(1)
Department of Neurosurgery, Children’s Hospital Colorado, University of Colorado School of Medicine, 13123 E. 16th Ave, 330, Aurora, CO 80045, USA
Electronic supplementary material
Supplementary material is available in the online version of this chapter at 10.1007/978-3-319-49319-0_13. Videos can also be accessed at http://www.springerimages.com/videos/978-3-319-49318-3.
13.1 Introduction
Epilepsy is the most common neurologic disorder of childhood, affecting 1 in every 100 children (Karsy et al. 2016; Ramey et al. 2013). Epilepsy has historically been defined as two or more unprovoked seizures separated by at least 24 h (Linehan and Berg 2011). An unprovoked seizure is one that occurs in the absence of an acute event, such as a traumatic brain injury or metabolic disturbance. The International League Against Epilepsy (ILAE) further expanded this definition to include individuals with epilepsy syndromes and individuals who are at higher risk for recurrent seizures (Fisher et al. 2014). The majority of individuals with epilepsy will obtain good seizure control with antiepileptic medications. However, up to 30% will be intractable to medical therapy (Karsy et al. 2016; Ramey et al. 2013). Medically intractable epilepsy can have devastating consequences on the developing brain, as can side effects from antiepileptic medications. Certain persons with medically intractable epilepsy, particularly those with identifiable lesions, malformations of brain parenchyma, or associated syndromes, may be candidates for surgery to help treat or even cure their epilepsy. Undertaken in the right patient, epilepsy surgery can allow for improved neurological outcomes, independence, and overall better quality of life.
13.2 Seizures
Initially, in a child’s workup, it is important to distinguish true epileptic seizures from non-epileptic behaviors. Non-epileptic events like breath-holding spells, syncope, and rhythmic self-stimulatory behaviors can be confused with seizures (Jette and Wiebe 2016; Asano et al. 2013). A complete and detailed history, thorough physical exam, and adjunct testing such as an electroencephalogram (EEG) can help diagnose seizure activity. An EEG records the electrical onset and progression of a seizure.
A seizure is a change in neurological functioning secondary to hypersynchronous discharges in cortical neurons (Linehan and Berg 2011). The specific neurologic changes that are seen during a seizure depend on the areas of brain affected. Seizure semiology is a description of the signs and symptoms displayed by a patient during a seizure. A universal classification system for seizures was first developed in 1964 by the Commission on Classification and Terminology of the International League Against Epilepsy (ILAE). The International Classification of Epileptic Seizures (ICES) was then revised in 1981 and 1989 to include epilepsies and epileptic syndromes. A more recent reclassification occurred in 2010 (Berg et al. 2010). The classification system defines seizures based on EEG findings and clinical manifestations. It provides a common nomenclature for providers across disciplines to describe seizures and the possible underlying pathology (Kellinghaus and Luders 2011; Loddenkemper 2011). Other classification systems, such as the semiology seizure classification (SSC) by Luders et al. (1998), have been proposed, which classifies seizures solely on semiology. Proponents of this system argue that it is more descriptive and applicable for everyday clinical use and can be used successfully to lateralize and localize seizure onset (Hirfanoglu et al. 2006; Parr et al. 2001). However, critics state that it is simply a list of descriptive epileptic features and not a classification system (Kim et al. 2002). The ILAE classification remains the more widely used, but some argue that it remains incomplete in its comprehensiveness and descriptions (Panayiotopoulos 2012).
13.3 Seizure Types
Globally, seizures can be classified as partial or generalized with further subdivisions within these classifications. Partial seizures involve an area of cortex that can vary in size but are isolated to one hemisphere. Simple partial seizures tend to involve a small area of cortex and consciousness is not impaired. Complex partial seizures involve a larger area of cortex and consciousness is impaired. Often, there is memory loss around the seizure event. Clinical manifestations in both types of partial seizures can be motor, sensory, somatosensory, autonomic, or psychic in nature depending on the area of cortex involved. Both types of seizures can stay limited to the initial area of onset, or they can spread. Simple partial seizures can progress into complex partial, which in turn can progress into a generalized seizure (Kellinghaus and Luders 2011).
Generalized seizures occur when both hemispheres are affected. Generalized seizures can be idiopathic (with onset in both hemispheres), or they can be the result of secondary spread from one hemisphere to the other (generalization). Generalized seizures are further described as tonic-clonic, tonic, clonic, myoclonic, atonic, and absence. Tonic-clonic seizures are the most common type of generalized seizure and are frequently referred to as grand mal. They are manifested by a loss of consciousness and an abrupt sustained contraction of multiple muscles (tonic phase), including respiratory muscles. The patient may fall, be incontinent, and often becomes cyanotic. A clonic phase then follows which involves repetitive convulsive movements throughout the body (Kellinghaus and Luders 2011).
Tonic seizures involve an abrupt contraction of muscles resulting in rigidity of the extremities and neck. There can be turning of the head and deviation of eyes to one side. Clonic seizures are typically characterized by repetitive muscle contractions. Myoclonic seizures, often called myoclonic jerks, are sudden, brief, and very strong muscle contractions that last less than 2 s. They may be confined to a specific area of the body or they may occur all over. Atonic seizures are a sudden and complete loss of muscle tone. They too can manifest in a specific area of the body or they can affect many muscle groups. When this occurs, they are referred to as drop attacks. Absence seizures are characterized by a sudden arrest in the individual’s activity. A blank stare or slight deviation of the eyes is accompanied by a lack of response to any external stimuli. Absence seizures can be accompanied by tonic, clonic, and atonic components (Kellinghaus and Luders 2011; Tatum 2011).
