Fig. 11.1
Defects of segmentation
11.3.1 Etiology
Development of the vertebrae begins at 4–6 weeks of gestation. This is when the mesenchymal mold is formed which is the model for the framework for the secondary cartilaginous and osseous development of the vertebrae (Dewald et al. 2003). Subsequent chondrification and ossification follow this mold. The neural axis is developing at the same time, which explains why children with vertebral anomalies may potentially have neural anomalies as well. The embryologic insult resulting in the vertebral anomaly is unknown, and there has been no clear-cut genetic etiology of congenital scoliosis to date. In animal models, it has been demonstrated that deformities have been linked with maternal hypoxia at the critical time of gestation (Devlin 2012). There have also been associations with maternal diabetes, ingestion of antiepileptic drugs during pregnancy, and maternal exposure to toxins (Hedequist and Emans 2007).
Congenital vertebral anomalies are rare. The prevalence rate of congenital scoliosis is approximately 1 in 1,000 live births (Hedequist and Emans 2007). Isolated anomalies (hemivertebra) are sporadic with no familial or genetic tendencies. However, there is a 5–10% risk of similar lesions in siblings or subsequent generations, as well as an increased risk of neural tube defects when there are complex anomalies in multiple locations (Dewald et al. 2003).
11.3.2 Related Conditions
Organ systems that are developing during the same gestational period as the spine may also be at risk for developing malformations. There are some common malformations that are associated with congenital spinal anomalies, including intraspinal abnormalities. Neural axis abnormalities, such as tethered cord, spinal stenosis, diastematomyelia (split cord), diplomyelia (complete or incomplete doubling of the spinal cord), and syringomyelia (fluid-filled space within the spinal cord), are present in up to 38% of patients with congenital vertebral anomalies. Clinical findings of posterior midline skin lesions like hairy patches or dimples, foot deformity (especially unilateral), muscle weakness, or spasticity may be a red flag as to an underlying intraspinal anomaly. Other anomalies associated with vertebral abnormalities are vertebral anomalies at another level, urinary tract structural abnormalities, cranial nerve palsy, upper extremity hypoplasia, clubfoot, dislocated hip, and congenital cardiac disease. Specifically, the vertebral malformation most often associated with an abnormality of the neural axis is a unilateral unsegmented bar and a same-level contralateral hemivertebra. It has been estimated that approximately 50% of this population have an associated neural axis abnormality (Devlin 2012). These may often be occult anomalies such as a tethered cord, intradural lipoma, syringomyelia, or diastematomyelia, which is the most common (Dewald et al. 2003). The clinical signs and radiographic findings of these spinal cord anomalies are discussed in the physical assessment and imaging sections of this chapter.
11.3.3 Evaluation of the Child with a Congenital Spine Deformity
As high as 35% of children with a congenital spine deformity may also have a congenital anomaly of the neural axis or a spinal dysraphism. Some of the forms of spinal dysraphism include spina bifida (spina bifida occulta, meningocele, and myelomeningocele), diastematomyelia, and tethered cord (Hedequist and Emans 2007). The symptoms of spinal dysraphisms are difficult to generalize as they are quite varied depending on the type and severity of the malformation. A thorough past medical history with focus on the physical exam should be obtained, keeping in mind the correlation of the congenital spine deformity and other congenital anomalies. Detailed questions should be directed as to the child’s neurologic status. Age-appropriate questions related to development history, toilet training, bed wetting, changes in bowel or bladder habits, and complaints of lower extremity pain, numbness, or weakness are all of great importance in the evaluation of the child’s neurologic status. A complete evaluation of the patient should include a comprehensive exam of the patient as well as a close evaluation of the spine itself with a thorough neurologic exam.
General patient evaluation should occur throughout the encounter with the child. A full exam should include sitting and standing heights or supine measurements for infants and head circumference. Take note of any alterations in head shape, along with size, shape, and symmetry of the ears and eyes. Assessment of the neck and cervical spine should be noted for range of motion. There are some syndromes, such as Klippel-Feil syndrome, which have congenital cervical fusions thus limiting cervical range of motion. The spine exam itself should focus on trunk and pelvic balance, including the coronal and sagittal planes (Hedequist and Emans 2007). The finding of abnormal kyphosis on physical exam may be postural or congenital and thus should be thoroughly evaluated. Complaints of back pain, muscle fatigue, and stiffness are common in kyphosis. In severe cases, there can be compression of the spinal cord with neurologic symptoms that include weakness, altered sensation, and loss of bowel and bladder control. The chest should be examined for symmetry and deformity. Observation of inspiration, expiration, and the abdominal wall for movement, masses, and hernias may be an indication of a fixed chest wall deformity (Dewald et al. 2003). Lastly, secondary sexual development should be noted with examination of the genitalia and Tanner staging.
