Chapter 8 The design of a global HIV vaccine1
Since our first knowledge of the HIV-1 epidemic in 1981 it is estimated that over 80 million persons have contracted the disease. In 2010 the United Nations Joint Programme on AIDS estimated a total of 33 million people living with HIV infection and 14,000 new infections per day—the majority of these infections occurring in resource-constrained settings where access to appropriate care and treatment is limited [1]. In sub-Saharan Africa HIV/AIDS is the leading cause of death and has reduced economic growth, caused social disruption, and loss of productivity.
It is possible, however, using available public health measures to significantly reduce the rates of infection. In a setting where the predominant route of infection was through commercial sex workers, Thailand dramatically slowed its HIV-1 epidemic by instituting a 100% condom campaign [2]. However, it has proven difficult to translate success in Thailand to other countries, as the characteristics of local epidemics, drug use, customs, sexual practices, funding, and perhaps viral subtypes have proved difficult to overcome.
For these reasons, since the discovery of the etiologic agent of HIV-1 in 1984, development of a vaccine has been a high priority. A number of approaches have been tested in efficacy trials [3–7], and one was recently found to have modest efficacy [7]. At the same time, efforts at prevention, care, and treatment have appeared to reduce the global burden of infection [1], and efficacy testing of both vaginal microbicides and pre-exposure chemoprophylaxis has recently been shown to prevent infection when used. However, as a public health tool, vaccines have the advantage of not requiring action on the part of the individual (except for vaccination itself). As a part of a comprehensive program of HIV prevention, an effective HIV-1 vaccine would still have a powerful impact on the epidemic, and modeling has suggested that a vaccine with efficacy as low as 30% (± antiretroviral introduction) would be cost-effective and could reduce the epidemic r0 to less than 1 [8].
HIV Biology
HIV-1 is a lentivirus, as are simian immunodeficiency virus (SIV), feline immunodeficiency virus, visna, equine infectious anemia virus, and bovine immunodeficiency virus. The HIV-1 capsid is surrounded by host cell membrane and 20–75 viral envelope spikes (gp120/gp41). The capsid holds two positive strand viral RNA genomes, tRNA, and reverse transcriptase. Viral entry occurs after a high-affinity interaction between CD4 and the gp120 envelope (Env) spike, causing a conformational change and exposure of a co-receptor binding site (CXCR4 or CCR5 most commonly) [9–11]. Interaction with the co-receptor initiates a further set of rapid conformational changes, resulting in apposition of the viral and host cell membranes and, ultimately, fusion. Membrane fusion is followed by entry of the capsid protein into the cytoplasm, release of the viral RNA and associated reverse transcriptase, reverse transcription, and integration of the viral DNA into the host cell DNA.
Once HIV-1 infects CD4 T cells, virions are formed at a rate of 108–109 per day. Infected CD4 T cells have a half-life of 1–2 days [12]. HIV-1 does not always result in lytic infection of the host cell. Latently infected, quiescent cells become a reservoir of infection; cell division is accompanied by bursts of viremia, some cell death, but also incidental replication of virus integrated in the host DNA by homeostatic proliferation [13, 14] (reviewed in Trono et al. [15)].
Two features and related implications of HIV biology deserve to be highlighted with regard to vaccines. The first, which will be described more fully later, is ability of the virus to generate genotypic diversity through its rate of replication, intrinsic error-prone replication, and frequent recombination. Practically this means that the virus rapidly evades or “escapes” from incipient humoral and cellular immune responses [16–18]. More broadly, however, it suggests that it may be necessary to develop multiple “subtype”-specific vaccines or vaccine(s) capable of tackling the issue of subtypes and regional epidemic diversity. The second feature is the speed with which the post-infection events occur; after a “take” the virus spreads rapidly to regional then systemic lymphatic tissue, most prominently the gut-associated lymphoid tissue (GALT). Taken together with the profound and persistent depletion of the GALT and the early establishment of latently infected cells, the prevention of acquisition, if it is possible, may need to occur before productive infection is established—in the earliest phases of virus interaction with the host [17].