Seizures of all types can last a few seconds to several minutes. Some are self-limiting, with the seizure activity stopping on its own. Others require the administration of antiepileptic drugs to stop them. Status epilepticus is recurring seizure activity without a period of recovery in between. All individuals with epilepsy are at risk for status epilepticus.
Careful identification of the semiology of seizures helps to determine appropriate treatment. Initial treatment of epilepsy begins with placement on an antiepileptic drug (AED). The choice of particular AED is based on the type of seizure, possible seizure etiology, frequency of seizures, and the presence of associated syndromes. A single AED will control seizures for the majority of individuals with epilepsy (Tsur et al. 2011). In a study by Kwan and Brodie (2000), 64% of newly diagnosed patients with epilepsy had their seizures controlled with medication. Of these patients, 47% responded to the first AED tried. Another 13% responded to a second drug when the first failed, but only 1% became seizure free when trying a third. An additional 3% became seizure free with the combination of two AEDs, leaving 36% of their study population with medically intractable seizures (Kwan and Brodie 2000; Holmes 2002).
Medical management of seizures beyond the use of AEDs is fairly limited. The ketogenic diet can be an effective treatment for some individuals but is not without drawbacks and risk (Nordli and De Vivo 2011; Neal et al. 2008). High-dose vitamin B supplementation may control seizures for a very small number of patients with a rare form of vitamin B deficiency (Neubauer et al. 2011). However, the number of patients well controlled on either of these therapies is small. The mainstay of medical management is AEDs, and there is a high risk of becoming intractable for those not responding to the first one or two medications tried.
13.4 Intractable Epilepsy
Making the diagnosis of medically intractable, or drug-resistant epilepsy (DRE), can vary among providers. The diagnosis is made based on multiple factors including number of antiepileptic medications tried, duration of treatment, degree of response, and tolerance of the medications. Other important factors include the number and characteristics of the seizures, the impact of the seizures on the patient, and the presence of an identified epilepsy syndrome (Go and Snead 2008; Ramey et al. 2013). Ambiguity in the diagnosis exists because the impact DRE has on a specific individual and family can vary greatly, as seizure frequency and semiology may be more disruptive for one patient than another (Schuele and Luders 2008). A grand mal seizure occurring three to four times a month in an older child with repeated absences from school as a result has a different impact than the same seizure frequency in an infant.
In an effort to improve patient care and facilitate research, the ILAE in 2010 released a consensus definition for DRE as “failure of adequate trials of two tolerated, appropriately chosen and used antiepileptic drug schedules (whether as monotherapies or in combination) to achieve sustained seizure freedom” (Kwan et al. 2010). This definition is now widely accepted and has shown interrater reliability in its use (Hao et al. 2011; Brodie et al. 2013).
Up to one third of patients diagnosed with epilepsy are at risk of having DRE (Karsy et al. 2016; Pittau et al. 2014; Ramey et al. 2013). Certain risk factors have been identified that are associated with the development of intractable epilepsy. The first is a patient’s initial response to medical management. Kwan and Brodie (2000) found that response to the first AED tried was a strong predictor of intractability. Of the 53% of patients who did not respond to the first AED tried, only 17% went on to become seizure free with the use of other medications. Further, patients who experience failure at higher dosages are also more likely to be resistant (Brodie et al. 2013). The number of seizures a person experiences before treatment was also found to be a strong predictor of drug-resistant epilepsy. Among patients who had more than 20 seizures prior to initiating treatment, 51% experienced intractable epilepsy, as compared to only 29% of patients who had experienced fewer seizures (Kwan and Brodie 2000; Holmes 2002). There are also specific etiologies of epilepsy and epilepsy syndromes that are known to be associated with intractability. These include temporal lobe epilepsy, cortical dysplasia, hemimegalencephaly, tuberous sclerosis, Sturge-Weber syndrome, Rasmussen’s syndrome, and the presence of focal lesions such as tumors (Go and Snead 2008; Cross et al. 2006; Kwan and Brodie 2000).
It is important to identify individuals with intractable epilepsy as early as possible. The sooner such individuals are identified, the earlier other therapies, such as surgery, can be considered. Drug-resistant epilepsy typically exists with other comorbidities either as a result of the underlying etiology of epilepsy, side effects of antiepileptic drugs, or effects of the seizures themselves. These comorbidities include cognitive impairment, developmental delays, attention deficit, depression, anxiety, and increased risk of death (Karsy et al. 2016; Kim and Ko 2016; Brooks-Kayal et al. 2013). The longer DRE goes untreated, the more significant these comorbidities can become.
Studies have shown that the effect of poorly controlled seizures on the brain, particularly the developing brain, can be devastating and result in significant cognitive decline (Shurtleff et al. 2015; Berg et al. 2012; Farwell et al. 1985). Not only are children with intractable epilepsy more likely to have lower IQs than their healthy counterparts, but their IQs have also been shown to decline progressively over time the longer their seizures are uncontrolled (Farwell et al. 1985). Similarly, antiepileptic medications can adversely affect cognitive and behavioral development (Jenny et al. 2016; Aldenkamp et al. 2016). Antiepileptic drugs work by decreasing the excitability of neurons; thus, they can also impair cognition. These effects are heightened when polypharmacy and increased doses are needed (Kim and Ko 2016; Meador 2011).