11.3.4 Neurologic Evaluation
Neurologic evaluation can quickly and easily be performed throughout the physical exam. Extremity development, length, and symmetry should be noted, as well as anomalies of the feet. Feet abnormalities may include extra or absent toes, cavus (high arch), or flat feet. Remember that flat feet alone may be a benign common finding in young children. These can be red flags as to underlying neurologic conditions. Congenital deformities of the upper body and extremities such as Sprengel’s deformity (undescended scapula) and syndactyly should also be noted (Fig. 11.2). Further neurologic exam can be performed simply by observing the child walk, run, and move, noting overall general motor function. Testing of reflexes should be carefully performed. Abdominal reflexes should be symmetric. Asymmetric or depressed reflexes can be symptomatic of a spinal dysraphism. Hyperactive reflexes can be indicative of spinal cord compression (Dewald et al. 2003).


Fig. 11.2
A 4-year-old boy with Sprengel’s deformity associated with Klippel-Feil syndrome
11.3.5 Associated Anomalies
Children with congenital scoliosis are also at risk for abnormalities of the cardiac and genitourinary systems. Congenital heart disease may be observed in up to 25% of children with congenital scoliosis and genitourinary anomalies in as many as 20% of these patients (Hedequist and Emans 2007). These abnormalities may be benign and asymptomatic. They are typically detected during a routine screening or preoperative appointment. Alternatively, these anomalies may be severe and have possibly already required treatment. If it is determined through the medical history that these patients have not previously been evaluated for possible cardiac and/or urologic anomalies, they should be referred to a cardiologist or urologist for further screening and evaluation.
11.3.6 Diagnostic Studies
When anomalies of the spine are suspected, radiologic study is essential, especially for anomalies of the cervical spine. Early identification may significantly reduce potential major complications (Albright et al. 1999). Plain x-rays are always a recommended and reliable starting point for radiographic evaluation; however, they may give limited information depending on the age of the patient and the type of anomaly present.
Ultrasound, a simple and very useful type of study, can be a good screening tool in neonates and infants when physical findings suggest an underlying dysraphic lesion. Prenatal findings, family history, and other anomalies may also be an indication for an ultrasound (Albright et al. 1999). Normal vertebral growth and ossification can demonstrate many patterns, as well as be difficult to read on plain films due to the many overlying structures. CT scans are often needed to assess congenital anomalies. This is true especially in the cervical spine. Both CT and MR are especially useful in studying the entire spinal cord and the cervical spine. If any anomaly or malformation is suspected, it is important to image the entire spine as multiple spinal anomalies can commonly present. (Albright et al. 1999).
The incidence of intraspinal abnormalities associated with congenital spine anomalies as detected by MRI has become better defined. In a study by Suh, Sarwark, Vora, and Huang in 2001, there was an incidence of intraspinal anomalies in 31% in children with congenital spine anomalies. A screening MRI is indicated in patients with a spinal deformity that is known to be associated with neural axis abnormalities because of its capacity to detect clinically unsuspected dysraphia in asymptomatic patients. Other indications would include patients with a progressive deformity, abnormal reflexes, neurological deficits, or major extremity anomalies (Hedequist and Emans 2007). Angiography may be required if vertebral circulation is a concern. Some of the intraspinal pathologic conditions that may be found in patients with such conditions include congenital spinal stenosis, tethered cord, syrinx, Chiari malformation, diastematomyelia, and spinal cord tumor (Devlin 2012). Again, as clinical manifestations may not be detectable initially, MR imaging of the entire spinal cord and vertebral column from the foramen magnum to the distal sacrum is essential for complete evaluation. The advantages of MR imaging is that it is a technique that avoids invasive procedures and high radiation exposure.
All systems should be thoroughly reviewed and examined. Frequently, a renal and cardiac consultation is a part of an expanded evaluation.
Computed tomography (CT) is not typically used for serial monitoring and observation of scoliosis; however, it is routinely used in preoperative assessment and planning. CT scans are extremely useful in clearly defining the anatomy and identification of previously unrecognized malformations (Hedequist and Emans 2007). There are concerns regarding high radiation exposure during a CT scan. Today, most institutions typically have written protocols for various scans in order to minimize the amount of radiation that children are exposed to during their scans.
Treatment of congenital scoliosis focuses on early recognition, diagnosis, and treatment. Surgical treatment may include early arthrodesis and the routine use of spinal instrumentation for progressive curves. Younger children with congenital deformities may be treated with fusionless surgery by using a growing rod technique. Further discussion of various surgical corrections, fusion, and instrumentation is beyond the scope of the chapter.
11.3.7 Skeletal Dysplasias
All conditions of skeletal dysplasia share a common theme of some systemic defect in skeletal development. The categories of spine-related problems common to many of the skeletal dysplasias are instability, stenosis, and deformity, although they may occur concurrently at different levels of the spine and in different planes. Any type of dysplasia may manifest one or more of these problems with some exceptions. The phenotypic variability seen in genetic diseases makes it difficult to predict specific patterns of problems within the skeletal dysplasia groups (Dewald et al. 2003).