Pathogenesis of Acute HIV-1 Infection
In the earliest stages of HIV infection, days–weeks after initial exposure in man, HIV-1 initiates massive infection and depletion of the GALT; home to roughly 70% of CD4 T cells [19–21]. In SIV vaccine challenge models, preservation of gut T central memory CD4 cells is correlated with improved survival. Integrin α4β7, which appears to bind to the V2 loop of HIV-1 Env and to up-regulate virus production, also directs homing of CD4 T cells to gut and genital mucosa through its ligand MADCAM-1, which is expressed on mucosal epithelial cells [22, 23]. Interestingly, the infusion of a rhesus-mouse hybrid monoclonal antibody (MAb) prior to high-dose intravenous (IV) challenge results in significant reduction in plasma viremia and latent virus pools [24]. Whether human vaccines can induce responses that interfere with α4β7 and whether this will affect acquisition of infection remain unknown.
Features of Transmitted Viruses
Within a chronically infected individual HIV-1 exists as a quasispecies, or swarm, with hundreds or thousands of different variants. Diversity is generated through two mechanisms. First, the HIV RT is error prone (3 × 10-5 bases) and lacks a proof-reading function. Second, the virus is capable of recombination (7–30 crossovers per genome) during reverse transcription. Practically this means that, given the rate of HIV replication in chronic infection, thousands of mutant progeny might be generated every day (reviewed in Taylor and Hammer [25)].
However, studies suggest that transmitted viruses have unique features and are not necessarily representative of the quasispecies in the transmitting individual. That the most commonly recognized sequences in the quasispecies are not necessarily the transmitted/founder (T/F) isolate argues that there is “selection” for certain characteristics. It was early found that the vast majority of transmitted isolates utilized the CCR5 co-receptor. Transmitted Envs tend to be shorter and less glycosylated than “chronic” Env [26–29], but this finding has not been universal [30]. Several groups have reported that T/F viruses have shorter V1V2 loops, and it was recently found that the T/F Envs have a greater affinity for α4β7 [31]. T/F strains may be able to use cells with lower CCR5 or CD4 density [31, 32], and are more susceptible to CCR5 inhibitors.
In addition several studies have shown that the typical infectious event in genital mucosal transmission involves a single T/F virus 80–90% of the time, while intrarectal mucosal transmission may involve single T/F 60–80% of the time [31, 33–35]. In transmission cases the founding viruses often resemble ancestral sequences more than the other members of the donor quasispecies [36], suggesting again that there are unique features of transmitting viruses that, in the relatively uncommon event of mucosal transmission, are associated with productive infection. These features of T/F envelopes suggest that there may be a brief window of opportunity, influenced by stochastic events not yet defined, where the individual infecting virus may be uniquely susceptible to innate or adaptive immune effector mechanisms that may limit subsequent events in pathogenesis that lead to local, then systemic, infection [37].
Challenges to HIV Vaccine Development
What makes an HIV vaccine different?
Historically, vaccines have been developed for infections where the natural immune response controls the initial infection and prevents further infection of the host. This does not hold for HIV-1. The host immune response does not eliminate infection or control progressive disease, and in fact, participates in its persistence. The host immune response does not prevent superinfection (this is how recombinants develop). Practically this means that there is no proof of concept from natural infection that informs vaccine development; there is no protective (against acquisition) natural immune response that a vaccine should mimic. An empiric approach to vaccine testing, informed by clues from basic and translational research, would be a traditional response. A positive signal (i.e. protection) would then provide: (1) additional direction or clues; (2) potential or actual correlates of risk/protection [38, 39]; (3) a representative animal model; and (4) a basis upon which to build further informed (but still empirical) testing.
Subtype diversity
HIV-1 diversity is one of the greatest challenges to the development of an effective vaccine and is manifest virologically by the intrinsic error rate of reverse transcriptase and frequent intragenic recombination and at a population level by the diversity of subtypes, recombinant forms, and rapid and continuous evolution within infected hosts. From the time of the initial zoonotic transmission events of HIV (early twentieth century), three main groups are recognized: M (major), O (outlier), and N (non-major, non-outlier). A fourth group, designated P, related to a gorilla SIV, has also been described. There are regionally prominent subtypes that are geographically based and differ from each other by roughly 25–35% (intraperson variation typically is <10% and intrasubtype diversity is 15–20%)—the nine current subtypes and 50+ circulating recombinant forms (CRFs) underscore the complexity of the global epidemic [25]. In general, subtype B predominates in North America and Europe, subtype C in southern Africa and India, subtype A in East Africa, CRF01_AE in Southeast Asia, and CRF02_AG in West Africa. There are other regions with a mixture of subtypes, and some areas, such as East Africa, where ~50% of strains are unique recombinant forms (URFs), found (thus far) in only a single infected individual. Also subtype diversity is increasingly recognized in Europe and the USA; an HIV vaccine that targets only a single subtype may have limited utility in many parts of the world.
