Translating Current Concepts in Brain Science to Inform the Practice of Teaching and Learning
Margaret M. Plack, PT, DPT, EdD and Maryanne Driscoll, PhD
CHAPTER OBJECTIVES
After reading this chapter, the reader will be prepared to:
- Describe some of the key structures and systems in the brain involved in learning, memory formation, and memory retrieval.
- Differentiate between declarative and nondeclarative memory.
- Discuss memory formation and retrieval and the various brain structures and systems implicated in each.
- Discuss the current research on the impact of emotion on attention and memory formation.
- Recognize the potential impact of stress and emotion on the learning environment.
- Recognize the role of emotion and meaning on gaining our learner’s attention.
- Recognize the role of establishing context for learning by connecting new information to prior knowledge and personal experiences.
- Examine the potential implications that brain research holds for the practice of teaching and learning.
- Recognize the need for future research that may enable educators to link current concepts in brain research to educational strategies.
STOP AND REFLECT
Figure 4-1. Structures of the brain. (Reprinted with permission from the National Institute of Alcohol Abuse and Alcoholism.)
- In Figure 4-1, how many of the structures in the brain can you name?
- What are their functions?
- Which structures are most involved in learning, memory formation, and memory retrieval?
You may be wondering why information about the brain’s structure is in a book about teaching and learning. You are not likely to perform neurosurgery! You are an educator in the health care environment, someone who wants to be an effective instructor. That is the main reason for including a section on the brain and how it functions. To teach as effectively and efficiently as possible, it is important for us to work in concert with brain organization and function, not at cross-purposes with it. This is an exciting time in educational research. Rather than working in silos, educators, neuroscientists, and cognitive psychologists are working together to begin to truly link research on the brain and learning to the classroom, and educators are posing complex questions about teaching and learning in the classroom that may well be informed by the research of neuroscientists and cognitive psychologists.1
In any teaching-learning situation, we want to engage learners as fully and as quickly as possible, we want to make complex information meaningful and accessible, and we want our learners to gain and retain the information that we are providing. Whether in the clinic or the classroom, we will have patients and students with different life experiences and different levels of background knowledge. Learning more about how the brain works, how neurons communicate with one another to form and reactivate connections, and how to enhance retrieval of prior knowledge may help to optimize the educational experience. In this chapter, we examine some of the cortical, subcortical, and deep cortical structures and mechanisms critical to the learning process.
Advances in technology have enabled researchers to better visualize what is happening in the brain during learning activities more than ever before. However, it is important to remember that information from neuroimaging studies cannot be applied directly to the classroom or clinic, nor should it be.2 However, what we can do as educators is analyze research findings to help us support or refute our observations about effective instruction in the classroom or clinic.
This chapter is intended to provide a broad and basic overview of neuroanatomy as it relates to teaching and learning. We describe some of the major structures of the brain related to learning, memory formation, and memory retrieval. We do not intend for this to be an in-depth study of neuroanatomy. Current literature on brain function as it relates to memory and learning is vast and growing daily, and we acknowledge that what is to follow may be considered an oversimplification of the complex workings of the brain and its myriad interconnecting systems. Recognizing the complexity of the human brain and that brain research is truly in its infancy, we discuss some of the potential implications for effective teaching and learning, with the caveat that further research is needed to make any direct links to the classroom or clinic. In particular, we explore the implications of stress in the learning environment and the impact of emotion, attention, and prior knowledge on learning. We also discuss various strategies and factors that can be used to enhance not only learning, but also retention of the material being taught. Much of this information has been available to educators through the work of educational theorists and cognitive psychologists. Brain research has provided potential links to neuroanatomical function. While we note that direct links from current brain research to the classroom cannot be made (yet!), the goal of this chapter is to pique your interest in the ongoing pursuit of information related to the potential application of brain research to teaching and learning.
NEUROIMAGING
Current technology enables us to visualize what is happening in the brain during various activities in ways that were impossible before the advent of techniques such as positron emission tomography (PET), magnetic resonance imaging (MRI), and functional magnetic resonance imaging (fMRI).3 Before these techniques became available, researchers relied on the study of pathology, autopsied brains, and more static studies. These newer technologies allow researchers to observe the activity level in various parts of the brain while the individual is engaged in different tasks. With the use of PET scans and fMRI scans and, even more recently, diffusion tensor imaging and magneto-encephalography, scientists can observe the level of activity in the brain during various tasks, enabling them to begin to map the function of the brain.3 Not only can these tools be used to better visualize brain activity during a learning event, but some tools have been shown to have an effect on brain function during the learning event, resulting in better retention. An example is transcranial magnetic stimulation, which has been shown to potentially enhance the formation of short-term motor memories and prerequisite motor learning.4
Ongoing research with neuroimaging tools continues to add to our knowledge of such topics as how abnormalities in neural circuitry involved in emotional regulation and reward processing contribute to bipolar disorder,5 the effects of medication on adults with attention-deficit/hyperactivity disorders,6 and the impact of chemical dependence on decision-making behavior.7 Through brain research and neuroimaging, we know that learning results in physical changes in the brain; networks are developed among neurons and these connections can be reactivated. The term neuroplasticity has been used to describe this ability of the brain to change in terms of structure and function based on learning.8–12 Related to this remarkable ability of the brain to change based on experience is the more recent finding that parts of the brain can grow new neurons throughout life. This process, called neurogenesis, occurs most often in the human brain’s hippocampus.13–15 Current research on neurogenesis has focused on the growth of new neurons as a result of nutrition,16 exercise,17,18 and inflammation.14 Research also continues to explore the role of neurogenesis in mood disorders, especially depression.14,19
BRAIN STRUCTURE AND FUNCTION
Brain Function and Learning on a Macrolevel
There has been a proliferation of popular writing about the brain and decision making in general,20 the brain and decision making in business and marketing,21 the brain and physical exercise,22 and the application of brain research principles to everyday life.20 These texts have piqued the interest of many professionals and lay people in basic brain anatomy and function. In the following pages, we provide an overview of some key terms and concepts related to major brain structures and their function.
