physician’s preference to insert a drain prior to the MRI scan, especially if the child is intubated (Ramaswamy et al. 2015).
A CT scan may be the first diagnostic test performed. Some tumors, especially small ones, may not be seen on a CT scan, and most tumors will not be visualized on a CT scan unless contrast is given. CT scans are most often used during the treatment processes as a screening tool for children who have experienced a change in their neurological status. It can be performed in less than 5 min and is not as sensitive to the child moving as an MRI. The CT scan does expose the child to radiation during the test, and there is a growing awareness of the potential harmfulness of cumulative radiation after many CT scans during the course of the illness (Leonard 2011). For this reason, clinicians will consider alternative testing, such as a fast MRI scan, when ordering a CT scan to avoid the risk of unnecessary radiation exposure (Krishnamurthy et al. 2016).
Other radiodiagnostic tests include positive emission tomography (PET), single-photon emission computed tomography (SPECT), and magnetic resonance spectroscopy (MRS), which focus on a tumor’s metabolism. These tests help clinicians determine the rate of cell growth and to differentiate between active tumor cells and treatment effects such as radiation necrosis. During a PET scan, radiotracer fluorodeoxyglucose (FDG) or methionine (MET) is injected in a vessel, and approximately 30 min later, CT scans are obtained (Bryant et al. 2010). These radiotracers demonstrate low uptake in normal brain tissue and increased uptake in primary brain tumors (Sharma et al. 2016). SPECT also uses radiotracers followed by CT scan to further evaluate lesions (Bryant et al. 2010). If there is concern on imaging following surgery and radiation therapy, MRS provides a noninvasive way to differentiate between tumor recurrence and radiation necrosis (Sanghvi 2009). MRS measures the following four principle metabolites within a tumor: choline, N-acetyl aspartate (NAA), lactate, and creatine. Pediatric brain tumors typically demonstrate elevated choline levels and decreased or absent NAA levels. Lactate levels vary with the degree of necrosis within the tumor (Krishnamurthy et al. 2016).
An electroencephalogram (EEG) measures the electrical activity within the brain and may be performed on a patient whose clinical presentation includes a seizure. Patients with tumors that are associated with difficult to control or generalized seizures undergo continuous EEG monitoring with videotaping in a special epilepsy-monitoring unit. Subdural grids are inserted in some cases to localize speech or motor centers prior to surgical removal of the tumor. A Wada test (intracarotid sodium amobarbital test) may be performed to determine which side of the brain is responsible for speech and memory. This test is slowly being replaced with the functional MRI scan or magnetoencephalography (MEG), which attempts to obtain the same information in a noninvasive manner (Smyth and Vogel 2015). Please refer to the Chap. 13 for more information.
Visual acuity and visual field studies performed by an ophthalmologist are utilized if a patient presents with visual abnormalities. Laboratory tests, including blood and CSF necessary to look for tumor markers or endocrine abnormalities, may also be part of the initial workup. Serum electrolytes, thyroid levels, and growth hormone levels are necessary for hypothalamic/pituitary tumors. Serum and CSF tumor markers of human chorionic gonadotropin (HCG) or alpha-fetoprotein (AFP) are needed for suprasellar tumors which may be germ cell tumors (Shiminski-Maher et al. 2014).
7.5.5 Treatment
Technical advances in medicine and surgery have dramatically changed the management of pediatric CNS tumors over the past several decades. Imaging allows for early diagnosis and treatment of tumors, in addition to monitoring the effects of those treatments. Evolution in the surgical equipment, radiation therapy equipment, and use of chemotherapy for CNS tumors has improved long-term survival and the quality of that survival. Treatment consists of any combination of surgery, radiation therapy, chemotherapy, and observation. Treatment depends upon the location and type of CNS tumor. The tumor location determines the surgical approach. Tumors near eloquent areas may require special planning. The type of tumor refers to its cellular makeup and rate of growth (Parsons et al. 2016).
7.5.5.1 Surgery
Surgery is the primary and frontline treatment for virtually all CNS tumors. On radiology confirmation of a tumor, the child is seen by a neurosurgeon and evaluated for surgery. The goals of surgery are for maximal safe surgical removal and also to provide a tissue diagnosis. It is optimal for a child diagnosed with a CNS tumor to be operated on by a pediatric neurosurgeon. This is because pediatric neurosurgeons are more likely to extensively remove pediatric tumors than general neurosurgeons, and the extent of surgical resection is a significant prognostic indicator (Rosetto and Cartwright 2011).
Ideally, surgery should be carried out as efficiently as possible under elective, controlled conditions. This is possible for the child who has minimal clinical symptoms or for whom medical management can temporize symptoms. Placing a child with subtle symptoms of ICP on steroids may temporarily relieve the symptoms as they decrease edema around the tumor. Diuretics such as mannitol may also be utilized to decrease ICP. The child should be monitored carefully for any change in status. In severe situations of increased ICP, intubation with mechanical ventilation is necessary to assure adequate oxygenation to the brain. Any child with significant symptoms or who is unstable should be operated upon immediately. If the child has hydrocephalus, the surgeon may place a ventricular drain to relieve the symptoms prior to the craniotomy for tumor removal. Simply removing the tumor, however, may open blocked CSF pathways and thus relieve the hydrocephalus (Ramaswamy and Taylor 2015).
Tumors of the hemispheres and posterior fossa are often readily accessible, allowing for gross total surgical resections (Figs. 7.9 and 7.10). Surgical debulking of tumors in the areas of the third ventricle, hypothalamus, optic nerve, and pituitary regions has also become possible. Surgery is not indicated in children with diffuse intrinsic brainstem tumors (most of which are malignant in histology) in which the surgical risks outweigh the benefits of a radical surgical procedure, because the overall prognosis is not changed with surgery. The exception is the focal tumor that is isolated to one area within the brainstem (either only in the medulla or only in the pons or those at the cervicomedullary junction). These tumors are benign in histology and are possible to remove surgically, thus delaying or avoiding adjunctive treatments (McCrea and Souweidane 2015).