The first issue of instability may be a bony or ligamentous issue and usually occurs at the C1–C2 level. Instability is most common in the mucopolysaccharidoses, spondyloepiphyseal dysplasia, and metatropic dysplasia (Dewald et al. 2003). Baseline flexion and extension films of the cervical spine are always indicated. Also, a clinical exam evaluating spasticity, strength, and coordination should be included. An MRI in neutral, flexion, and extension would be indicated if it is not clear whether cord compression is developing. Stenosis may occur at any level of the spine. It is most commonly found in the patient with achondroplasia (80–90%) (Dewald et al. 2003). Treatment, typically decompression of the affected region, is determined by the extent and location of the neurologic deficit (Fig. 11.3 and Box 11.1).


Fig. 11.3
A young lady with Larsen syndrome, a connective tissue/skeletal dysplasia disorder associated with congenital spinal instability and spinal cord injury. This young lady went on to have a posterior cervical fusion as well as spinal fusion from T2 to L4 for her cervical kyphosis, cervical myelopathy, and progressive lordoscoliosis. She mostly mobilizes with her wheelchair but is able to walk with a walker
Box 11.1 Our Angel
This story begins the same as many, following the joyous birth of an expected-to-be-healthy child. The delivery was a bit challenging, but it was well worth knowing we had a beautiful baby girl! Immediately, grandmother noticed that the baby had unusually long fingers and hands, but we were not concerned at first. We also noticed that she was not meeting developmental milestones, even the simple ones like being able to hold her head up. This was the beginning of our journey, meeting with many specialists and undergoing multiple examinations. Finally, a geneticist identified her problem, and it was called Larsen syndrome or Larsen’s syndrome.
We learned that this is a very rare diagnosis that is one of a group of conditions known as “skeletal dysplasias.” It is an inherited condition characterized by congenital interruption of multiple body joints and connective tissue. The children have a characteristic appearance of the face, hands, and multiple skeletal problems. They may have learning disabilities as well.
Identifying the diagnosis was just the beginning. We had to find out who could provide the very best care for her. We began by going online and reaching out to other parents. We found specialists in orthopedics who could stabilize her spine that was compressed by almost 97%! She underwent a fusion and was placed in halo traction for the first 4 years of her life.
We have never lost faith that our little girl would grow up – she is now a freshman in high school! You can see by her beautiful picture that she cares about all the things that most teens do. She chose the picture because she wanted to look her best.
We do have a wonderful support system in place that includes our family, our church, and our many friends. We also have sought out what we feel is the best care possible with the help of other parents from around the country. We have also counted on the surgical specialists, the orthopedic and neuroscience nurses, the many therapists, and her schools to guide and support us.
Most important of all throughout her 14 years of life, the resilience of our daughter and granddaughter has endured. No challenge is too great, no obstacle too much for us to overcome. We have come together and endured it all for our “little angel” because she is worth it all.
11.4 Larsen Syndrome
Larsen syndrome is a heterogeneous, rare genetic disorder occurring in approximately 1 in 100,000 births. It was first recognized as an entity by Larsen in 1950 (Hennekam 2010). It is known to follow an autosomal-dominant or autosomal-recessive mode of inheritance, although sporadic occurrences have also been described in the literature (Becker et al. 2000).
Symptoms and severity in Larsen syndrome may vary greatly, ranging from a lethal form of the disorder to a mild clinical expression where there is an absence of major diagnostic features. Characteristic findings include skeletal and large joint abnormalities with distinctive facial features, cleft palate, hearing loss, and spinal abnormalities. Classic facial features of the syndrome are flattened, hypoplastic midface, a prominent forehead, depressed nasal bridge, and hypertelorism (Becker et al. 2000). Some symptoms are present at birth such as dislocation of large joints, especially the hips, knees, and elbows. Club foot is present in 75% of affected individuals. Spine abnormalities occur in about 84% of those with Larsen syndrome. This includes scoliosis and cervical kyphosis. Cervical kyphosis can occur from subluxation or fusion of the cervical vertebral bodies associated with dysplasia of the vertebral laminae and hypoplasia of the lateral processes of the cervical vertebrae. This is potentially the most life-threatening manifestation due to the impingement of the spinal cord at the apex of the kyphosis (Johnston et al. 1996). Early diagnosis and intervention of cervical kyphosis can help patients avoid potential neurologic deficits.
11.5 Loeys-Dietz Syndrome
Loeys-Dietz syndrome (type 1–5), also referred to as LDS or Marfan 2, is a rare genetic disorder. The exact prevalence of LDS is unknown. Its characteristic features of craniofacial, vascular, and musculoskeletal malformations are also common to Marfan syndrome. Although LDS has been called Marfan 2, there are some significant differences between Loeys-Dietz and Marfan syndrome (The Marfan Foundation 2016).