Seizures also have a tremendous social and financial impact on affected children and their families. Not only can the actual occurrence of seizures socially isolate a child and his or her family, but the social stigmata that still exist around epilepsy can be isolating as well. Seizures persisting into adolescence and adulthood can severely limit independence and overall quality of life (Puka and Smith 2015; Cross et al. 2006). Finally, although rare, sudden unexplained death in epilepsy (SUDEP) affects approximately 1 person in 1,000 individuals per year and remains the leading cause of epilepsy-related death (Maguire et al. 2016; Seneviratne 2016). Sanchez et al. (2015) found that across all age groups, epilepsy surgery yielded a higher life expectancy than medical treatment alone.
13.5 Surgical Candidate Selection and Preoperative Workup (Phase I)
Surgical treatment should be considered in all individuals who have proven themselves to have drug-resistant epilepsy (Jette and Wiebe 2016; Pittau et al. 2014). In the appropriately chosen patient, epilepsy surgery can result in improved cognitive and quality of life outcomes by decreasing exposure to antiepileptic medications and continued seizure activity (Guan et al. 2016; Karsy et al. 2016; Ramey et al. 2013). Yet, some debate still exists on the which patients should be referred for surgery and when (Engel 2013; Ramey et al. 2013; Go and Snead 2008). Research supports earlier referral and intervention, if appropriate, to achieve the best outcomes (Jenny et al. 2016; Engel 2013; Ramey et al. 2013). In particular, studies have shown better cognitive outcomes and seizure control in toddlers and preschoolers (Shurtleff et al. 2015). The goal of epilepsy surgery is to eliminate or decrease the number of seizures, without causing a permanent neurological deficit, and limit the long-term effects of continued seizures and exposure to AEDs. (Karsy et al. 2016; Engel 2013; Vendrame and Loddenkemper 2010; Schuele and Luders 2008). This goal can be achieved in over 80% of appropriately chosen patients (Ryvlin and Rheims 2016; Karsy et al. 2016). Identifying appropriate surgical candidates requires a thorough and systematic workup. The goal of this workup is to identify the epileptogenic zone, or area of seizure onset, and determine if it is resectable. The success of epilepsy surgery is directly related to accurate identification of this zone and ability to remove it (Guan et al. 2016; Nissen et al. 2016).
All patients being considered for epilepsy surgery should be referred to a center with the necessary resources available for appropriate evaluation and workup. Appropriate evaluation requires a multidisciplinary team with specific expertise in pediatric surgical epilepsy. This team includes epileptologists, neurosurgeons, advanced practice providers, neuropsychologists, therapists, EEG technicians, and physiatrists. Phase one of the evaluation involves gathering information from a detailed history, physical exam, EEG, and imaging. A detailed history can provide information regarding behaviors observed before, during, and after a seizure. It also provides information regarding age at onset, progression of seizures, and seizure semiology. The physical exam can reveal neurologic deficits that may be associated with the affected areas of the brain. A scalp EEG can show areas of epileptiform discharges and can often lateralize onset and show other areas of potential onset. However, the scalp EEG in and of itself is limited, as rapid generalization of seizure activity and distortion by tissue can misrepresent the area of onset.
13.5.1 Imaging
The goal of neuroimaging in the workup of patients with DRE is to identify the epileptogenic zone and any related structural abnormalities, as well as the location of eloquent brain in surrounding areas. Workup tends to proceed from least invasive to maximally invasive, and multiple modalities are often used to accurately define the epileptogenic zone (Pittau et al. 2014; Go and Snead 2008). Various imaging modalities have become increasingly more sophisticated and sensitive at not only detecting structural abnormalities but also in identifying areas of differing metabolism that are associated with seizure onset. The ILAE has recommended that all patients with DRE should undergo a high-resolution brain MRI, done with a specified epilepsy protocol, as the primary imaging study (Go and Snead 2008). MRI can help identify structural abnormalities that may be causing seizures. Examples of structural abnormalities include neoplastic or vascular lesions, cortical anomalies like cortical dysplasia (abnormally formed cortex) and heterotopias (normal gray matter in an abnormal location), or mesial temporal sclerosis (neuron loss and scarring in the temporal lobe). As MRI technology has advanced, the ability to detect even very subtle abnormalities has increased. CT can be useful in identifying areas of calcification, which are not well visualized on MRI.
Functional MRI (fMRI) works on the principle that increased neuronal activity is associated with increased blood flow. The primary role of fMRI in the workup of a potential surgical candidate is to identify areas of eloquent brain. fMRI has shown efficacy in lateralizing language and identifying motor and sensory tracks (Widjaja and Raybaud 2008) (Figs. 13.1 and 13.2).



Fig. 13.1
fMRI showing activation with word generation

Fig. 13.2
Left: sentence completion. Right: verb generation
Positive emission tomography (PET) and single-proton emission tomography (SPECT) scan also work on the principle that increased neuronal activity results in increased blood flow and increased metabolic demand. Areas of epileptogenic focus tend to be hypometabolic during times when a seizure is not actively occurring (interictal) and hypermetabolic with increased blood flow during a seizure (ictal). PET scan is generally performed interictally. An agent, typically FDG (fluorodeoxyglucose), is injected intravenously, and the brain is then scanned looking for areas of hypometabolism, as evidenced by decreased agent uptake. SPECT uses a different agent (HMPAO) which is often injected during the ictal phase. Scans are then obtained looking for areas of increased uptake. A second SPECT scan can be obtained interictally looking for areas of decreased uptake. Often, the two scans are compared, and the subtraction between the two can provide additional information (Go and Snead 2008; Widjaja and Raybaud 2008) (Figs. 13.3 and 13.4). Both FDG and HMPAO are radiopharmaceuticals and expose children to ionizing radiation.