One of the first authors to apply what we know about the brain to the teaching-learning experience in the university setting was Zull in his 2002 book The Art of Changing the Brain: Enriching the Practice of Teaching by Exploring the Biology of Learning.11 Zull describes the 3 major functions of the cerebral cortex as sensation, integration, and motion, and notes how these functions not only form the foundation of all nervous systems, but also provide the underpinnings of experiential learning. Incoming data from the external world (eg, sights, smells, sounds) are routed to areas of the brain that can interpret them appropriately. The brain continues to extract meaning from these data and integrates that meaning with prior knowledge to develop new meaning. Developing new meaning often results in new thoughts, plans, or actions.
Zull notes that concrete experience, the first phase of Kolb’s23 learning cycle, corresponds with the reception of sensory data in the sensory cortex and is the first step in the learning process. The second phase of Kolb’s learning cycle requires us to integrate the incoming information to make meaning of the incoming data, using varying degrees of observation and reflection. In the third phase of the cycle, we begin to connect our observations to what we know (ie, prior knowledge) and begin to formulate hypotheses about our experiences, which depend on activity in the frontal cortex and in other cortical and subcortical areas. Finally, in the fourth phase of Kolb’s learning cycle, we begin to actively test those hypotheses and/or implement the various “plans” we developed, which requires activity in the motor cortex.11 As we implement our plans and ideas, we are faced with new experiences. As a result, each experience shapes and reshapes how we learn and how we view the world. Zull11,24 and Kolb23 provide nice neat descriptions of how the brain functions—for which they have been critiqued—because, of course, it is not that simple. Both provide a framework for how learning occurs and what that might look like a from a big picture perspective. Truly what occurs is, as information that comes into the brain is processed, myriad connections are made across a broad range of areas in the brain. Without the imaging we have today, neither Zull nor Kolb could have known how intricate these interconnections truly are; what they did provide, however, is a framework for us to begin to think about how brain function and learning might align.
CRITICAL THINKING CLINICAL SCENARIO
You are a clinical instructor (CI) working with a first-year physical therapy student during her first exposure to clinical education. She is in clinic 1 day/week for 10 weeks. She wants to observe a surgical repair of the anterior cruciate ligament. In the current hospital setting, this is possible, but you would need to rearrange a number of things for this to happen, and the student would miss time observing direct physical therapy interventions. You are just not sure whether the possible benefits of this observation are worth the efforts required to set it up, particularly because it is so early in your student’s education.
Reflective Questions
- Given the description of Kolb’s learning cycle, how might this observation enhance your student’s learning?
- How might this experience enhance her understanding of clinical practice?
- How might this experience help to prepare her when she takes her course in the management of patients with musculoskeletal dysfunction?
- What new information and/or shift in perspective might the student develop as a result of the observation?
- Which learning preference might best appreciate this opportunity?
- What could the you do to maximize this learning experience for students with different learning preferences?
The Neuron: The Basis for Brain Function and Learning
Any review of the learning process must begin with a description of a neuron, which serves as the basis for all learning. What distinguishes neurons from other cells that make up the human body is their ability to “communicate” with each other and form increasingly complex networks based on sending and receiving physical, electrical, and chemical signals.
Figure 4-2 depicts a neuron with its cell body, axon, and dendrites. Learning occurs when neuronal connections are developed; retrieval of prior learning occurs when these connections are reactivated or when the elements of the memory are reconstructed.8,25 Dendrites receive incoming chemical signals, generated by the electrical impulses from the axons of other neurons. Electrical impulses travel down the axon to the axon terminal, where one or more chemicals, called neurotransmitters, are released. Examples of these chemical neurotransmitters include dopamine, serotonin, epinephrine (adrenaline), norepinephrine, and acetylcholine.26 These neurotransmitters spread across the tiny space or synaptic cleft between the end of the axon and the dendrite of another neuron.25,27 On the surface of the neighboring dendrite are receptors specifically shaped to receive certain incoming neurotransmitters. These neurotransmitters can be either excitatory or inhibitory. Once the neurotransmitter fits into the receptor, there is a change in the membrane permeability. Because there can be thousands of dendrites on a single neuron, the number of incoming signals is vast. If enough excitatory signals arrive on the dendrite at the same time, the neuron will initiate an electrical impulse down its axon, beginning another potential neuronal connection. The nervous system communicates via these neurotransmitters and electrical impulses. Any increase or decrease in these neurotransmitters will impact this neural transmission and communication. This simple description fails to convey the vast tangle of networks that develop throughout our lives as we continually form new neuronal connections through learning.
Figure 4-2. Depiction of a neuron, cell body, axon, and dendrites. (Reprinted with permission from Lisa Moglianski.)
A basic adage of neurologists and others involved in applying brain research is, “The more nerves fire, the more nerves wire.”27–30 The task of effective teachers is to tap into and connect new information with whatever prior knowledge or neuronal connections related to that information exists in the learner. Answering questions, repetition, retrieval practice, analogies, multiple modes of presentation, and hands-on activities all related to a single concept will result in multiple neuronal connections being strengthened through the “firing” or transmission of electrical and chemical signals across multiple synapses. Even the act of thinking about a new adaptation of a physical therapy intervention observed in the clinic, for example, can result in neurons being stimulated to form new connections.
STOP AND REFLECT
A first-year physical therapy student is learning basic medical terminology, along with other content, in various courses. The word extension has developed new meaning for this student.
Reflective Questions
- What possible meanings might the word extension have had for a person prior to physical therapy school?
- What other words have new meaning for you now?
- How could the instructor have used the prior connections to teach the meaning of the word in a physical therapy context?
- Can you list as many different study strategies as you can think of to learn medical terminology, to capitalize on the adage “the more nerves fire, the more nerves wire”?
Subcortical and Deep Cortical Structures and Functions
Before addressing the parts of the brain most clearly recognized as being involved with direct instruction, it is important to mention the subcortical and deep cortical structures of the brain, such as the brainstem, cerebellum, thalamus, amygdala, and hippocampus, that are involved in human survival. These structures support critical life functions and are also involved in our learners’ readiness to learn (eg, arousal, attention, motivation) and in their ability to learn, form, and retrieve memories.