Loeys-Dietz is an autosomal-dominant connective tissue disorder. It is caused by a mutation in the transforming growth factor-beta receptor 1 or 2 (TGF-BR1 or TGF-BR2) genes (Fuhrhop et al. 2015). Loeys-Dietz syndrome, first described in 2005, is characterized by a triad of aggressive aneurysm formation, bifid uvula or cleft palate, and hypertelorism. Other common physical features in Loeys-Dietz include translucent skin, easy bruising, and craniofacial abnormalities such as craniosynostosis, retrognathia, and malar hypoplasia. Vertebral anomalies and cervical instability also have a high prevalence in Loeys-Dietz syndrome. Cervical instability especially at C2–C3 is associated with C3 vertebral body hypoplasia and C2–C3 kyphosis (Fig. 11.4). Other spine malformations may include scoliosis and spondylolisthesis. Deformity of the extremities including arachnodactyly, talipes equinovarus, and joint hypermobility are also noted. Pectus excavatum or carinatum deformities may also be present (Fuhrhop et al. 2015).


Fig. 11.4
Preoperative x-ray of a 12-year-old male with Loeys Dietz. Note the cervical kyphosis
As evidenced by the literature, cervical midline defects are common in Loeys-Dietz syndrome as are vascular abnormalities. With these patients having a high prevalence of cervical instability, there is a very important need for frequent imaging to monitor for progression and subsequent neurologic sequelae from the deformity (Fig. 11.5). Early diagnosis is critical for appropriate and timely intervention (Fuhrhop et al. 2015).


Fig. 11.5
Preoperative MRI demonstrates stenosis and compression of the spinal cord
Box 11.2 Devin’s Story
(Told by Devin’s mother)
Our story began the same as many, however changed and became a high-risk pregnancy. Many tests were being done in the last 3 months of the pregnancy. We were never told anything was wrong with our sweet baby boy. The delivery was like many others, but we only got a few minutes to see our son before he was taken to the NICU (neonatal intensive care unit.) After 11 days of being in NICU, our baby was able to come home, with a lots of issues and doctors.
After 7 years of his life, we requested a test to be done on our son to see if he has Loeys-Dietz syndrome. The geneticist looked him over to see if he met the criteria and she believed he did. Two weeks later, we finally had a diagnosis. After many appointments and surgeries with many doctors and specialists, we then knew we were dealing with Loeys-Dietz syndrome (Fig. 11.6).


Fig. 11.6
12 year old male with Loeys-Dietz
Our son was born with many skeletal issues from his head to his feet, ligament issues in his knees and ankles, and an enlarged aorta. After three head surgeries, his skull was reshaped (due to having two soft spots at birth), two jaw surgeries to pull his jaw forward (the jaw was pushed back and crushing his airway), one heart surgery to replace the section of the aorta that was enlarged, and two neck surgeries due to two extra vertebrae, causing the neck to curve and smash his spinal cord (Fig. 11.7). Both knees had to have ligaments tightened to stop dislocating. A screw was placed in his left foot to help straighten it, and through all of these bad experiences, he still has a great outlook on life! He is an inspiration to all of us when we think that our own problems are bad!


Fig. 11.7
Note postoperative spinal fixation extending into the cervical spine to correct the preexisting kyphosis and instability
11.6 Spinal Manifestations of Achondroplasia
Achondroplasia is an autosomal-dominant genetic disorder affecting endochondral bone formation. It is the most common form of short-limbed dwarfism. The prevalence rate is approximately 1 in 26,000 live births (Wright et al. 2000). The mutation of the gene which is involved in the growth and development of bone results in abnormal stunting of bone formation at the epiphyseal plates (Alman 2002; Shiang et al. 1994). The bones in the face, skull base, spine, hands, feet, and proximal long bones, such as the humerus and femur, are especially affected resulting in characteristic features involving these structures (Fig. 11.8) (Alman 2002; Poseti 1988). Hydrocephalus, upper cervical spinal cord compression, and spinal stenosis are three neurological problems seen in achondroplasts (Park et al. 2003). The spinal manifestations of achondroplasia will be the primary focus of this section.


Fig. 11.8
Twins, one with achondroplaisa. Notice the classic physical features of achondroplasia
Abnormal development of the bones of the skull base results in abnormalities of the foramen magnum, posterior fossa, and brain stem. These abnormalities contribute to compression at the cervicomedullary junction. The diameter of the foramen magnum is significantly decreased in achondroplasia leading to compression of the upper cervical spinal cord and distal medulla (Schnuerer et al. 2001). The foramen magnum is also displaced anteriorly resulting in a shallow posterior fossa. As a result, the brainstem is displaced upward and posteriorly, simulating hyperextension of the neck. The compression on the upper cervical spinal cord is exacerbated further because it is often stretched over the edge of the occipital bone (Kokoska et al. 2001; Kopits 1988).