Fig. 13.3
Interictal PET scan showing decreased uptake in right posterior temporal occipital lobes (note the lack of redness shown by the arrows)

Fig. 13.4
Ictal SPECT showing increased uptake in right hemisphere (note increased redness in area shown by arrows)
Magnetoencephalography (MEG) is a noninvasive imaging technique that can help localize the epileptogenic zone. MEG measures neuronal activity by measuring the magnetic fields produced by electrical activity. Unlike scalp EEG monitoring, MEG allows for direct measurement of the electrical activity without distortion from surrounding tissues (Englot et al. 2015; Pittau et al. 2014). Potential areas of seizure onset are seen as spikes on MEG and have been shown to correlate with epileptogenic areas identified with subdural electrodes. MEG can be helpful in defining the epileptogenic zone in patients who have a normal MRI (Guan et al. 2016; Pittau et al. 2014). MEG is a relatively newer diagnostic tool, but it’s efficacy in identifying the epileptic zone and association with good surgical outcomes is being established (Englot et al. 2015).
13.5.2 vEEG Monitoring
Imaging and initial scalp EEG are essential pieces of information to begin localizing seizure onset. However, the gold standard for characterizing seizures is inpatient video-EEG monitoring (vEEG). With vEEG monitoring, a patient is admitted for a minimum of 24 h to a neuromonitoring unit (NMU). Scalp electrodes are placed and the patient is monitored continuously. An NMU has patient rooms specially equipped to provide continuous EEG and video monitoring. A camera captures the clinical manifestations of seizures, while simultaneous continuous EEG captures the electrical activity of the brain during and between seizures. vEEG is an invaluable source of information, as it records not only the electrical onset and spread of the seizure but also the corresponding physical behaviors. Patients are monitored until they have had enough seizures to provide information regarding localization of seizure onset, spread, and duration. If need be, medications are withdrawn to help promote seizures. vEEG provides information regarding likely areas of seizure onset. It also allows for the correlation of behaviors seen during ictal and interictal activity. It can also identify seizure-like behaviors that are not a result of abnormal brain electrical activity (pseudoseizures).
13.5.3 Cognitive Evaluations
Another critical piece to the workup of a potential surgical candidate is neuropsychological testing. This involves various cognitive tests performed by a psychologist experienced in the needs and concerns of the pediatric epilepsy patient. One purpose of this testing is to establish a baseline of preoperative functioning. It also identifies problem areas that may be made worse by surgery. Identifying the presence of premorbid deficits can also help in making the decision whether to proceed with surgery. For example, if certain deficits that would be expected postoperatively already exist, that can be key information in the decision to proceed. Neuropsychological testing can also help to determine cerebral dominance for language and memory. However, if the patient is old enough to cooperate, a much more sensitive test for establishing dominance is the Wada test.
A Wada test, when indicated, can be used to help determine hemispheric dominance of language and memory. Depending on the proposed surgical procedure, this information is important in determining whether or not surgical resection would impair these functions. The procedure is technically a cerebral angiogram. The internal carotid is accessed with a catheter from the femoral artery, and a barbiturate, typically sodium amobarbital, is then injected. Patients are put through a series of age-appropriate language and memory tasks looking for impairment caused by the medication. If language ceases or memory is impaired while injecting one hemisphere, then dominance is established. If the seizure focus is felt to arise in that hemisphere, then the patient may be at more risk for language and cognitive deficits postoperatively. One limitation of this study is that patients must be old enough to participate as they must be awake for the actual testing. The arterial accessing can be done under anesthesia with reversal of agents prior to testing. As functional MRI has improved, it has been shown to be a noninvasive alternative to the Wada test in lateralizing language dominance for some patients (Dym et al. 2011). But it too has limitations related to age of the patient and cooperation. Additional limitations exist for both studies in children less than 10 years of age as language lateralization is not always identifiable in this age group (Schevon et al. 2007).
The goal of the preoperative evaluation is to attempt to identify the focus of seizure onset in relation to eloquent cortex for functions such as memory, language, cognition, and sensorimotor (Guan et al. 2016; Schuele and Luders 2008; Harvey et al. 2008). Data from the various sources is compared and analyzed in an attempt to “map out” eloquent cortex and seizure onset and their relationship to each other. Factors complicating this in children include incomplete functional maturation, level of patient cooperation, and the abnormal functional organization that can result secondary to malformed cortex and lesions (Yang et al. 2014; Schevon et al. 2007). Data gleaned from the various sources is reviewed and compared by the multidisciplinary epilepsy group. At times, the information is concordant with regard to localization of seizure onset, but other times it is not. More often, the information may lateralize to a single hemisphere but have imprecise or discordant data regarding specific localization of onset. For example, imaging may reveal a subtle abnormality in the left temporal lobe, but vEEG may suggest onset in the left frontal or parietal lobes. If all data is concordant and associated with a specific lesion, surgery may proceed without further testing. Conversely, if concordant data shows multifocal or bilateral onset, no further testing is needed because that patient is not a candidate for potentially curative surgery. A patient may, however, be a candidate for palliative procedures such as corpus callosotomy or vagus nerve stimulator. If the information obtained from a potential candidate for resective surgery requires further clarification, the patient will need additional invasive monitoring (phase II). Also, if the seizure onset lies near eloquent brain, invasive monitoring is indicted so that cortical mapping can occur.