Animal models
Non-human primates (NHP) models of infection and challenge have been evolving rapidly in recent years (reviewed in Genesca et al. [40)]. In the absence of a validated animal model (i.e. mirroring what is seen in terms of human protection) it may be important to look at the parallels between the human and NHP infection models. We consider NHP first because many of the other issues are impacted by data from NHP studies, and appreciation of the strengths and weaknesses of the model are critical to this discussion. There are two types of virus: SIV and chimeric SIV-HIV (SHIV). Within SIV there are two different sources of challenge and inserts, SIVmac251 (SIVmac239 is a clone) and SIVsmE660. There are three principal challenge models: intravenous, intrarectal, and intravaginal. All are powered to achieve infection relatively quickly, and not with the frequency of infection/challenge seen with human intrarectal and intravaginal infection.
Vaccination with recombinant Ad5 (Merck) HIV vaccine followed by simian-human immunodeficiency viruses (SHIV) intravenous challenge was associated with reduction in post-infection viral load [41], but did not predict the outcome of the Step trial where the challenge was intrarectal. The SHIV used in those studies, SHIV89.6P, targeted CXCR4+ cells and was therefore not a good mimic of acute HIV infection in vivo. When the challenge was SIVmac251, no benefit was seen [42]. Whether IV challenge with an X4/R5 tropic virus was an appropriate model for screening of candidate vaccines underscores the difficulties faced by NHP studies in the absence of human correlates. Alternatively, SHIV162P3 has been used to demonstrate the efficacy of various subunit HIV Env vaccines [43, 44] and to establish potential correlates of protection. These studies await confirmation in human efficacy testing, which will inform vaccine development and confirm the animal model/virus challenge.
In humans, the viral quasispecies in an infected person is broad (10–15%) and the T/F virus is usually (except in acute infection) a minority member of that quasispecies. In NHP studies, the challenge stocks are more narrow in distribution and are based largely upon SIVmac251 or SIVsmE660 (an alternative SIVdelta670 is not widely used). While it is possible to establish challenge conditions where the number of T/F viruses [45, 46] approximates the human condition, the chances of acquiring infection after a single intrarectal or intravaginal challenge still exceed the probability seen in humans [45, 46]. Since it has long been established in vaccinology that large challenge inocula can overcome vaccines of known efficacy, the impact of the high per challenge infection rate is unknown.
The use of low-dose mucosal SIV challenge has, however, demonstrated acquisition effects similar to that of the Thai HIV vaccine trial [47, 48], and refinements to the NHP model including penile challenge are being developed. One of the vaccines showing a protective effect in NHP is the Vaccine Research Center (VRC) DNA/recombinant adenovirus type 5; it is in phase IIb clinical testing now (HVTN505) and the outcome will inform and refine the NHP model [48]. SHIVs are the only way to test HIV Env vaccines and may be the principal test for new constructs utilizing “mosaic” antigens [49, 50]. The use of new SHIV constructs (SHIV SF162P3/4) and pathogenic SHIVs [51] will provide new insights and may extend the utility of the NHP model in conjunction with advances in human HIV vaccine design.
Defining protective immune responses
Which immune response(s) protects against HIV infection? Unfortunately the answer is unknown. Perhaps the recently completed RV144 trial will provide a correlate of risk that can be incorporated into the testing of future candidates. In the absence of correlate, empirically derived target immunogenicity that can be tested in efficacy trials in humans provides the most systematic (if expensive) approach. It is known from NHP studies that HIVIG and broadly neutralizing antibody (BNAb) can protect animals from intravaginal SHIV challenge. Interestingly, an ADCC null mutation of the Fc chain of the b12 BNAb reduces its ability to protect against infection, suggesting that neutralization works in concert with other humoral effector mechanisms in vivo to achieve an optimal effect [52, 53]. HIV-1-infected persons have recently been the source of a number of BNAbs [54–56] that neutralize 70–90% of all tested viral isolates. These BNAbs have high rates of VH gene mutation (12–30%) and abnormally long HCDR3 regions, so whether BNAbs can be elicited by vaccination is unknown. Also, BNAbs were isolated from persons with chronic progressive infection, implying that these antibodies were a response to chronic infection but did not control it [54–56].