Fig. 7.9
Child in prone position in cranial fixation pins in preparation for removal of a posterior fossa tumor

Fig. 7.10
Child in Mayfield head rest prior to a pterional craniotomy (Photo courtesy of Dr. Robert Lober)
Staged surgical procedures and second-look operations have become a frequently used modality to increase extent of tumor resection and to decrease morbidity. Deep tumors can be approach from two different trajectories to maximize the resection. A surgeon will choose to perform a second operation when the postoperative imaging study shows residual tumor and the pathology is low grade. Second-look operations after a specific treatment modality has been given to allow for evaluation of tumor response to the treatment as well as the potential for rendering the patient free of disease (Gupta et al. 2010).
Standard neurosurgical tools utilized by the surgeon in the operating room include the operating microscope, loupes, ultrasonic surgical aspirator, carbon dioxide laser, ultrasound, endoscopy, and intraoperative monitoring, such as electrocorticography and sensory and motor evoked potentials (Fig. 7.11). An MRI scan obtained prior to surgery can provide preoperative and intraoperative localization using a frameless navigational system, as shown in (Fig. 7.12). In some situations, this allows the surgeon to use a smaller craniotomy to maximally remove the tumor (Pollack 2015). An ultrasound is used to localize the tumor, and it is usually removed with the ultrasonic surgical aspirator. An endoscope may be used to remove or biopsy a tumor within the ventricular system. The endoscope can also be used to perform an anterior third ventriculostomy to treat obstructive hydrocephalus, thus avoiding insertion of a ventriculoperitoneal shunt (Fig. 7.13) (Rosetto and Cartwright 2011). The laser is commonly used to remove spinal cord tumors (Wetjen et al. 2015) (Fig. 7.14).





Fig. 7.11
Neurosurgeon in OR using loupes, a magnifying device used to visualize intricate neuroanatomy (Photo courtesy of Dr. Robert Lober)

Fig. 7.12
Intraoperative guidance systems

Fig. 7.13
Neurosurgeon preparing to used an endoscopic

Fig. 7.14
Operating room setup
Intraoperative monitoring (called motor evoked potentials) involves watching the nerve impulses travel from the brain to important functional areas, such as arms, legs, face, eyes, bowels, and bladder (Fig. 7.15). By using this technology during the operation, the surgeon can determine the location of the tumor in relation to important body function, thus maximizing resection while attempting to minimize injury (Groves and Jallo 2015). Electrocorticography, as shown in Fig. 6.6, allows the surgeon to place electrodes or grids into the cavity after a tumor resection to ensure that any abnormal tissue that can generate a seizure is also removed (Pollack 2015) (Fig. 7.16).