13.6 Phase II Monitoring
Phase II monitoring involves direct recording from electrodes surgically placed in or on the brain. There are two main approaches to placing electrodes intracranially. One is through an open craniotomy for implantation of subdural strip or grid electrodes on the surface of the brain. The other is depth electrodes placed through individual burr holes. (Mullin et al. 2016a, b). Surgically implanted electrodes have been successfully placed in children of all ages, from infancy through adolescence. Which method to use, and where to place the electrodes, is dictated by the information obtained during Phase I monitoring. There are benefits and drawbacks to each method. The goal of Phase II monitoring is to define the epileptogenic zone and its relationship to eloquent brain as precisely as possible. Then the decision can be made as to whether or not the epileptogenic zone can be safely removed with minimal deficits and, if so, the likelihood of seizure freedom as a result. Regardless of the method used to place intracranial electrodes, one drawback is common to both: at least one more surgery is required to remove the electrodes.
Subdural electrode placement involves an open craniotomy with implantation of various grid and strip electrode configurations to cover a wide area over the suspected epileptogenic zone (Fig. 13.5). The exact number and configuration of electrodes used varies depending on the areas of the brain to be monitored. Typically, electrodes are only placed over one hemisphere (Fig. 13.6). However, single strip electrodes may be placed over the other hemisphere if there is concern for an area of onset in the contralateral hemisphere. Subdural electrodes are limited to placement on the surface of the brain; however, depth electrodes can be placed in conjunction to monitor suspected seizure foci deep within brain parenchyma.



Fig. 13.5
Subdural electrodes in place. Black arrow denotes large grid. Green arrow denotes a strip electrode

Fig. 13.6
Skull x-ray showing postoperative subdural electrode placement
The benefits of subdural electrode placement include the ability to monitor a large area of cortex. Subdural placement also allows the ability to perform cortical stimulation and mapping of eloquent areas such as language, sensorimotor, and memory (Yang et al. 2014) (Fig. 13.7). This method is often used when it is suspected that, if fully defined, the epileptogenic zone will be resected at the same time as electrode removal. Drawbacks to subdural placement include the need for a large craniotomy and the inability to adequately define an area of onset that may lie in deeper or contralateral cortex (Mullin et al. 2016a, b).


Fig. 13.7
Brain “map” showing eloquent functions in monitoring area
Depth electrodes are individually placed through small incisions and holes in the skull. These are typically used when the area of seizure onset is thought to be located in deep or difficult to access cortex. Depth electrodes are also used when prior workup has failed to clearly lateralize onset and bilateral monitoring is needed. Depth electrodes can help define the epileptogenic zone when subdural monitoring has failed to do so and in patients with difficult to localize epilepsy (Mullin et al. 2016a, b; Vadera et al. 2013; Gonzalez-Martinez et al. 2013) (Fig. 13.8). The benefits of depth electrodes include less invasive placement and the ability to monitor bilateral hemispheres, multiple lobes, and deep structures. Depth electrodes are limited in their ability to map eloquent cortex, though some studies have shown the ability to lateralize language using bilateral frontal electrodes (Alonso et al. 2016). Depth electrodes, like subdural electrodes, require additional surgeries, one more to remove the electrodes and an additional surgery if resection of the epileptogenic zone is undertaken.


Fig. 13.8
X-ray showing SEEG depth electrodes in place
Depth electrodes are typically placed stereotactically and are commonly referred to as SEEG (stereoelectroencephalography). The technique was developed in Europe in the 1960s, but did not become widely used in the United States until 2009 (Vadera et al. 2013; Gonzalez-Martinez et al. 2014). Stereotactic placement can be done using frameless stereotaxy or with traditional frame-stereotaxy (Fig. 13.9). Frame-based stereotaxy generally is more accurate but can be time intensive. More recently, robotic stereotaxy has come into use making the placement of SEEG leads faster, safer, and more accurate (Karsy et al. 2016). One such system is the ROSA™ (MedTech Surgical Inc., Newark, NJ) (Fig. 13.10). Electrode placement and trajectories are planned preoperatively using the system’s software and high-resolution imaging. Prior to surgery, fiducials are placed on the patient, and a new scan is obtained that is then fused with the preoperative planning scan. In surgery, the patient is “registered” to the robot, and the robot guides the exact location and trajectory of the electrode placement. The exact location and number of depth electrodes placed is guided by a predetermined hypothesis, based on Phase I workup, of where potential epileptogenic sites are located (Fig. 13.11) (Video 13.1).




Fig. 13.9
CT showing stereotactic frame in place.

Fig. 13.10
Rosa™ (MedTech Surgical Inc., Newark, NJ). Red arrow showing arm where robot attaches to patient head holder

Fig. 13.11
Planning software showing proposed electrode targets based on identified epileptogenic foci
Following electrode placement, the wounds are closed with the electrodes in place. The patient then undergoes continuous vEEG monitoring. This provides the most direct EEG monitoring without distortion caused by the meninges, skull, and scalp. Medications can be withdrawn if needed to induce seizures. Electrodes can be left in place for days to weeks in order to capture a sufficient number of seizure events to define the area of seizure onset and spread. Once enough seizures have been captured, the same electrodes can be used for direct stimulation of the brain and “mapping” of surrounding eloquent cortex if possible. This information is then used in planning the resection.