Whether T cell responses, in the absence of antibody can protect against infection is not known [57]. Depletion of B cells did not affect NHP control of an intravenous SIV challenge, though depletion of CD8 T cells did [58, 59]. It is an assumption that the induction of non-neutralizing (and certainly non-broadly neutralizing) antibody may provide de facto evidence that T cell responses are sufficient to protect against infection. The premise of vaccines inducing primarily cellular immune responses (CMI vaccines) was that, while sterilizing immunity might be achieved by BNAb, CMI vaccines might reduce post-infection viral load [60–63] after high-dose intravenous (or mucosa) challenge. More recent studies have suggested that some “CMI” vaccines, such as DNA/Ad5, are capable of inducing antibody at the same level as seen in the Thai HIV vaccine trial and might still protect against infection by SIVsmE660 in low-dose mucosal challenge [48, 64].
Two other sources of information on what might constitute protective immune responses are found in special cases: those persons who, despite repetitive exposure remain uninfected (highly exposed persistently seronegative, HEPS) and those HIV-infected persons whose immune systems appear to nearly completely control viral replication (long-term nonprogressors, elite controllers, EC). It appears the HEPS groups manifest some level of HIV-specific cellular response [65–67]. Both cellular (proliferation) and IgA-mediated HIV neutralization were implicated as protecting HEPS against HIV infection in Kenyan CSW [68]. Among EC strong, polyfunctional, and broad HIV-specific cellular immune responses are associated with persistent, highly suppressive control of HIV infection [69, 70]. Similarly, strong cellular immune responses against SIV associated with post-infection viral load control are reported with both attenuated SIV vectors and replicating CMV-SIV vaccines [60, 61, 71].
Correlates from monkey studies
How much antibody is enough? While protection from intravenous or high-dose intravaginal SHIV challenge required relatively high levels of NAb, polyclonal HIV+ sera, or 1b12 [72, 73], lower doses appear to be necessary for prevention of acquisition using a low-dose intravaginal challenge [72, 74], but even these levels may exceed that seen in human HIV vaccine efficacy trials.
Using a NAb-sensitive SHIV (SF162P4) it was possible to prevent infection via intravaginal challenge using a homologous protein administered intramuscularly (IM) or IM and intranasally [44]. Protection appeared to correlate with NAb levels against SHIVSF162P4. Priming with alphavirus (Venezuelan equine encephalitis) viral vector encoding SF162P4 Env and boosting with a gp140ΔV2 homologous Env (MF59 adjuvant) protected NHP against an intrarectal SHIVSF162P4 challenge. Analogously, protection correlated with NAb to the challenge strain, but was also correlated with total Env binding antibody and pre-challenge avidity [43]. An HIV-ADA-based, DNA prime and MVA boost also protected NHP against intrarectal challenge with SHIVSF162P3 (not neutralization sensitive), and acquisition was inversely correlated with avidity to natural (but not monomeric or trimeric) ADA [75]. Letvin et al. have also reported that, after DNA (SIVmac251) prime and recombinant Ad5 boosting (SIVmac251), protection against intrarectal E660 challenge was correlated with low levels of NAb to E660 in a human PBMC assay and to a lesser degree in pseudovirus TZM-bl neutralization assays [48]. CD4 Env-specific responses were also correlated with protection against acquisition [48]. Whether the same correlations will hold in human studies is unknown. A DNA prime/rAd5 (subtype A, B, C) phase IIb study is currently under way, and has recently been changed to evaluate HIV-1 acquisition (HVTN505).
A replicating CMV-SIV vaccine has been shown to substantially reduce post-infection viral load in an SIVmac239 intrarectal challenge, occasionally to undetectable levels, with CD8 T effector memory (Tem) cells being the immune response correlated with viral load, and more recent data suggest that this vaccine may protect against acquisition as well in a low-dose intrarectal challenge [60].