Figure 4-3 depicts a medial view of the brain that shows some of these subcortical and deep cortical structures of the brain. Although we generally refer to brain structures in the singular, it is important to recognize that almost all of the structures are paired, with the exception of those at the midline.
Figure 4-3. Medial view of the brain. (Reprinted with permission from the National Institute of Alcohol Abuse and Alcoholism.)
The brainstem is located at the base of the brain where it joins the spinal cord and coordinates sensory and motor information between the body and brain. It contains the midbrain, pons, and medulla. It regulates functions related to survival, such as respiration, blood pressure, heart rate, and sleep. The brainstem also includes the reticular formation, which is a functional system that serves to regulate our level of arousal and our sensory input and motor behavior (including posture and locomotion).31
The cerebellum, a small 2-hemisphere structure, is located just superior to the brainstem and tucked under the occipital lobes. The cerebellum accounts for approximately 11% of the total brain mass; only the cerebrum is larger.31,32 Physical therapy clinicians and students alike will recognize the importance of the cerebellum in maintaining posture and balance and in coordinating automatic movements.
Researchers recently identified functional linkages between the cerebellum and the frontal cortex that suggest an important role for the cerebellum in cognitive and executive functioning.8,33,34 It is believed that the cerebellum is involved whenever we are called upon to coordinate thoughts, attention, and feelings; to sequence information; to prioritize plans; or to figure out how long it will take us to do something.33,35 Not surprisingly, given the importance of these types of functions in interpersonal interactions, the cerebellum is considered to play an important role in our social skills as well.33,35
Researchers continue to examine the nonmotor contributions of the cerebellum in studies of autism,36,37 neuropsychological disorders,38 verbal memory,39 and cognitive development.40 Recent brain research suggests that the function of the cerebellum is much more complex than initially thought. The cerebellum actually functions to support our cognitive processes by linking information from portions of the brain that perform mental tasks with those that gather sensory information. The cerebellum performs this function on a subconscious level, essentially freeing up the conscious part of the brain for additional cognitive activity.41
The thalamus is sometimes referred to as a relay station as it projects fibers to and receives fibers from all regions of the cortex. All incoming sensory data, except for olfactory data (ie, smell), first pass through the thalamus, where they are processed and directed to the appropriate parts of the brain for further processing. As with other sensory data, olfactory data are directed to the cortex for interpretation through the thalamus; however, they also bypass the thalamus and are forwarded directly to the amygdala to be analyzed for danger.42 Through its myriad connections, the thalamus is thought to be involved in sensory, motor, learning, and memory activities, as well as cortical arousal.31
The amygdala is an almond-shaped structure primarily involved in evaluating sensory information. Although its primary purpose is the detection of a potential threat in the environment,43–45 some researchers emphasize that the amygdala evaluates incoming sensory data more broadly, in terms of unpredictability, ambiguity, significance, and salience.45–47 The amygdala can be considered one channel of a multifaceted system for initial processing of sensory data.46,48,49 Because the amygdala has many connections to cortical regions, especially the prefrontal cortex, and to subcortical structures, it plays a role in many aspects of attention, learning, and behavior. Research continues to examine the exact nature of this involvement.43–46,50 The amygdala is also part of a network that includes, among other areas, the anterior cingulate cortex and the anterior insular cortex, which connects emotional content (positive or negative) to a person’s memory of an experience, making it more likely to be remembered in the future.42,49,51
The hypothalamus, among other functions, initiates the physiological responses associated with the fight, flight, or freeze reaction when danger is perceived. When you are frightened by something and experience a sudden increase in your heart rate and respiration, it is your hypothalamus that helps coordinate these reactions. Your hypothalamus also plays a key role in maintaining your body’s homeostasis or balance (eg, sleep-wake cycle, regulation of food and water intake, body temperature, hormone secretion).27,31 As is the case with other cortical structures, research confirms extensive, bidirectional connectivity between the hypothalamus and the cerebral cortex, highlighting the fact that no structure in the brain acts in isolation.46
The hippocampus, another structure with connections to the amygdala and hypothalamus, plays an important role in the formation and retrieval of memories.52 While the mechanism remains unclear, some scientists suggest that the hippocampus is important in helping to shape the cohesiveness of a given memory; however, over time, a memory is consolidated (ie, all sensory data related to that particular memory become linked) and, at that point, the hippocampus may no longer be needed to retrieve the memory; the memory is likely stored in other cortical regions and is now considered stored in long-term memory.25,52–54 Current research on the relationship between the hippocampus and the prefrontal cortex, in particular, suggests that the involvement of the hippocampus in memory retrieval may be of much longer duration than previously thought. The hippocampus may be necessary long after the consolidation of the initial memory if the reconsolidation requires memory traces that are unique to the specific context in which they occurred.52
The basal ganglia are a collection of cells deep within the brain that consist of the caudate nucleus, putamen, globus pallidus, and subthalamus. The putamen and caudate nucleus are also known as the striatum, which receives input from the cortex. Although the function of the basal ganglia is not fully understood, they are known to be involved in the initiation of voluntary movement and in the formation of memories related to skills, habits, and routinized behaviors.27
Before leaving the subcortical and deep cortical structures of the brain, we must briefly consider the essential role of various neurochemicals (eg, neurotransmitters, neurohormones, neuropeptides) in brain function. During learning and memory formation, not only are there structural changes in the brain, but there also is evidence of neurochemical changes. These neurochemicals have modulatory effects on learning and memory formation. For example, the neurotransmitter acetylcholine is linked to the processing of sensory information and memory formation, and has an influence on an individual’s level of arousal and readiness to learn.26 The neurotransmitter dopamine has been implicated in learning based on rewards and reinforcement, as well as movement and memory consolidation.57,58 Neuropeptides have also been shown to influence arousal, concentration, motivation, stress response, and memory formation.26 Finally, varying levels of circulating neurohormones (eg, catecholamines, glucocorticoids) have been shown to either enhance or impair learning and memory formation.26 A broad range of neurochemicals impact the function of all brain structures and therefore affect our abilities to attend, learn, and form and retrieve memories.