Depending on the location of onset, this information allows resection to occur safely without deficit or for appropriate planning with the family regarding what deficit to expect. At times, such mapping and monitoring will show that resection is not possible as too significant of a deficit will be inflicted. It is important that the area of seizure onset is established prior to undertaking cortical mapping, as mapping can trigger episodes of status epilepticus resulting in the need to administer AEDs.
13.6.1 Nursing Care of the Patient Undergoing Phase II Monitoring
If a craniotomy is performed, the patient will typically spend their first postoperative night in the PICU. Ideally, continuous EEG recordings should take place during this time. Often, patients will not experience seizure activity during this initial phase, but if they do it should be captured. If medically stable on postoperative day number one, the patient will be transferred to the NMU. Following SEEG placement, the patient can often go directly to the NMU. The NMU should be staffed by nurses who are not only proficient in caring for neurosurgical patients but also in recognizing and responding to seizure activity. NMU rooms are equipped with monitoring systems that alert nursing staff to seizure activity on the continuous EEG. Family members are also instructed to push an “alert button” when they feel that seizure activity is occurring. These patients are at risk for episodes of status epilepticus, especially as AEDs are weaned or when mapping is occurring. Each patient must have a clearly outlined rescue plan stating which AED is to be used, in what order, and after what length of time. With the risk of status epilepticus, all patients must have IV access throughout the monitoring process. Given the length of time often required, strong consideration should be given to placing a peripherally inserted central catheter (PICC) at the time of electrode placement.
Pain management is an important aspect of postoperative care. A combination of narcotic and non-narcotic medications seems to provide the best control, and nurses must be constantly sensitive to this. Narcotics typically do not deter seizures from occurring and need not be withheld. All patients are different in their tolerance of implanted electrodes. Some patients experience pain that is easily controlled, resume normal diets, and have fairly uneventful postoperative courses. Other patients may have poorly controlled pain, experience protracted nausea and vomiting, or display irritability and some degree of cognitive depression throughout the entire time the electrodes are in place. These side effects are seen more commonly with subdural grid and strip electrodes, as the risk of cortical irritation and swelling is greater given a larger area of the brain is in contact with the electrodes. When this occurs, a short course (24–72 h) of dexamethasone may be used in the initial postoperative period and may need to be extended in some cases.
In some centers, following a craniotomy to implant electrodes, the bone flap is left off. It is important for all caregivers to be alert for this and avoid pressure or trauma to that side of the head. Drainage from the incision while the electrodes are in place is not uncommon and can vary greatly from large to small amounts on a daily basis. The need for prolonged antibiotics with implanted electrodes is a matter of surgeon preference but largely is not done. Most children inherently leave their leads alone, but restraints may be needed if wound and electrode integrity are threatened. EEG monitoring is continuous and should not be disconnected unless an absolute emergency warrants it. As such, the patient is restricted to the bed and immediate surrounding area. This can be challenging for the patient and family, and distractive activities should be employed. Careful attention to developmental and emotional needs is essential. Family members are encouraged to stay with the patient and be an active member of the team. The participation of therapists, child life specialists, school specialist, and other ancillary services is invaluable for the overall success of the monitoring process (Fig. 13.12).


Fig. 13.12
Patient with implanted subdural electrodes
13.6.2 Complications of Cortical Electrodes
There are risks with surgically implanted electrodes. The electrodes themselves are thin and pliable and are generally well tolerated. However, especially in the immediate postoperative period, there is the risk of acute hematoma formation, cerebral edema, vascular compression, and inflammation (Mullin et al. 2016a, b; Gonzalez-Martinez et al. 2013). Any of these can cause varying degrees of mass effect on the brain, resulting in increased intracranial pressure, and possible need for emergent return to the OR for electrode removal. Complications such as these are uncommon and typically seen only in the first 24–48 h postoperatively. A meta-analysis review of the complications seen with SEEG and subdural electrode placement showed that the risk of these complications was less in SEEG (Mullin et al. 2016a, b). There exist small risks of infection and cerebral spinal fluid leak. There is a risk that electrodes will be implanted – only to show that resective surgery is not possible due to multiple areas of onset, seizure onset in eloquent cortex, or seizure onset outside the area being monitored. It is possible that despite withdrawal of medication and various activities to induce seizures, seizures may not occur during the monitoring period. Implanted electrodes can be left in place for up to 3–4 weeks, but concerns for infection or complications may warrant removal sooner. Status epilepticus may occur as AEDs are withdrawn or during mapping.
13.7 Epilepsy Surgery
Once the focus of seizure onset has been identified and surrounding brain has been mapped, if necessary, decisions are made regarding the type of surgery that can be offered. Generally, there are two types of epilepsy surgery, potentially curative and palliative. Potentially curative procedures are offered to those with onset within one hemisphere. The exact procedure offered is dependent not only on the area of seizure onset but also on the underlying cause of the epilepsy. Potentially curative procedures include lesionectomy, temporal lobectomy, extratemporal resections, and hemispherectomy. Individuals who have bilateral or multifocal onset are not candidates for potentially curative surgery. However, they may be candidates for palliative procedures, including corpus callosotomy or vagus nerve stimulator.