Fig. 7.15
Use of evoked potentials during tumor resection to monitor motor function

Fig. 7.16
Grid placement for evaluation of seizure focus outside tumor cavity
Localization of the tumor using intraoperative guidance systems has become common in the last decade. An MRI scan is obtained preoperatively using neuronavigational MRI protocol which includes contiguous, non-overlapping, thin slices (1.0–3.0 mm). If present, surface markers on the scalp must also be included in the MRI. The computerized scan is then sent to the navigational system in the operating room. In the operating room, the surgeon can touch the scalp or surface markers upon the scalp with a stylet, and the computer then generates a picture of the lesion in relation to that specific marker. This allows for surgical removal of a tumor through a much smaller craniotomy, reducing recovery time and length of hospitalization. It is extremely efficient in localizing small lesions within the brain (Ganslandt et al. 2002). Specific MRI scans such as functional MRI and diffusion MR tractography are noninvasive techniques that can be obtained preoperatively to localize the tumor in relation to eloquent areas, such as visual or motor pathways (Shaikh et al. 2015).
Hydrocephalus that does not resolve with the removal of tumor, and for which an endoscopic third ventriculostomy is not possible, is treated with insertion of a ventriculoperitoneal shunt (Madden et al. 2014). The clinicians must also monitor for the development of hydrocephalus in the weeks or months following surgery as approximately 10–35% of patients following posterior fossa tumor removal will require CSF diversion (Morgenstern and Souweidane 2012). See Chap. 2.
7.5.5.2 Observation
A postoperative MRI is performed, usually within the first few days after surgery. The purpose of the scan is to evaluate the amount of tumor removed. This scan, combined with the histology of the tumor, will determine the next treatment step. For tumors that are very slow growing and for which a significant surgical removal has been possible, observation with frequent MRIs may be the only recommendation. The ability to detect subtle changes in tumor growth with the MRI has made clinicians more comfortable in observing these tumors. Observation may delay the use of more surgery or other treatments (Shiminski-Maher et al. 2014).
Observation may be the treatment of choice at the original diagnosis if the tumor is very small and/or was diagnosed incidentally. MRI is again utilized to monitor the lesion for any change in size over time. Observation is also required following any adjunctive treatment that may be used following surgery. Once a child or adolescent has completed additional treatments for their tumor, MRI scans and other pertinent diagnostic tests are performed on an interval basis (Shiminski-Maher et al. 2014).
7.5.5.3 Radiation
Many CNS tumors are very sensitive to radiation treatments, also called radiotherapy. Prior to the technical advances in surgery, radiation was the primary treatment for CNS tumors in children. It became clear as children began to survive their CNS tumors, however, that radiation treatments also carried acute and long-term side effects that dramatically impacted the individual’s ability to function. The intensity of the side effects was directly related to the age of the child at the time radiation was given. In general, younger children were much more vulnerable to significant toxicity than were older children. Common long-term effects include delays in cognition and growth, hormone deficiencies, and hearing deficits. Children who have had radiation therapy may develop vascular abnormalities and secondary malignancies many years after the completion of therapy. Radiation treatments can only be administered once and, therefore, should be employed at the time of minimal disease based upon tumor type and age of the patient. Beginning in the late 1980s, clinicians chose to treat younger children requiring adjunctive treatment with chemotherapy first in an attempt to withhold radiation for as long as possible. This past quarter century has brought about technological advances, which allow for MRI and CT computerized planning of radiation treatments. This allows for focused treatments to the tumor while sparing as much normal brain tissue as possible. Currently, radiation treatments (especially more targeted treatment plans) are being considered for younger children if the tumor is not responsive to chemotherapy (Ermoian et al. 2016).
Radiotherapy directs high-energy x-rays called photons at targeted areas within the CNS to destroy tumor cells. Radiation can be given internally or externally, with the majority of pediatric CNS tumors treated with external radiation. A large machine called a linear accelerator delivers external radiation via x-rays (photons) to the precise portion of the CNS where the tumor is located (Fig. 7.17). These photons enter the patient at a high dose and deposit that radiation to the tumor target and beyond. Radiation doses are measured in Gray (Gy) or centiGray (cGy) (Ermoian et al. 2016). Treatment doses for CNS tumors range between 18 and 60 cGy with the dose determined based on presentation and the type of tumor. If the tumor has the potential of spreading or already has spread beyond the primary site, the entire CNS will receive external radiation dosing up to 23.4 cGy. Radiation treatments are normally given once a day at around the same time. Twice a day treatments (called hyperfractionation) are another way of delivering radiation treatments. The daily dose is split into two fractions and delivered at least 6 hours apart with the intent on hitting the tumor cells at different times of their cycles and increasing cell death (Kun et al. 2011).