By now, you are beginning to understand the complexity of the human brain and the number of structures that have a role in our ability to learn and retain information (ie, form memories). From this, you can see that the human brain drawn by Zull11 and the learning process described by Kolb23 are oversimplifications for sure, but, again, ones that provide frameworks or entry points to thinking about how the brain functions and impacts how we learn.
STOP AND REFLECT
Each of the previously described brain structures is likely to be familiar to you from coursework in anatomy or neuroanatomy. Select 2 of the structures and think about your prior knowledge of them.
- In what ways might knowledge of these structures impact patient care?
- In what ways might knowledge of these structures impact teaching and learning?
- What, if any, shift in perspective have you had about these structures and the teaching-learning situation?
Cortical Structures and Function
All of the brain structures discussed previously often operate on an unconscious level; they detect potential threat, influence what we attend to, and make it possible for us to remember much of what is presented. These structures continuously coordinate physiological responses and allow us to form factual, experiential, emotional, and movement memories. They comprise a relatively small portion of the brain, yet they make it possible for us to learn.
Our ability to reflect on experience, make appropriate decisions, plan ahead, discuss our feelings, and act reasonably depends primarily on our cerebral cortex8,30; however, as noted previously, current research suggests that the cerebral cortex relies on its connections with other structures such as the cerebellum to perform these functions well.33,35
Figure 4-3 shows the cerebral cortex, whose 33-pound weight belies the fact that it is the “greediest” organ of the body and consumes more than 20% of the body’s energy.8,30 Sylwester8 describes the cerebral cortex as being composed of hundreds of millions of highly specialized mini-columns of neurons that process units of information to allow us to make meaning and use of incoming sensory data. The 6 layers of the cerebral cortex enable us to receive, process, and respond to incoming information.
The cerebral cortex is typically described in terms of the right and left hemispheres. Both sides of the brain are involved in all activities and are connected primarily through the corpus callosum. While there remains much debate, current thought suggests that the right hemisphere is dominant for visual-spatial functions (eg, getting the gist or big picture) and attention, whereas the left hemisphere is dominant for language and skilled movement. Research on spoken and written language development confirms that multiple areas of the brain are involved.59 Although dominance is noted, it is important to remember that the corpus callosum allows for interaction between the hemispheres for many functions, including language and movement.27,60
The cerebral cortex consists of the occipital, temporal, parietal, and frontal lobes. The lobes derive their names from the skull bones above each area. The occipital lobes, in the posterior portion of the cortex, process visual stimuli, which is the most highly evolved special sense. There is great specificity among the visual processing neurons in the occipital lobe, with different areas devoted to different aspects of vision, such as distance, depth perception, motion, and color.8,27
The temporal lobes, located on each side of the brain above the ears, are primarily responsible for processing auditory stimuli (eg, language, music perception and comprehension), face and object recognition, and aspects of long-term memory. The temporal lobe is also involved in language comprehension.8,27
The parietal lobes, located near the top of the brain, contain a strip of cells called the somatosensory cortex that processes information related to sensation, such as touch and temperature, as well as the body’s sense of position in space.
Remember Zull’s conceptualization of Kolb’s learning cycle? All learning begins with concrete experience, which generates incoming sensory information. Whether you are a physical therapy student, CI, or patient, the sights and sounds associated with entering a very busy physical therapy gym, for example, will present a great deal of incoming visual, auditory, and somatosensory stimuli that need to be processed for a person to understand what is happening. Clinicians who regularly work with patients in this setting may have forgotten how potentially overwhelming the gym area can be for a patient or student experiencing it for the first time. Allowing time to get one’s bearings, look around, and ask questions can help the learner to process the many incoming sensory stimuli before focusing on the demands of the treatment itself.
CRITICAL THINKING CLINICAL SCENARIO
Think back to the first time you entered a complex environment. For example, think about the first time you entered a hospital room, an intensive care unit, or a busy physical therapy gym. Think about the sounds, activities, and scents. Did you feel a sense of being overwhelmed by different sensory input? Think about entering the same scenario today. Would you be equally overwhelmed? Or does it feel less overwhelming because you have made meaning of the environment? Now imagine working with a young patient who requires surgery and will be entering a hospital for the first time.
Reflective Question
- What strategies might you use to help prepare your patient to not be so overwhelmed by the sights, sounds, scents, etc, that he or she will encounter in the environment as you move from the hospital room to the physical therapy room?
The frontal lobes, more than any of the brain’s other structures, are what make us uniquely human. This area of the brain, comprising 41% of the cerebral cortex, has continued to grow and expand over the past thousands of years because of the increasingly complex and sophisticated tasks we, as humans, have undertaken.8 The frontal lobes enable us to process, plan for, and respond to potential threats and challenges identified by our more primitive structures. They play a critical role in our ability to properly sequence our thoughts and actions.10 Researchers suggest that the frontal lobes, with their interconnections to the sensory processing areas of the brain, allow us to temporarily hold information in our working memory that is needed for us to respond to an environmental demand or retrieve relevant information for problem solving.24 Not only does this area of the brain contribute to our ability to focus attention, reflect on the past and future, and problem solve, it also enables us to be conscious of these abilities.10,25,30
In addition, the frontal lobe plays a significant role in the planning, execution, and control of movement. Of course, the frontal cortex, as all brain structures, does not act in isolation. It requires linkages with subcortical and deep cortical structures for many of its functions (eg, prefrontal cortex and cerebellum [executive function]; amygdala [arousal and attention]; hippocampus and striatum [memory]; parietal, temporal, and occipital lobes [sensory input]; basal ganglia [movement initiation]; cerebellum [movement control]).
The prefrontal cortex is the forward-most portion of the frontal lobes. It is often described as the “CEO” of the brain because it coordinates and integrates almost every function of the brain and is responsible for the highest order of processing. Research from 2013 highlights the role of the prefrontal cortex in memory, especially in the process of integrating new information into existing networks of prior learning.52 Ongoing studies of the adolescent brain indicate that this area of our brain continues to mature, especially in terms of increased myelination, well into our twenties.8,61
Damage to any area of the frontal lobes, particularly the prefrontal cortex, through trauma or cerebral vascular accident can result in problems with executive function, defined by Squire and Kandel as “the ability to direct one’s actions toward future goals.”25 Difficulties with executive function include problems in planning, coordination, and inhibition of impulses, any of which can pose a challenge in a teaching-learning situation.62 Suggestions for providing patient education to individuals who demonstrate impairments in executive function appear in Chapter 9.