11.6.1 Cervicomedullary Compression
Cervicomedullary compression resulting from foramen magnum insufficiency is usually identified in young achondroplastic patients and is a common cause of early morbidity and mortality in this patient population. It is also thought to be a contributing factor in the hypotonicity and motor developmental delay common in achondroplastic infants. The manifestations of this chronic neurological compression can be wide ranging. Patients may be asymptomatic initially but may develop signs and symptoms of upper spinal cord compression, including paresthesias and paresis, which can be progressive, even leading to quadriplegia. Signs of myelopathy including hyperreflexia and clonus may be evident even in the patient who is apparently asymptomatic. Symptoms such as spastic quadriparesis may not manifest until adulthood in patients with mild, chronic compression. Respiratory manifestations such as hypoventilation and apnea may also occur because of medullary compression. Simple extension of the neck can cause acute compression of the spinal cord at the cervicomedullary junction which could lead to sudden death of the achondroplastic infant (Hunter et al. 1998; Hurko et al. 1988; Morgan and Young 1980; Reid et al. 1988). The foramen magnum eventually increases in size as the child grows, easing the compression at the cervicomedullary junction (Rimoin 1988).
Radiographic evaluation should be undertaken if there is any question of cervicomedullary compromise. Computed tomography (CT) can provide detailed imaging of the bony architecture of the skull base, foramen magnum, and upper cervical spinal column. Measurements of the foramen magnum can be taken from these scans to determine the degree of stenosis. Magnetic resonance imaging (MRI) provides information on the soft tissue structures including the brain stem and upper cervical spinal cord. Compression of these structures is evident with this study and changes within the upper cervical spinal cord can be identified, including atrophy and abnormal signal within the spinal cord itself (Fig. 11.9) (Hurko et al. 1988). Any patient who exhibits respiratory abnormalities should undergo a thorough evaluation to determine if there is a neurologic component to their respiratory dysfunction. Additional studies which may be helpful in evaluation of these patients include somatosensory evoked potentials to evaluate brain stem and spinal cord dysfunction and sleep apnea studies to rule out central versus obstructive apnea (Hurko et al. 1988; Reid et al. 1988).


Fig. 11.9
MRI of patient with achondroplasia. Note the upper cervical cord compression and spinal stenosis
Caution should be exercised in positioning the achondroplastic infant until better control of the head and neck muscles develops. Any position or activity that results in hyperextension of the neck could cause additional impingement of the spinal cord and distal medulla at the craniocervical juncture. Sitting or standing postures without adequate support of the neck and head should be avoided, as well as the use of bouncing type seats (Hurko et al. 1988).
11.6.2 Thoracolumbar Kyphosis and Lumbosacral Hyperlordosis
Abnormal alignment of the spine, including kyphosis in the thoracolumbar junction and hyperlordosis at the lumbosacral junction, is also frequently seen in this patient population and contributes to the congenital spinal stenosis common in achondroplasia. Kyphosis is very common in the achondroplastic child, with more than 90% of achondroplastic infants under 1 year of age exhibiting a kyphotic deformity (Pauli et al. 1997). Truncal hypotonicity, ligamentous laxity, the large size of the infant’s head, flat chest, and protuberant abdomen are thought to be contributing factors to the development of this deformity. Abnormalities of the vertebral body, such as wedging at the apex of the kyphotic deformity, may be evident in some, but not all, of these patients (Ain et al. 2004). Many children will have spontaneous improvement in this deformity once they begin to sit upright and walk; however, up to one-third of these patients will develop a progressive kyphosis into adulthood (Ain and Shirley 2004; Lonstein 1988; Pauli et al. 1997).
Hyperlordosis of the lumbosacral juncture, present in children with achondroplasia, becomes evident as the achondroplastic child begins to walk. The incidence of this deformity increases as these children reach adulthood. This deformity is not thought to be a primary disorder of the spine but rather occurs as a result of abnormal forward flexion of the pelvis and a horizontally oriented sacrum (Giglio et al. 1988; Kopits 1988).
Patients with thoracolumbar kyphosis and hyperlordosis of the lumbosacral spine have a very distinct posture. The kyphotic deformity is most notable while patients are in the seated position. The spine assumes a C shape and the patient appears to sit with a slumped posture (Reid et al. 1988). In the standing position, the kyphotic deformity becomes much less noticeable, but exaggeration of the lordotic curve becomes evident. Plain, lateral view x-rays illustrate these abnormalities well (Fig. 11.10).