Fig. 7.17
Child undergoing radiation therapy with a stereotactic face mask in place to hold the head perfectly still (Photo courtesy of Dr. John Breneman)
Conformal radiation therapy is a technique used to target radiation doses to the tumor while limiting the dose to normal tissues. Conformal radiation therapy requires brain imaging with MRI and CT so that the tumor target and critical normal structures can be accurately defined (Fig. 7.18). It is now the accepted standard for radiation treatments for pediatric CNS tumors. There are several specific types of conformal radiation therapy that utilize photons. 3D conformal radiation therapy uses radiation fields from several directions that overlap at the tumor. In this manner, the tumor receives the high-dose radiation, while the normal tissue surrounding it receives a smaller dose. Intensity-modulated radiation therapy (IMRT) is a sophisticated type of 3D conformal therapy that modifies the radiation beam based on the shape of the target to be treated. Another type of sophisticated 3D technique is stereotactic radiosurgery/radiotherapy, which delivers radiation to a small, precisely defined target. Stereotactic radiosurgery is delivered as one single treatment, while stereotactic radiotherapy is multiple fractionated treatments (Ermoian et al. 2016).


Fig. 7.18
Intensity-modulated radiotherapy plan for treatment of a glioma (Photo courtesy of Dr. John Breneman)
Proton beam radiation therapy (PBRT) is another type of conformal treatment. Unlike the other therapies that utilize photons, this uses protons. Protons, unlike photons, enter the patient with a relatively low dose and deposit their energy within the tumor with no exit dose of radiation to the normal tissues beyond the tumor. Pencil-beam scanning proton therapy is a second-generation PBRT. In addition to preventing an exit dose beyond the tumor, pencil-beam scanning proton therapy helps to decrease radiation exposure proximal to the tumor. New studies have demonstrated that verbal comprehension, perceptual reasoning, and working memory remain stable following PBRT; however, processing speed can decline over time. PBRT is a promising alternative over traditional radiation therapy (XRT) in helping to decrease the long-term neurocognitive effects (Sands 2016). Although PBRT centers have increased in numbers over the past decade, the overall number of centers are few and unable to provide treatment for all seeking care (Vega et al. 2015).
Internal radiation, or brachytherapy, implant therapy, or interstitial therapy is used much less than external radiation to treat childhood CNS tumors. Brachytherapy uses radioactive seeds or implants which are surgically placed into the tumor cavity. Brachytherapy may be useful in treating CNS tumors because it delivers high-dose radiation directly to the tumor site while sparing surrounding healthy tissue. Unlike external radiation, it provides a continuous low dose of radiation to the tumor rather than intermittent bursts once or twice a day (Parsons et al. 2016).
Children should receive radiation therapy at major medical centers with experience in treating children with CNS tumors. This will ensure that treatments conform to the standards set up by COG. Immobilization devices are necessary to ensure that the radiation beam is directed with precision (Figs. 7.19 and 7.20). Sedation or anesthesia may be required on a daily basis for those who cannot tolerate the immobilization process (Shiminski-Maher et al. 2014).



Fig. 7.19
Custom stereotactic radiation mask on a child preparing for radiation therapy (Photo courtesy of Dr. John Breneman)