Beneath the cortex (ie, gray matter) is an area described as white matter. This description is derived from the myelin, or white fatty covering, of many axons. The myelin functions to speed the transmission of electrical impulses along the axons. The white matter within the cortex facilitates communication throughout the central nervous system. The axons within the white matter form the following 3 major bundles or large tracts:
- Commissural fibers make connections between the 2 cerebral hemispheres. A good example of commissural fibers is the corpus callosum.
- Association fibers make connections within a single hemisphere. Short association fibers connect within the same lobe; long association fibers connect different lobes.
- Projection fibers make connections between the cortex and the rest of the nervous system. A good example of a bundle of projection fibers is the internal capsule, which connects the basal ganglia and thalamus, among other structures.31
These fibers serve to integrate information from multiple regions of the nervous system. For example, they serve to link sensory and motor information, speech and language information, and auditory information with visual information. It is the complexity of these interconnections that allows us to process, integrate, coordinate, and act upon the multiple types of sensory information that we receive.
For example, as you are sitting at your desk, you may begin to feel a sensation of being cold. This subconscious sensory information is brought to the conscious level in the cortex, where a decision needs to be made. Your brain begins to connect this sensation with other incoming sensory input (eg, you feel a cool breeze, you see an open window, you hear trees rustling outside), and you then make a conscious decision as to whether you should close the window, turn up the thermostat, or find a sweater.
Movement: A Sample of the Complexity and Interconnectedness of Brain Function
Before leaving this section on brain structure and function, it is worth reinforcing that these structures do not work in isolation. Rather, it is the interconnectedness of these structures that enables us to learn and function effectively. Performing a goal-directed movement is an excellent example of the complexity of this process and provides a basis for you to understand how we both teach and learn movement, which will be explored in future chapters. Bear et al27 describe a goal-directed movement as requiring the following 3 levels of activity:
- Strategy: Before initiating a movement, we must first obtain sufficient sensory information to determine where we are in space and what surrounds us. From there, we must determine the goal of our movement and the best approach to use in achieving that goal. This planning may require input from multiple areas of the cortex, including the parietal lobe (sensory input), the prefrontal lobe (planning, abstract thinking, decision making, and anticipating potential consequences), the motor cortex of the frontal lobe (movement activity), the temporal lobe (auditory input), the occipital lobe (visual input), and some of the deep and subcortical structures, such as the basal ganglia and thalamus. We need to link information from each of these structures to strategize in planning a motor act.
- Tactics: Once we have decided on the goal of the movement, we must determine the appropriate sequence, timing, and direction, among others, needed to achieve a smooth movement. This requires activity in the motor cortex of the frontal lobe and activity from the cerebellum. The cerebellum is intimately involved in ensuring that movement occurs smoothly, timely, and with precision. Through various feedback loops, the cerebellum functions to continually adjust the movement to ensure the successful achievement of the goal.
- Execution: Implementing the movement requires the activation of the appropriate muscles to perform the movement. This requires activity in structures in the brainstem, spinal cord, peripheral nervous system, and muscles or effectors. Once movement is initiated, ongoing neuronal feedback among cortical cells and neurons in the basal ganglia, thalamus, and cerebellum is required to again continually adjust our movement and posture.
This is a relatively simplistic description of how goal-directed movement occurs; however, it demonstrates the complexity of movement and the vast number of brain structures required in the control of movement. This clearly complicates our task of helping our patients to learn effective movement strategies.
KEY POINTS TO REMEMBER
- It is the interconnectedness of brain structures and systems that enable us to learn and function effectively.
- Learning requires a coordinated effort among the following:
- Cortical structures
- Subcortical structures
- Deep cortical structures
- Functional neurochemical systems
- Cortical structures
- Function requires neuronal communication in the following:
- Between hemispheres
- Within hemispheres
- Between cortical and subcortical structures throughout the nervous system
- The frontal lobes, in particular the prefrontal cortex, are the areas of the brain that make us uniquely human. Damage in this area has major implications for all aspects of the teaching-learning process.
- The brain sculpts itself (changes physically) based on interaction with the environment (neuroplasticity).
- We are all wired differently based on our previous experiences.
- Theories and information about how the brain functions are being continually updated based upon advances in neuroscientific techniques.
- Between hemispheres
MEMORY FORMATION AS THE BASIS FOR LEARNING
How do we know that we have learned something? As a CI, how will you know whether your student knows enough about gait training to allow him or her to assist patients? How will your patient know how to perform his or her exercise program correctly at home? The answer to all of these questions has to do with memory. The only way we can gauge success in teaching is by assessing our learner’s ability to retrieve and implement the knowledge and skills we taught. One way a learner can tell that he or she has learned something is if he or she can demonstrate that knowledge. In all of these examples, the learner must retrieve the knowledge from what is referred to as long-term memory. How initial attention to something ultimately results in long-term memory is an integral part of the learning process. Because memory is inextricably intertwined with learning, it is essential for educators to use what we know about the process of memory formation to facilitate learning.
We have explored the function of various cortical and subcortical structures of the brain as the foundation for teaching and learning. Memory formation is the basis upon which we learn. Memory formation, like many other processes throughout the brain and body, requires the use of multiple memory systems, multiple neuroanatomical structures, and multiple neurochemical systems throughout the brain.54,55,58,63–66 In this section, we provide a broad overview of memory, including information on what it is and how it is formed.
Types of Memory
Researchers generally describe 2 major types of memory, although the words they use to label them vary. Squire and Kandel26 provide a concise history of various approaches to the study of memory in their comprehensive book, Memory: From Mind to Molecules. While they use the terms declarative and nondeclarative to classify the 2 types of memory, others use the terms explicit and implicit, respectively, to describe these same processes.25,27,55,67 Declarative or explicit memory refers to memories that can be verbalized, declared, or made explicit. Declarative memory is subdivided into semantic memory and episodic memory, as described in the following:
- Semantic memory refers to our memory for factual information, general knowledge about which we can speak or write, and information that is available for conscious recall. These are memories of people, places, and things that are not connected to a specific event (time or place). Semantic memory allows us to remember something and describe it.