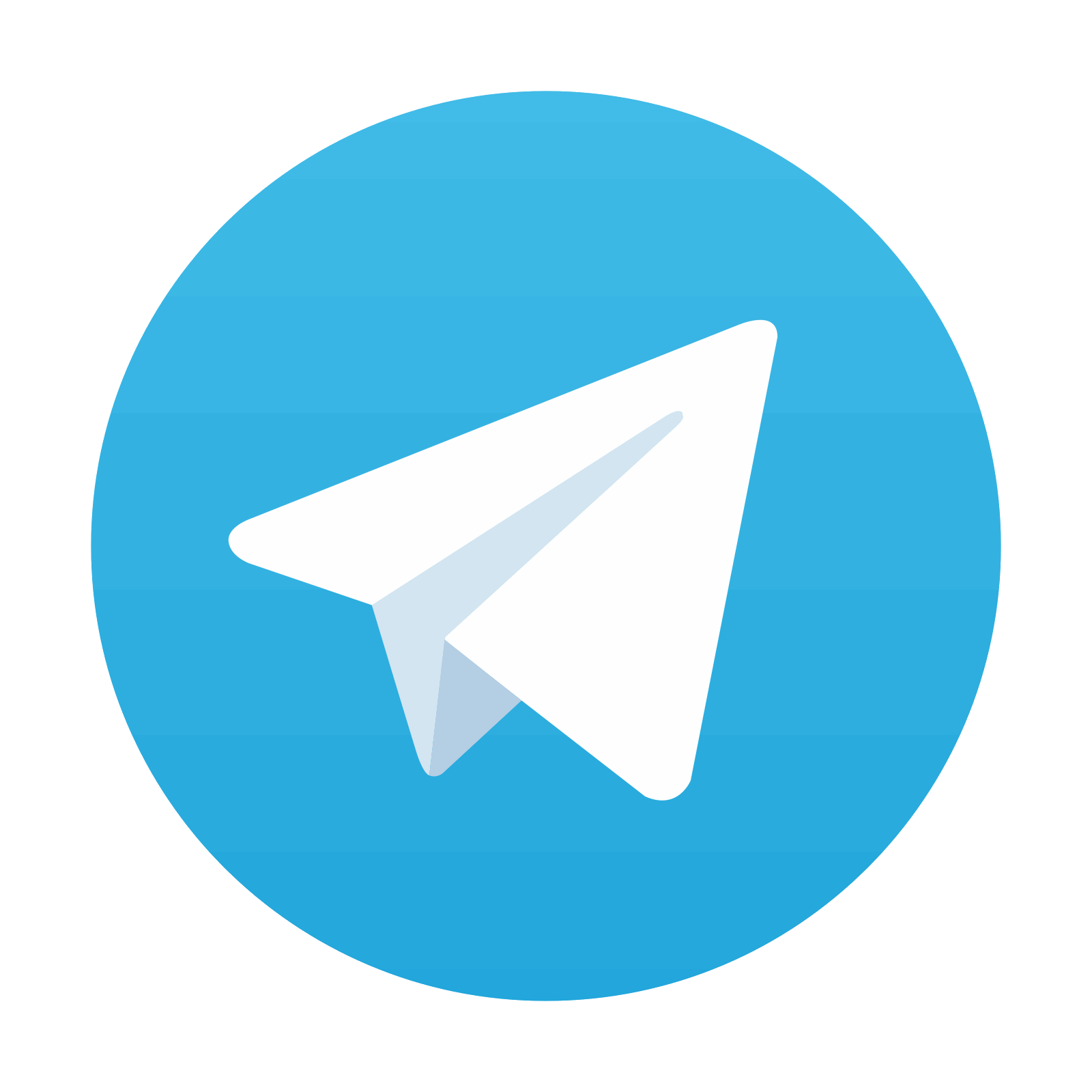
Stay updated, free articles. Join our Telegram channel

Full access? Get Clinical Tree
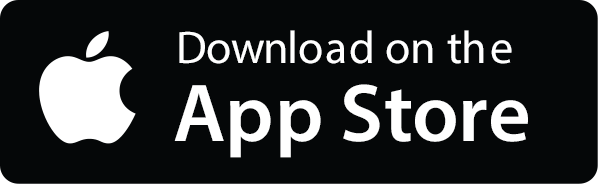
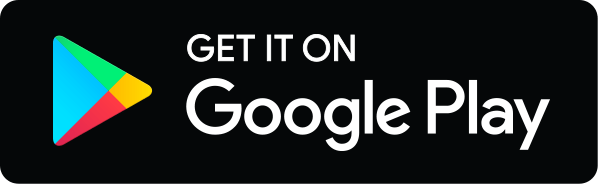