Fig. 7.20
Child in a stereotactic radiotherapy ring (Photos courtesy of Dr. John Breneman)
7.5.5.4 Chemotherapy
Until the 1980s, chemotherapy was not used to treat pediatric CNS tumors. It was felt that the blood–brain barrier would prevent the penetration of chemotherapy into a tumor within the CNS. Chemotherapy, either alone or as part of a multimodality treatment, is used to treat all malignant CNS tumors and has also been shown to be effective in the treatment of some benign tumors. Cooperative group studies within COG have allowed for testing of specific chemotherapeutic protocols and have been responsible for increasing the progression-free survival in children with CNS tumors. In the past decade, higher doses of chemotherapy followed by stem cell rescue have been used in treating tumors in infants (while delaying radiation treatments) or resistant tumors (Watral 2014). In addition, researchers have begun to look at the genetic components of specific resistant tumors and tailoring treatments based upon this research (Gajjar et al. 2015).
The goal of chemotherapy includes destruction or interruption in growth of tumor cells. The destruction occurs when the chemotherapeutic agent enters the cell and disrupts its proliferative process. In addition to destroying the abnormally growing tumor cells, the chemotherapy also affects normal growing cells such as the hair, skin, and blood cells, with common adverse effects that include immunosuppression, hair loss, nausea, and vomiting. For more information, see Table 7.3 (Shiminski-Maher et al. 2014).
Chemotherapy is given either as a single agent alone or in combination with other drugs. Most treatment plans have a schedule or road map that outlines the drugs given over the treatment plan. When these drugs are given in high doses or at frequent intervals over a long period of time, bone marrow suppression can be a major side effect. Autologous stem cell reinfusion can regenerate the bone marrow, thus allowing for dose-intensive treatments (Watral 2014). Chemotherapy is sometimes given simultaneously with radiation therapy in an attempt to increase the radiation’s effectiveness. In this case, the chemotherapy is referred to as a radiosensitizer (Hanson and Atlas 2016).
7.5.5.5 Rehabilitation
Rehabilitation services are a necessary treatment for the majority of children with CNS tumors. The tumor itself or effects of treatments may impair the use of or coordination in an extremity. Problems with speech, language, memory, and processing may also occur. Physical, occupational, and speech therapy as indicated should be initiated immediately and often will continue for months or years depending upon individual needs. Therapies are incorporated into school educational plans with exercise supplements built into daily activities (Shiminski-Maher et al. 2014).
7.5.5.6 Types of Tumors: Malignant Versus Benign
CNS tumors develop from astrocytes or neuroglial cells. Other tumors develop from neuronal or germ cell lines. After a sample of the tumor is obtained during surgery, a neuropathologist looks at the tumor under a microscope. He determines the type of tumor, depending upon the cell from which it develops and the rate of cell growth. There is no single uniform classification system for pediatric brain tumors. More than one pathologist may look at the same tumor and assign it a different name. This can be very frustrating and confusing for the families. The WHO recently restructured their classification of CNS tumors to include not only histology but also the molecular features of each tumor (Figure 7.1). Although this is an improvement in reaching a uniform classification of CNS tumors, there will still be some tumors that do not meet the criteria for any one specific class of tumors (Louis et al. 2016). Names for different types of CNS tumors include astrocytomas, gliomas, glioblastoma multiforme, ependymomas, oligodendrogliomas, gangliocytoma, ganglioglioma, medulloblastoma, PNET, pineal cell tumors, choroid plexus tumors, germ cell tumors, craniopharyngiomas, hemangioblastomas, and dermoid and epidermoid tumors (Petriccione and Gilger 2011).
The terms malignant and benign are confusing when applied to many CNS tumors. In order to identify if a tumor is malignant or benign, it must be looked at in terms of rate of growth and location. The WHO four-tier grading system is used to classify all tumors according to their histologic features, with the higher grades demonstrating more atypical cellular characteristics that are frequently seen in the highly malignant tumors (Adesina 2010). Unlike adult brain tumors, which are primarily histologically malignant or fast growing, childhood brain tumors have a greater likelihood of being histologically benign or slow growing. When surgically removed, slow-growing tumors rarely regrow and do not require chemotherapy or radiation. However, some slow-growing tumors are found deep within the brain or brainstem where aggressive surgery is not possible because of significant risk of damaging adjacent structures. In these cases, other treatments (chemotherapy and/or radiation) are used in an attempt to shrink or halt further tumor growth. Thus, even if a tumor deep in the brain is slow growing, it may require the same treatments as malignant brain tumors—and these treatments can cause the same long-term side effects. Some health-care providers call this “malignant by location.” Figure 7.21 shows the microscopic histology of a slow-growing tumor (Shiminski-Maher et al. 2014).


Fig. 7.21
Histology of slow-growing tumor (Photo courtesy of Dr. L. David Mirkin)
All fast-growing tumors are considered malignant and cancerous. Figure 7.22 shows the microscopic histology of a malignant CNS tumor. Malignant tumors contain undifferentiated cells that display increased cellular proliferation, significant mitotic activity, and microscopic seeding of tumor cells into surrounding tissue. These tumors can invade surrounding tissue without forming a definite mass. Even if totally removed with surgery, these tumors will usually grow back without further treatment, including radiation and/or chemotherapy. Malignant CNS tumors rarely spread to other parts of the body (Hickey 2014).