- Episodic memory, the other type of declarative or explicit memory, is more autobiographical and allows us to remember when and under what circumstances we learned that information; it is specific to a certain time and place. It represents a more complex aspect of memory than being able to label or describe something.
Declarative memory enables us to make comparisons with what we already know. These memories are encoded in terms of relationships among multiple events, which allow us to generalize across similar concepts.25,67 Explicit or declarative memories are also shaped and reshaped over time and with each new related experience.27,68 Although the hippocampus is most directly involved with the formation and retrieval of long-term declarative memories, our long-term memories are thought to be stored throughout the cerebral cortex.25
Nondeclarative or implicit memory occurs earliest in human development, when a baby has not developed sufficient language to describe the memory. It includes memory for any automatic behaviors and skills, conditioned responses, and unconscious awareness of past experience.25,55,67 Nondeclarative memory includes several categories of memories, procedural, emotional, and classical conditioning.27 The 2 that we are most concerned with are as follows:
- Procedural memory is our memory for skills and habits such as piano playing and bicycle riding. This type of memory forms the basis for motor learning and for how we teach movement, which will be discussed in a future chapter. Nondeclarative procedural memories require repetition and practice over a longer period of time and are less likely to be forgotten than declarative memories. For example, you may not remember how you learned to tie your shoes, but you can tie your shoes with ease.
- Emotional memory is our memory that has been associated with positive or negative arousal. Emotional memory formation—fear, in particular—has been a subject of significant ongoing research.55,56,69–72
CRITICAL THINKING CLINICAL SCENARIO
You are working with a patient who has diabetes. You notice that the patient is wearing new shoes and, when the shoe is removed, you see that there is an area of redness on your patient’s foot. You had instructed your patient about the importance of foot care and examination of the feet on a regular basis and you had described the potential consequences of not being vigilant. At that time, your patient had expressed little interest in following your recommendations. Now, your patient appears concerned about the redness and comments, “I haven’t been checking my feet and I am afraid I have developed a bad blister; now I know why you want me to check my feet every day. I’m going to use different shoes and pay more attention to my feet.”
Reflective Questions
- Why might this patient be more diligent about foot care and self-examination in the future?
- What memory process is being engaged in this patient?
- How might your knowledge of brain function help to explain this new desire to be more diligent?
- How can you help this patient remain diligent?
How Memories Form
Once a person attends to incoming sensory information, the potential for learning occurs, and this act of learning is linked to the process of memory formation and memory retrieval. Memory used to be considered in terms of where learned information was stored in the brain; a physical location that was determined after something was learned.22,73 Memory also used to be thought of as a process of recording and storing experiences, almost like on video.34 There remains much that is still unknown about how memory works. Current researchers concur, however, that there are multiple memory systems involved in memory formation and memory retrieval, each with projections to multiple cortical, subcortical, and deep cortical areas requiring input from various neurochemical systems of the brain.25,54,55,66
An important component of memory formation is the concept of consolidation, the process by which memory traces are strengthened, reorganized, and linked to prior knowledge and experiences.52,74 The process of consolidation can occur over periods varying in length from hours to days or longer.74 Whenever a learner needs to retrieve previously learned information (ie, remember, recall), the memory traces, once again, are strengthened, with components of them becoming clearer and connected to prior learning in additional ways.52 This process is referred to as reconsolidation.74,75
Current thought suggests that the hippocampus, along with other parahippocampal and cortical regions, is involved in the consolidation and retrieval of most declarative and nondeclarative memories.25,27 The striatum in the basal ganglia, with input from several cortical and subcortical structures, is critical to the development and retrieval of procedural memories27; the amygdala is essential to the development and retrieval of emotional memories.56,76–78 In addition to the amygdala, the paraventricular nucleus in the thalamus has been identified as a key component in the retrieval and maintenance of long-term memories of fear in animals.79,80 As you will read later, not only do the structures of the brain play a critical role in our memory formation and retrieval, but so do our neurotransmitters, neurohormones, and other neurochemicals.56,81
Although multiple structures are involved in the memory formation, it is important to remember that these structures do not work in separate and independent ways. There is fluid interaction among all of the neural pathways.25 Once incoming information is attended to, these stimuli must be scanned for relevance (threat and meaning) and therefore need to be routed to the appropriate areas of the brain for analysis.46,52
As previously described, most incoming sensory information is routed through the thalamus. The thalamus processes this sensory information and directs it to the appropriate sensory processing areas of the cerebral cortex and other areas (eg, visual data to the visual cortex, which covers the occipital lobe). A small portion of incoming information makes its way to our consciousness, and this information forms the basis of what we consider our thoughts, conscious visual and auditory memories, and language-based memories.
People often use a multistep framework to conceptualize the various aspects of memory formation and retrieval. As long as we do not consider this model as depicting actual places and discrete systems in the brain, it can help us to make sense of how we encode, store, consolidate, retrieve, and integrate new information into existing neuronal networks (Figure 4-4). Typically information moves from sensory input, to immediate or working memory, to short-term memory, and, after some time, to long-term memory after being consolidated. It has been noted, however, that some memories can move directly from sensory input to long-term memory.27
Figure 4-4. Framework for memory formation and retrieval.