13.7.1 Lesionectomy
A lesionectomy is the removal of a well-defined lesion, such as a tumor, vascular malformation, or hamartoma, which has been shown to be the site of seizure onset. Resection may take place with or without phase II monitoring. Phase II monitoring may be needed if there is concern for potential seizure onset in the surrounding cortex, if the margins of the lesion are not clearly defined, or if the lesion’s location does not fully explain seizure semiology. Phase II monitoring also allows for cortical mapping so that potential postoperative deficits can be fully understood and avoided if possible. The most common tumor types seen in association with intractable epilepsy are DNET (dysembryoplastic neuroepithelial tumor), ganglioglioma, and astrocytoma, but any tumor can be epileptogenic. Benign tumors that may not require resection from an oncology perspective may need resection in order to treat intractable epilepsy. If a tumor is present, but seizures are well controlled on easily managed and well-tolerated AEDs, resection may not be necessary. Individuals undergoing epilepsy surgery for a well-defined lesion have the highest likelihood of becoming seizure free (Guan et al. 2016; West et al. 2016; Kunieda et al. 2013; Tellez-Zenteno et al. 2010) (Fig. 13.13).


Fig. 13.13
T2 (left)- and T1 (right)-weighted MRI images showing epileptogenic lesion (ganglioglioma) in left posterior parietal lobe as indicated by the arrows
Risks specific to a lesionectomy include injury to eloquent brain. The exact deficits seen depend on the area of brain affected. There is also a risk of incomplete resection of epileptogenic zone resulting in continued seizures. This is more likely if the lesion is not clearly defined, lies close to eloquent cortex, or if it is associated with other unseen abnormalities like mild cortical dysplasia. Results from a lesionectomy may be improved with intraoperative electrocorticography which allows direct recording from the brain to identify areas of abnormal electrical activity near the lesion that may be epileptogenic.
13.7.2 Temporal Lobectomy
Temporal lobe epilepsy (TLE) is one of the most common epilepsy syndromes amendable to surgical treatment. While it accounts for up to 75% of all surgically treated epilepsy in adults and adolescents, it accounts for only 15–20% in children (Englot et al. 2013). Surgery for TLE dates back to 1886. Because of this and its commonality, it is one of the most studied forms of epilepsy surgery (Ramey et al. 2013; Rzezak et al. 2014; Velasco and Mather 2011). As research has progressed, and more has been learned about underlying pathology, it has become clear that pediatric and adult TLE are quite different. Mesial temporal sclerosis (MTS), particularly hippocampal sclerosis, is the most frequent pathology found in intractable TLE in adults (Deleo et al. 2016; Guan et al. 2016; Velasco and Mather 2011). Mesial temporal structures include the hippocampus, parahippocampal gyrus, and the amygdala. Sclerosis is scarring and atrophy secondary to neuronal loss that can be caused by such insults as infection, traumatic injury, or hypoxic events. In pediatric TLE, the pathology is more likely to be low-grade tumors (ganglioglioma or DNET) or cortical malformations, primarily dysplasia. Another difference in pediatric TLE is the high prevalence of dual pathology. Dual pathology refers to seizures arising from mesial temporal structures as well as from the surrounding temporal cortex and adjacent structures (Ryvlin and Rheims 2016). Dual pathology, or “temporal plus epilepsy,” is much more common in children than in adults (Guan et al. 2016; Ryvlin and Rheims 2016). Dual pathology may not always be readily evident during the presurgical workup. Seizures arising within the temporal lobe spread quickly to involve all of the temporal lobe, medial structures, adjacent lobes (frontal and parietal), and the contralateral temporal lobe, making the exact identification of ictal onset difficult. Different techniques are used for temporal lobectomy. Resection can involve just the mesial structures (amygdalohippocampectomy) with or without varying degrees of temporal lobe resection, from full to partial (Guan et al. 2016; Ramey et al. 2013). The extent of resection can also vary depending on whether or not dominance has been established. The temporal lobe plays a significant role in language, and if the child is old enough for dominance to have been established, a more limited resection of the lateral posterior temporal lobe will be done on the dominant side. Outcomes for temporal lobectomy are generally very good in both children and adults, with reported rates of seizure freedom ranging from 60 to greater than 90% (Englot et al. 2016; Guan et al. 2016; Lee et al. 2015; Ramey et al. 2013). Rates are slightly lower in children compared to adults. This is likely related to the frequency of dual pathology and greater difficulty in completely defining the epileptogenic zone.
13.7.2.1 Complications of Temporal Lobe Surgery
The temporal lobe is responsible for many memory and language functions. Therefore, potential complications following temporal lobe surgery involve deficits in these areas. The temporal lobe is the location of the primary auditory cortex and plays a role in auditory perception. Postoperatively, patients having undergone a temporal lobectomy are at risk for problems with comprehension of verbal stimuli, naming, and verbal memory impairment. The hippocampus is involved in long-term memory and in making new memories, so impairment can be seen there as well. In the younger child, language is less likely to be affected or may recover more quickly, as language rarely completely lateralizes before age 10 (Lee et al. 2015; Englot et al. 2015). In individuals where the anterior temporal lobe has been resected, a partial homonymous superior quadrantanopia can be expected postoperatively secondary to disruption of the optic radiations (Lee and Adelson 2004). Sometimes referred to as the “pie in the sky” defect, this visual field cut involves the left or right upper quadrant in each eye and affects the side opposite of the resection. For example, a right temporal lobectomy will result in a defect in the left upper quadrant in both eyes. There is also a risk of CN III (oculomotor) injury resulting in impaired eye movements and a ptosis, as medial temporal structures lie immediately adjacent to the third nerve at the tentorial edge (Holmes 2002).