Fig. 11.10
Lumbar kyphosis
Parents of achondroplastic children should be instructed to avoid having the child sit in an unsupported position until truncal hypotonia improves. The back should be supported utilizing counterpressure with the hand when the child is held, and only hard-backed seating devices should be used. Periodic radiographic follow-up is important during this time to assess for continued progression of the kyphosis. If the kyphosis is determined to progress or if vertebral body wedging is evident, then bracing utilizing a firm brace such as a thoracolumbosacral orthosis (TLSO) can be instituted (Kopits 1988; Pauli et al. 1997). In many patients, the kyphotic deformity will improve with these measures; however, approximately 11% of these patients will develop persistent and progressive kyphosis (Ain and Shirley 2004; Kopits 1988). In these cases, surgical intervention involving stabilization with fusion and instrumentation is often necessary. Surgery of this nature in the achondroplastic patient carries a significant risk of neurologic injury (Ain and Shirley 2004).
11.6.3 Spinal Stenosis
The overall diameter of the spinal canal in achondroplastic patients is diminished as a result of abnormalities in the development of the bone of the spinal column.
Symptoms related to spinal stenosis below the cervicomedullary juncture usually do not become problematic until the late teens. In most cases, symptomatic spinal stenosis does not occur until later in adulthood with the average age of symptom onset occurring late in the third decade of life (Ain et al. 2000; Hunter et al. 1998; Hurko et al. 1988). Low back pain is a very common complaint in patients with achondroplasia. Neurogenic claudicatory-type symptoms are typical in patients with symptomatic spinal stenosis. These symptoms include pain, paresthesias, and weakness involving the lower extremities with walking. The symptoms are often relieved by rest, bending forward, or squatting. A typical scenario is the patient who must lean on a shopping cart in order to complete the task of grocery shopping. The symptoms usually affect both lower extremities and can progress to the point where they are present even at rest. If the stenosis is severe and ongoing, neurologic changes including weakness, abnormal reflexes, spasticity, alterations in sensation and proprioception below the level of the stenosis, and bladder dysfunction may be present (Hurko et al. 1988).
Radiographic evaluation is warranted in any patient experiencing symptoms of spinal stenosis. Plain x-rays are often the first line of evaluation. These x-rays can determine if abnormalities of spinal alignment, degenerative changes such as the presence of osteophytes, degenerative disk disease, and abnormalities of the shape and configuration of the vertebral body complex are present. CT will provide a detailed evaluation of the bony structure of the spine. Myelogram is very useful in evaluating the spinal canal; however, performing the lumbar puncture necessary for the myelogram may prove difficult because of the anatomical changes of the spine in achondroplasia. Magnetic resonance imaging (MRI) provides very detailed information about the soft tissue structures including the spinal cord, ligaments, and intervertebral disks (Morgan and Young 1980).
11.6.4 Conclusion
Achondroplasia is a complex genetic disorder involving bone and cartilage formation. The manifestations of this disorder result in abnormalities of the craniocervical juncture and the spine. These abnormalities can become problematic at any time throughout the achondroplast’s lifespan; however, cervicomedullary insufficiency and abnormalities of spinal alignment, including thoracolumbar kyphosis and lumbosacral hyperlordosis, are most common in early childhood. Stenosis below the cervicomedullary juncture often does not become symptomatic until later into adulthood, but can begin to manifest in the teen years. Early recognition and treatment of these disorders is important to promote normal growth and development of the achondroplastic child.
11.7 Klippel-Feil Syndrome
Klippel-Feil syndrome (KFS) was first described in 1912 by Maurice Klippel and Andre Feil (Sullivan and O’Dononghue 2005). This syndrome is a rare congenital disorder that affects the spine as well as many other body systems. This disorder is characterized by “the congenital fusion of any two of the seven cervical vertebrae” (Sullivan and O’Dononghue 2005). The resulting fusion is caused by a failure of the normal division of the cervical somites vertebrae during early fetal development. Patients with Klippel-Feil syndrome present with a triad of symptoms: short neck, low hairline at the back of the head, and restricted mobility of the upper spine (Fig. 11.2).
11.7.1 Diagnosis
Diagnosis is based on clinical presentation and radiographic exam. The clinical presentation varies because of the number of associated syndromes and anomalies that can occur. Associated abnormalities may include scoliosis, spina bifida, anomalies of the kidneys and the ribs, cleft palate, respiratory problems, and heart malformations. The disorder also may be associated with abnormalities of the head and face, skeleton, sex organs, muscles, brain and spinal cord, arms, legs, and fingers (Curcione and Mackenzie 1995). Careful evaluation is essential as some associated anomalies may be fatal if not recognized and treated.
11.7.2 Radiographic Evaluation
Anteroposterior (AP) and lateral views of the cervical spine are done to determine the presence of anomalies. Flexion-extension radiographs should be done if instability or anomalies are suspected or if two fused segments are separated by an open segment (Fig. 11.11). AP and lateral chest radiographs may be done to rule out rib fusions and cardiac involvement. CT scans of the entire spine are useful to determine associated abnormalities. MRI scans are indicated in patients with neurological deficits. These scans may reveal cord compression, spinal stenosis, and central nervous system anomalies such as syringomyelia. Renal ultrasounds are used to determine renal involvement, and an intravenous pyelography is done to delineate any abnormality found on ultrasound.