The neural connections that make up a memory lie in many different areas of the brain, often near the part of the cortex involved in the original processing. For example, memory traces of a physical therapy student’s first day in an acute care setting, with its myriad visual, auditory, and olfactory cues, may be scattered among the occipital lobes, temporal lobes, hippocampus, prefrontal cortex, and amygdala, especially if some of the sights, sounds, and smells from that day were emotionally charged. Whether the student’s experience was positive or negative, it was likely emotionally charged, and, as a result, the amygdala was involved in coordinating memory formation.55,76 If the student visits a hospital again years later, the various sights, sounds, and smells of the environment may easily trigger memories of that first internship. Just as the amygdala was involved in coordinating the actions of the cortical, subcortical, and neurochemical systems in the formation of this emotional memory, it is likely involved in the reformation and retrieval of this emotional memory.76,82
The frontal lobes, especially the prefrontal cortex, hold new information a few seconds in what Squire and Kandel25 refer to as immediate memory and others describe as working memory.27 Much of the incoming information will be discarded and never make it into our short- or long-term memories. When incoming information is considered important enough for more analysis, it is routed to the hippocampus and then back to the prefrontal cortex for further processing and consolidation into our long-term memory.52
Whether one refers to this aspect of the memory formation as immediate or working memory, researchers agree that time and capacity are limited in working memory. For declarative memories, rehearsal or repetition is often what allows us to maintain and begin to encode information in our working memories.27 Active manipulation or elaboration (ie, further processing) of the data is necessary to move information from working memory to long-term memory. Examples of elaboration include answering questions, focusing on the meaning or relevance of the information to be learned to one’s own life, grouping discrete pieces of information into meaningful units, creating a story or song based on the content, or employing other mnemonic devices.25,30,83 Additional strategies for memory enhancement will be discussed in the next section.
IMPLICATIONS FOR TEACHING AND LEARNING
As noted in the introduction to this chapter, we cannot currently make a direct link from brain research to the classroom.2,84 However, what we can do as educators is remain abreast of current brain research and become critical consumers of the research (ie, continually question and analyze what science is telling us, reflect on how it may or may not apply to our own practices).85 Teaching, like physical therapy, is both an art and a science. Our role as evidence-based practitioners is to understand and appropriately apply current research in designing effective teaching-learning situations. As noted earlier, it is important that we work in concert with brain function and not at cross-purposes. What becomes challenging is that, as in all of health care, new and, at times, conflicting information is being discovered daily and it takes time for consensus to develop among scientists. What we currently know about the brain is quite limited; as research continues, new knowledge will be developed, and it will be up to us to determine how and when to apply that knowledge not only in the clinical setting, but also in the classroom setting. In addition, the application of all new knowledge requires systematic assessment and evaluation in the classroom and the clinic.
Having discussed brain structure and function, we will now explore some potential implications that current brain research may hold for us as educators. While only future research will be able to solidify the direct links between brain research and classroom teaching, research findings suggest a number of important concepts to consider in planning optimal teaching and learning experiences. In the next section, we examine the following concepts related to teaching and learning: stress and emotion; arousal and attention; past experiences and prior knowledge; practice strategies; reflection; desirable difficulties; mindset; and the role of sleep, exercise, and nutrition on learning and retention. We end the chapter with concepts related to memory and aging.
STRATEGIES TO ENHANCE MEMORY FORMATION
Earlier in the chapter, we described memory formation as the basis for learning and discussed the potential implications that cognitive neuroscience holds for teaching and learning. The purpose of incorporating content boosters into your presentations is to reinforce content and enhance learning. Here, we provide additional strategies that you can use to enhance your memory formation, retention, and retrieval, and encourage you to consider using these same types of strategies in your presentations and with your patients to help them learn and retain the information you are providing.
Rehearsal and Elaboration
How many times have you forgotten someone’s name right after being introduced to him or her? Has anyone suggested any strategies to help you better remember the name of the person to whom you were introduced? Perhaps they suggested that you say the person’s name immediately (eg, “Oh, it is so nice to meet you, Mary”) or try to associate the person’s name with something about him or her or someone else you know (eg, Mary has the same color hair as my Aunt Mary, who also happened to grow up in the same state as Mary). These are examples of 2 key strategies to enhance our memory formation, rehearsal, and elaboration. We use rehearsal and elaboration to manipulate information while in working memory to increase the likelihood that this information will make its way into long-term memory.30 Repetition is one of the most commonly used types of rehearsal. We literally repeat what it is that we need to remember. Think about a time when you needed to remember someone’s phone number long enough to punch in the numbers; you probably used repetition in this situation. If you were interrupted in the process, however, you probably could not remember the telephone number. Straight repetition may help you to remember the phone number for a brief period; however, if you want to remember a particular phone number long term, repetition may not be sufficient. Later in this chapter, you will see how varying your practice and using different retrieval strategies will help you to retain this information longer.
Repetition is particularly effective for skill or procedural memory. Repeating or practicing a motor skill over and over generally results in improved performance and enhanced memory of movement, especially if you vary the practice. We provide more suggestions for getting the most out of repetitive practice later in this chapter and in Chapter 8. For example, at some point in time, the steps involved in a task such as riding a bicycle become unconscious and you began to go through them automatically. Repetition helped you to store these movements in your implicit or procedural memory.
Elaboration, or elaborative rehearsal, is a more effective strategy for manipulating semantic information while in working memory.30 Brown et al,74 Medina,83 and Squire and Kandel25 emphasize the importance of focusing on meaning when trying to learn new semantic information. One example of an elaborative rehearsal strategy is to create a story to remember a list of items. You are more likely to remember the list of items if you create a story that includes all of the items that you want to remember in a cohesive narrative; rather than simply trying to memorize the list. The story helps to put meaning to the words in the list, even if it is a silly or fun story!
STOP AND REFLECT
Try it! Think of a long list of items that you need to remember for class. Perhaps it is all of the bones in the wrist or foot.
- Try to memorize the list first and see how you do.
- Now build a story around that list. Tell your story as you recall each item on the list and then see how you do.
A mnemonic, as noted previously, is a form of elaborative rehearsal as well. For example, how do you remember the names of the planets in order? Have you used a story such as, “My Very Educated Mother Just Served Us Nine Pickles,” to remember Mercury, Venus, Earth, Mars, Jupiter, Saturn, Uranus, Neptune, and Pluto? We will not tell you how many years ago we learned this “story,” but it remains easily recalled as a result of both repetition and elaboration. This strategy is not used solely in grade school; you likely have used the same strategy to learn material in graduate school. For example, how do you remember the cranial nerves? Does the story, “On Old Olympus’ Towering Tops A Friendly Viking Grew Vines and Hops,” sound familiar? How do you remember whether each nerve is a sensory nerve, motor nerve, or both (mixed)? Listen to the following one-line story/mnemonic: “Some Say Marry Money, But My Brother Says Big Brains Matter More.” Does it have meaning for you?