Fig. 7.22
Histology of fast-growing tumor (Photo courtesy of Dr. L. David Mirkin)
7.5.5.7 Based Upon Histology
Once the tissue diagnosis has been made with a surgical procedure, the next step is to formulate a treatment plan. Postoperative imaging is obtained to determine the extent of tumor removal that has been obtained. This information, along with any other preoperative testing, is summarized, and the next step of care is recommended. Many large centers utilize a tumor board to make treatment decisions. This is a multidisciplinary group including surgeons, oncologists, neuro-oncologists, radiation therapy physicians, radiologists, and other appropriate specialists. A case may be presented to the tumor board at various times during the course of the illness when treatment decisions need to be made. A summary of treatments based upon tumor histology is found in Table 7.2. A discussion of treatment plans based on specific pathologic diagnosis follows (Shiminski-Maher et al. 2014).
Table 7.2
Brain tumors: treatment based upon type and location of tumor
Part of the CNS | Type of tumor | Treatment |
---|---|---|
Frontal lobes | Astrocytoma | Maximal surgical removal using intraoperative guidance, monitoring, electrocorticography, and MRI; observation between surgical treatments for tumors that are slow growing; chemotherapy and/or radiation may be considered for disease progression in low-grade lesion or for adjunctive treatment for high-grade astrocytomas or gliomas, PNET, or ependymoma |
Parietal lobes | Glioma | |
Temporal lobes | DNET | |
Occipital lobes | Rarely PNET or ependymoma | |
Cerebellum | Astrocytoma | Maximal surgical removal; observation following surgery for slow-growing tumors with reoperation at progression; chemotherapy and/or radiation for medulloblastoma and ependymoma |
Glioma | ||
Medulloblastoma | ||
Ependymoma | ||
Brainstem/fourth ventricle | Astrocytoma | Radiation ± chemotherapy is standard treatment for diffuse brainstem tumors. Surgery is possible for focal lesions followed by observation, chemotherapy, or radiation |
Glioma | ||
Ependymoma | ||
Medulloblastoma | ||
Midbrain/thalamus | Astrocytoma | Conservative surgery or observation for low-grade tumors; observation ± chemo for low grade; chemotherapy and radiation for germ cell or other high-grade tumors |
Diencephalon | Glioma | |
Optic pathway | Craniopharyngioma | |
Ventricular system | Germ cell | |
Choroid plexus | ||
Spinal cord | Astrocytoma | Maximal surgery then observation; radiation for high-grade tumors or tumor progression |
Low-Grade Astrocytomas
Low-grade astrocytomas (LGAs) are slow-growing tumors that rarely metastasize and have low cellularity and low mitotic activity. LGAs are also called low-grade gliomas as they are made up of a type of glial cell called astrocytes. Based on their histology, LGAs are classified as grade I or grade II under the WHO classification system and are the most common type of CNS tumor in children (Petriccione and Gilger 2011). The average age at diagnosis is between 6 and 9 years, and males are more frequently affected than females. Approximately 70% of the low-grade gliomas occur in the cerebellum, but they can occur anywhere in the brain and spinal cord (Parson et al. 2016). The following tumors are included in this group: pilocytic astrocytoma or juvenile pilocytic astrocytoma, pilomyxoid astrocytoma, diffuse astrocytoma, pleomorphic xanthoastrocytoma (PXA), low-grade diffuse astrocytoma, optic glioma, and subependymal giant cell astrocytoma (SEGA). Activation of the mitogen-activated protein kinase (MAPK) pathway by a mutation in the BRAF protein is the most common genomic alteration in low-grade astrocytomas in children (Gajjar et al. 2015). Surgery is the primary treatment for slow-growing astrocytomas. This is possible in many areas of the cerebrum and always possible in the cerebellum. If there is question of residual tumor after surgery, the surgeon can either reoperate or follow closely with frequent MRI scans and reserve surgery unless there is an increase in tumor size. Surgery is also possible with astrocytomas located at the cervicomedullary junction and gliomas growing out of the posterior brainstem (also known as exophytic gliomas) (Parsons et al. 2016).
Pilocytic astrocytomas (PAs) are the most frequently diagnosed WHO grade I astrocytoma with 80% occurring in the cerebellum (Gan and Haas-Kogan 2010). The histologic diagnosis is made with the presence of Rosenthal fibers, eosinophilic granular bodies, and a biphasic pattern of packed bipolar cells mixed with loosely packed astrocytes (Glod et al. 2016). Children with cerebellar pilocytic astrocytomas will often present with signs of hydrocephalus, as the location and size of the tumor will cause obstruction of the outflow of CSF (Kieran et al. 2015). On MRI, most pilocytic astrocytomas have a classic presentation of a cyst with a minimal or non-enhancing mural nodule. Despite sometimes being very large in size, these tumors will have little or no surrounding vasogenic edema (Chapman 2016) (Fig. 7.23). Following complete resection, cerebellar astrocytomas are potentially the most curable form of LGA with 5-year progression-free survival rates as high as 95% (Petriccione and Gilger 2011).