13.7.3 Extratemporal Resections
The temporal lobe is the most common site of seizure onset in adults, but extratemporal onset is more common in children (Englot et al. 2013). Extratemporal lobe epilepsy (ETLE) can include the frontal, parietal, or occipital lobes. The most common pathology seen is malformations of cortical development, particularly cortical dysplasia. ETLE is also involved in multifocal epilepsy syndromes such as Sturge-Weber and tuberous sclerosis complex (Guan et al. 2016; Ramey et al. 2013). Resection of the seizure focus can involve a full or partial lobectomy as well as portions of multiple lobes, depending on the causative pathology and its location. Seizure onset in extratemporal epilepsy typically occurs early in life, often in infancy, and can be quite severe. Therefore, extratemporal resections account for the largest number of epilepsy operations among younger children (Vendrame and Loddenkemper 2010).
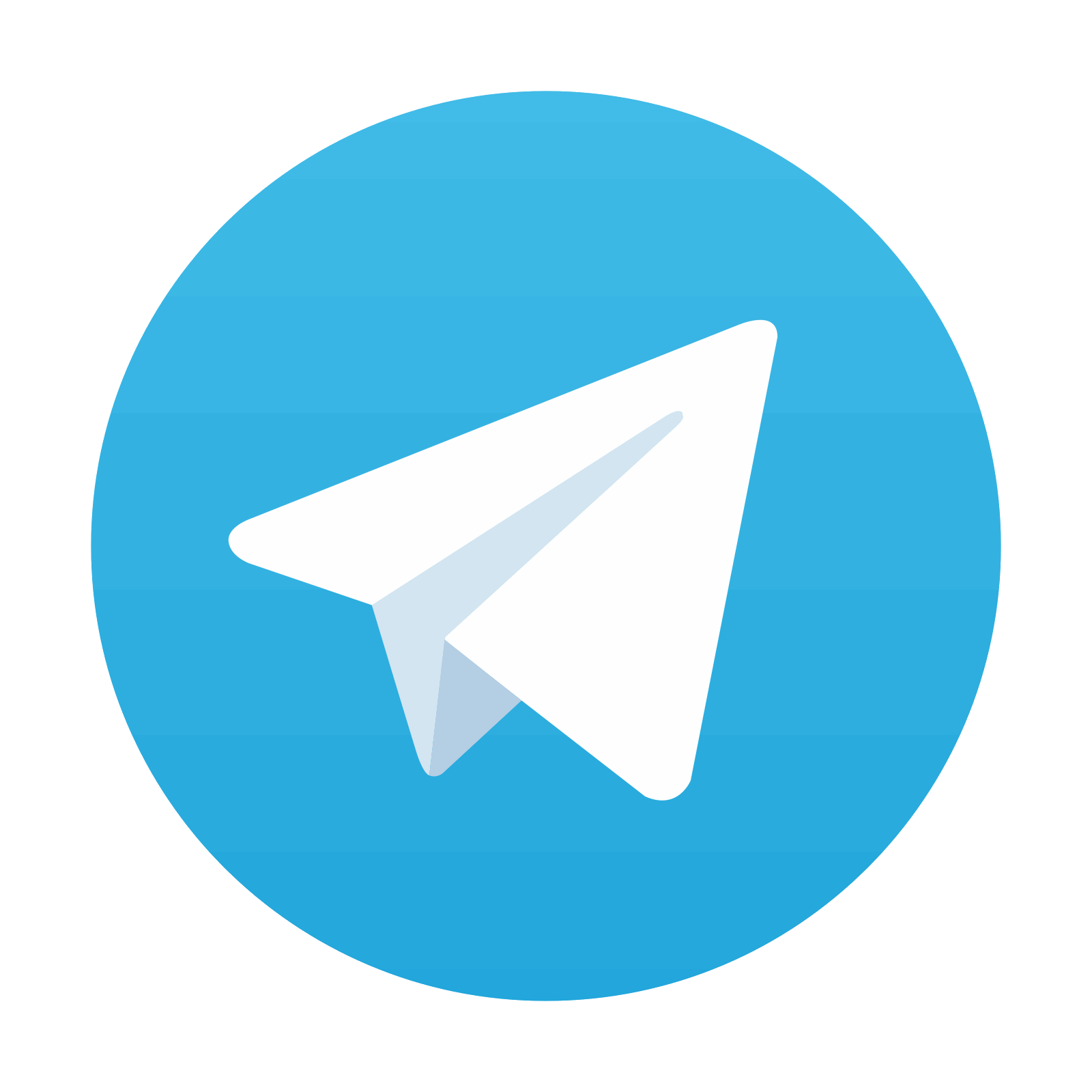
Stay updated, free articles. Join our Telegram channel

Full access? Get Clinical Tree
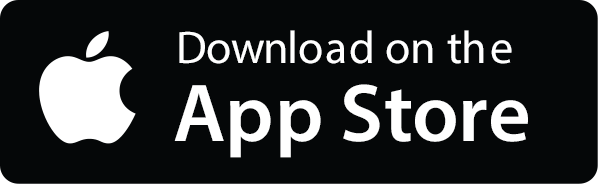
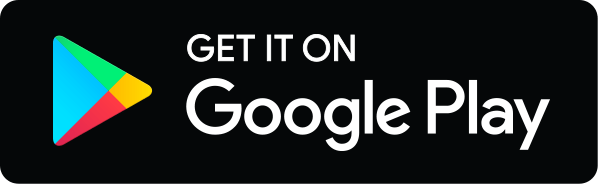