There are many other familiar activities that provide opportunities for elaboration, although we might not have considered them in this framework. Whenever we engage in a group discussion about newly presented material or we debrief after practicing a new physical therapy intervention, we have a chance to increase our connections to the new information and to increase the likelihood that we will remember it. Reciprocal teaching, whereby learners partner up and take turns teaching one another, in their own words, the key points presented by the instructor, is an excellent activity to foster elaboration. For example, in the clinic, the physical therapist can ask the young patient to describe the key components of his or her home exercise program (HEP) and how he or she might work on it at home to his or her parent before leaving the session as a means of facilitating both repetition and elaboration for the purposes of retrieval practice and enhanced recall.
KEY POINTS TO REMEMBER
- Rehearsal and elaboration can enhance memory formation.
- Rehearsal, or repetition, is most effective for nondeclarative or implicit memory formation (including skill and procedural memory).
- Elaboration, or elaborative rehearsal, is most effective for declarative memory formation.
- Elaborative rehearsal includes strategies such as the following:
- Creating stories
- Creating mnemonics
- Discussions
- Debriefing sessions
- Reciprocal teaching
- Creating stories
- Rehearsal, or repetition, is most effective for nondeclarative or implicit memory formation (including skill and procedural memory).
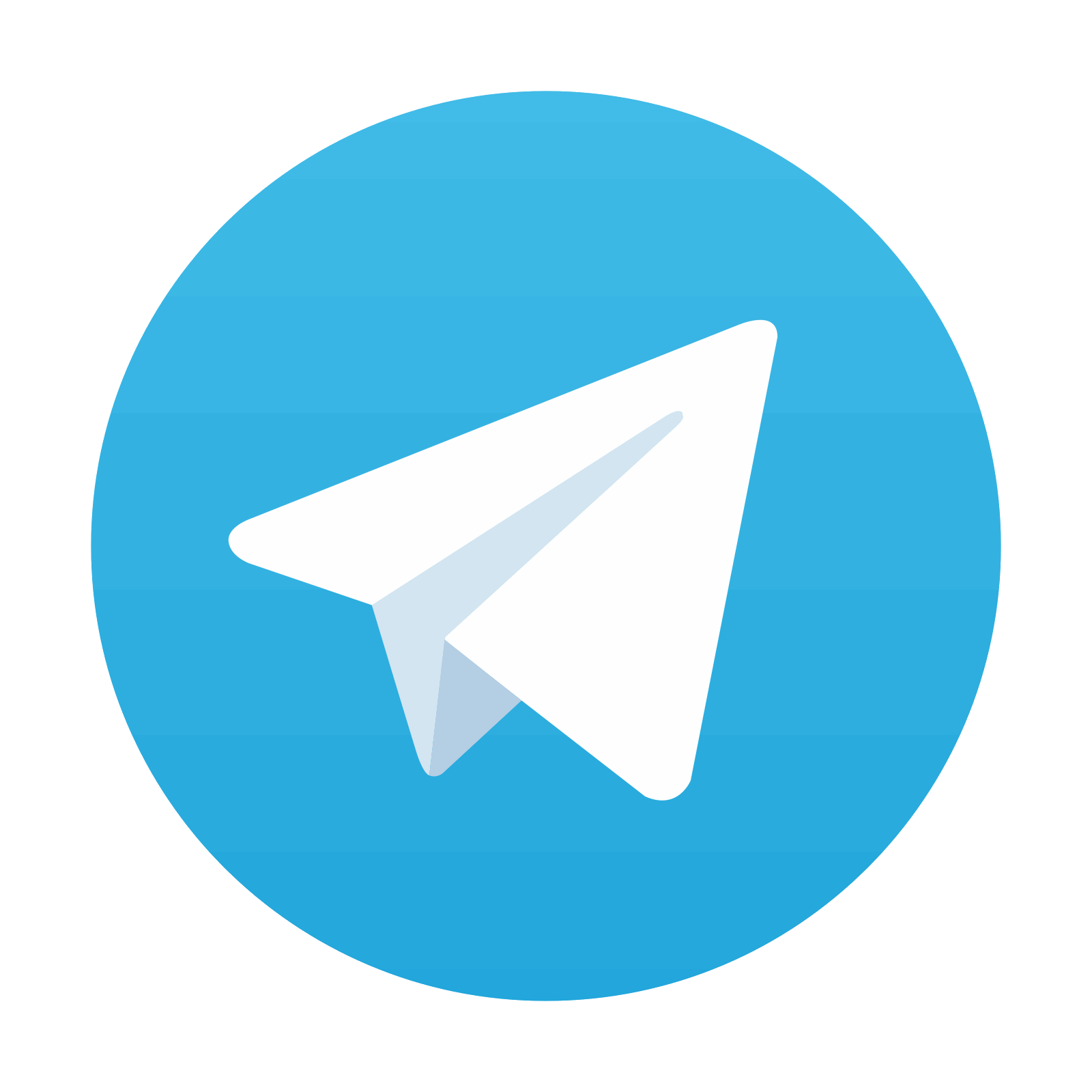
Stay updated, free articles. Join our Telegram channel

Full access? Get Clinical Tree
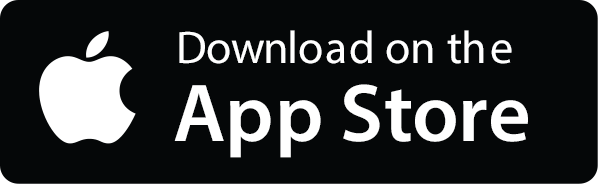
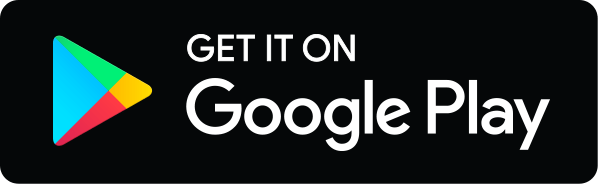