Fig. 11.11
Fused segment of Klippel-Feil syndrome (arrow)
11.7.3 Treatment
Treatment for KFS is focused on relieving associated symptoms. Medical treatment may involve a wide variety of specialists depending on which anomalies are present. A patient with KFS may be seen by a cardiologist, audiologist, and urologist among others. Physical therapy may be useful. Because the spinal anomalies are often progressive in nature, surgical intervention is often necessary to relieve cervical or craniocervical instability and constriction of the spinal cord and to correct scoliosis. Depending on the type of surgery needed, neurosurgery, orthopedic surgery, or both may be necessary to correct the anomalies.
KFS may be found at any stage of life. This syndrome may be progressive and may involve other specialty care. As with the previously discussed syndromes and skeletal dysplasias, it is important to educate the parents of possible related anomalies and symptoms associated with those anomalies. Early diagnosis, consistent follow-up, and multidisciplinary care are important for positive outcomes.
11.8 Mucopolysaccharide Disorders
Mucopolysaccharide disorders (MPS) were first identified in 1917 (website MPS Society). These inherited disorders are errors of metabolism that are progressive in nature and may not become apparent until later in childhood. The disorder may also be referred to as lysosomal storage disorder (LSD). The lysosomal enzyme normally found in each cell is needed to degrade and recycle glycosaminoglycans. If these enzymes are not degraded and recycled, they accumulate within the cells causing the disease process with progressive damage to the body (Vogel et al. 2004). People affected with these disorders either do not produce enough of any one of the 12 identified enzymes that normally degrade and recycle the sugar chains into proteins, or the enzymes do not work correctly to produce the enzyme necessary for degradation.
11.8.1 Inheritance
The nature of the disease has an autosomal-recessive inheritance and an abnormal gene is inherited from each parent. The odds of receiving the disorder if both parents are affected are one out of every four pregnancies. The unaffected child of parents with the gene has two in three risks of being a carrier and a one in three chances of being a noncarrier of the disorder. The sole exception is MPS type II (Hunter syndrome) as this is X-linked and recessive inheritance. The Hunter gene is carried by a normal female and there is a 50/50 chance of transmission to each of the male offspring. Estimates have the occurrence in population at 1 birth in 31,000 (NCBI website, MPS Society website) (Table 11.1).
Table 11.1
Syndromes associated with MPS
Type/syndrome | Disease name | Deficiency |
---|---|---|
MPS I | Hurler/Hurler-Scheie syndrome | α-l-Iduronidase |
MPS II | Hunter syndrome | Iduronate sulfatase |
MPS III A | Sanfilippo syndrome | Heparan-N-sulfatase |
MPS III B | Sanfilippo syndrome | α-N-Acetylglucosaminidase |
MPS III C | Sanfilippo syndrome | Acetyl CoA: α-glycosaminide |
MPS III D | Sanfilippo syndrome | N-Acetylglucosamine-6-sulfatase |
MPS IV A | Morquio syndrome | Glactose-6-sulfatase |
MPS IV B | Morquio syndrome | Galactosidase |
MPS VI | Maroteaux-Lamy syndrome | N-Acetylgalactosamine-4-sulfatase |
MPS VII | Sly syndrome | Glucuronidase |
MPS IX | Hyaluronidase | |
ML II | I-Cell | N-acetylglucosamine-1-phosphotransferase |
ML III | Pseudo-Hurler polydystrophy | N-acetylglucosamine-1-phosphotransferase |
11.8.1.1 Characteristics
The children with MPS may appear normal at birth and develop normally for the first few years of life. The slow progression of this disorder is related to the gradual buildup of metabolites. Usually, the symptoms that prompt further clinical investigation are the repeated upper respiratory infections, colds, runny noses, and ear infections (MPS Society website). Clinical features may produce neurological complications through impaired signals as damage occurs to neurons affecting motor function and pain receptors. Children with MPS often have mental retardation, hyperactivity, depression, and pain, and their growth and development may be stunted (Fig. 11.12). All children with MPS have coarse facial features and skeletal involvement, such as skeletal dysplasia (Cleary and Wraith 1995; Menezes et al. 2001; Neufeld and Muenzer 2001; Scheie et al. 1962). Some may have an absence of the odontoid process or odontoideum. They may have a thoracic gibbus deformity, a form of structural kyphosis. The curvature is not smooth, as the posterior curve is angled sharply, and results in a humpback that is more prominent on forward bend.