Fig. 7.23
Pilocytic astrocytoma: Sagittal view T1 FLAIR of a solid-enhancing mural nodule arising from the midbrain and pons as indicated by the long white arrow. The tumor is surrounded by a large posterior fossa cyst, indicated by the short white arrow. There is anterior displacement of the brainstem, posterior displacement of the cerebellum and hydrocephalus
Diffuse low-grade astrocytomas (also known as fibrillary astrocytomas) are the most common WHO grade II astrocytoma (Gan and Haas-Kogan 2010). The WHO divides diffuse astrocytomas into three molecular subtypes: diffuse astrocytoma, NOS (not otherwise specified); diffuse astrocytoma, IDH-mutant; and diffuse astrocytoma, IDH-wild type (Louis et al. 2016). These tumors can be found anywhere in the brain and spinal cord with the majority found in the frontal and temporal lobes of the cerebrum (Petriccione and Gilger 2011). The histologic diagnosis is made with the presence of long thin fibrillary cells that comprise a microcytic tumor matrix (Pfister et al. 2009). This tumor is more widely infiltrative of the surrounding normal brain parenchyma and is more likely to undergo malignant transformation compared with the WHO grade I astrocytomas (Parsons et al. 2016). Seizures are a common presenting symptom, especially if the tumor is located in the temporal lobe. On MRI, diffuse low-grade astrocytomas will typically not enhance; however, new post-contrast enhancement will be present if progression to a WHO grade III or grade IV astrocytoma has occurred. Surgery is the primary treatment for this tumor with a goal of a gross total resection (Kun et al. 2011). In cases where the tumor is located in an eloquent area of the brain, chemotherapy or radiation is the primary treatment (Parsons et al. 2016). The 5-year progression-free survival for diffuse astrocytomas is 80% (Kun et al. 2011).
Optic gliomas account for about 3–5% of all pediatric intracranial tumors with the majority of these tumors found in the first decade of life. Most optic gliomas are pilocytic astrocytomas, and some are diffuse or fibrillary astrocytomas (Parsons et al. 2016). Optic gliomas are found in the optic pathway region in the diencephalon and may involve the optic chiasm, optic nerves, optic tracts, and optic radiations (Kieran et al. 2015). Optic gliomas may also invade surrounding structures such as the thalamus, hypothalamus, and frontal and temporal lobes (Imbach 2014). Over 70% of patients with optic gliomas have NF type 1 (Hanson and Atlas 2016). These patients have a mutation of the NF1 gene located on chromosome 17q which encodes neurofibromin, a protein that helps to control astrocyte proliferation (Pfister 2009). Presenting symptoms of optic gliomas are progressive vision loss, nystagmus, proptosis, and strabismus. If the tumor involves other nearby structures, symptoms may include endocrinopathies and signs of hydrocephalus. On MRI, there is a post-contrast enhancing mass that frequently contains cysts and involves at least one of the optic nerves. Surgery is the primary treatment indicated for unilateral tumors of the optic nerve in cases where vision is absent and debulking of the tumor is required to treat hydrocephalus. Surgery is also indicated if vision is deteriorating and there is a cystic or exophitic component of the tumor that is causing compression of nearby structures and is surgically accessible. In patients who are asymptomatic, visual assessments every 6 months and serial MRIs are the recommended treatment (Hanson and Atlas 2016). Following surgery, or if surgery is not an option, chemotherapy has become the next line of treatment for symptomatic patients with optic glioma. This is largely in part due to the risk of developing cognitive impairment, vasculopathies, and endocrinopathies that may occur following radiation therapy (Ertiaei et al. 2016). Radiation is often reserved for tumor progression following chemotherapy (Imbach 2014). Many optic gliomas become dormant in adulthood. Unfortunately, the location of the tumor leads to progressive vision loss resulting in serious long-term morbidity (Kieran et al. 2015). Progression-free survival is poorest in optic gliomas patients that do not have NF type 1 (Kalin-Hajdu et al. 2014).
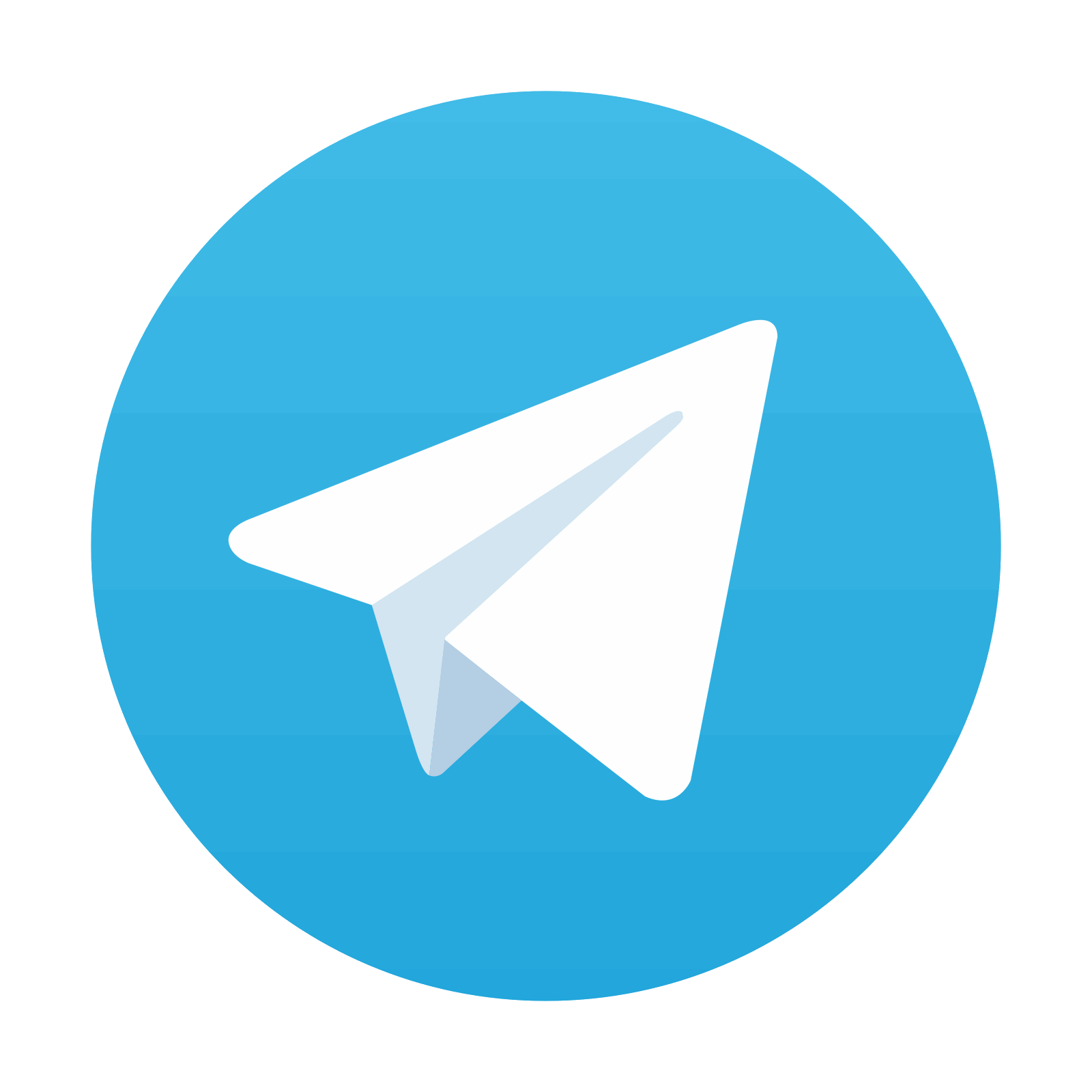
Stay updated, free articles. Join our Telegram channel

Full access? Get Clinical Tree
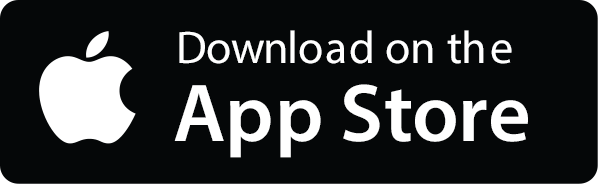
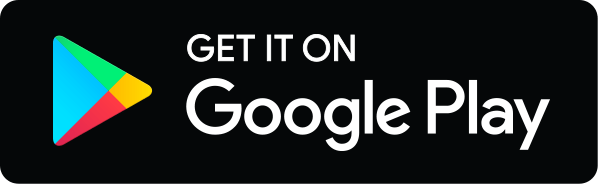