Fig. 11.12
13-year-old female with mucoplysaccharide disorder, Morquio syndrome
Morquio syndrome, MPS type IV, is another disorder that involves skeletal and spinal problems. Death commonly occurs by age 7 years from hypoxia secondary to a cervical myelopathy and subsequent effects upon the respiratory system (Cleary and Wraith 1995; Menezes 1999). Atlanto-axial instability, scoliosis, and kyphosis of the thoracolumbar spine are problems. There is also a characteristic flattening of the vertebrae with a flame-shaped pattern of ossification (Fig. 11.13). Other characteristics of MPS may include general ligamentous laxity, thought to contribute to atlanto-axial subluxation (Menezes et al. 2001); corneal clouding; speech or hearing impairment; chronic runny nose; hernias; heart disease; development of hydrocephalus; stiff joints; splenomegaly; liver enlargement; diarrhea; and shortened life expectancy. Symptoms appear as the storage of the enzymes increases. The projected life expectancy of children with MPS is 10–20 years (Cleary and Wraith 1995; Neufeld and Muenzer 2001; Scheie et al. 1962; MPS Society website).


Fig. 11.13
Flame-shaped vertebrae (arrow) with spinal cord narrowing on MRI characteristic of MPS in Morquio syndrome
11.8.2 Diagnosis
The diagnosis of MPS may be made through clinical examination, and urine and tissue testing. Genetic counseling and reviewing family history for at least three generations may assist couples in determining if they are carrying the mutated gene responsible for the development of the disorders (Clarke 2005). Prenatal diagnosis utilizing amniocentesis and chorionic villus sampling at 14–17 weeks gestation should be done to determine if the fetus has the defective gene or is affected by the disorder. Clinical signs and symptoms do not demonstrate a diagnosis. Further definitive testing such as enzyme-specific assays for alpha-L-iduronidase, peripheral blood leukocytes, and plasma or cultured fibroblasts should be ordered (Hall et al. 1978; Neufeld and Muenzer 1995, 2001). For an infant suspected of having an inborn error of metabolism, laboratory studies should include complete blood counts, urinalysis, capillary blood gases, electrolytes, glucose, ammonia, urine-reducing substances, urine ketones, plasma and urine amino acids (quantitative), urine organic acids, and plasma lactate (Burton 1998).
11.8.3 Treatment
Currently there is not a cure for MPS; therefore, treatment is focused on relieving and treating symptoms as they arise. The FDA has approved several medications for use in MPS. Some of the enzyme replacement therapies are specific for a particular disease: Aldurazyme (laronidase) in 2003 and Naglazyme in 2005. These enzyme replacement therapies for MPS types I and IV are proving to be useful in the reduction of pain and non-neurological symptoms of MPS. They replace the deficient enzymes and are given IV once a week for life. Bone marrow transplant has been utilized to replace lost enzymes; however, some of the children have heart disease due to the syndrome and cannot withstand the chemotherapy required for the transplantation of bone marrow. Bone marrow transplantation, along with umbilical cord blood transplants, is showing limited success with improved survival.
11.9 Traumatic Spinal Cord
11.9.1 Pathophysiology
11.9.1.1 The Pediatric Spinal Column
The immature pediatric spinal column has unique features that increase the spinal cord’s susceptibility to injury without obvious evidence of abnormality in alignment or bony integrity. Among those are a higher fulcrum of cervical movement, a larger head to body ratio, wedge-shaped vertebral bodies, underdeveloped uncinate processes, increased elastic interspinous ligaments, and underdeveloped neck and paraspinous muscles. Those anatomic features increase the mobility of the pediatric spinal column, causing it to be hypermobile and more susceptible to flexion and extension type injuries.
Pediatric spinal trauma patients can be divided into two unique groups. The first group is from birth to 8 years of age. The spinal anatomy of children becomes more similar to adults by age 8 but may not reach full maturity until age 16–18 years (Bosch et al. 2002; Ergun and Oder 2003). As mentioned, there are specific anatomic differences in the younger age group. First, the fulcrum of cervical movement is higher (C2–C3), as opposed to the adult level (C5–C6) (Grabb 2008). Accordingly, there is a high percentage of cervical spine injuries between occiput and C3 in children under 8 years of age. In a study of 227 children with spinal cord injury (SCI), 87% of those less than 8 years old had an injury C3 or higher (Khanna and El-Khoury 2006).
The disproportionate size of the head also contributes to the risk of spinal cord injury in children under age 9 (Grabb 2008). The development of head to body ratio is illustrated in Fig. 1.1 in the Assessment chapter. Additionally, the large head is not well supported because the neck muscles are underdeveloped in the younger child. Those factors put the young child at greater risk for flexion-extension injuries to the C-spine.
The spine is also hypermobile related to both the laxity of the intraspinous ligaments and the underdevelopment of the paraspinous musculature (Carreon et al. 2004; Lang and Bernardo 1993; Pang et al. 1982). In comparison, the spinal cord is much less elastic. This accounts for higher incidence of subluxation without fractures and spinal cord injury without radiographic abnormality (SCIWORA) in children.
Pediatric bony development of long bones includes growth plates called epiphyses and ossification centers (which are the areas where cartilage is turning to bone). Similar to long bones, the spinal column also has 11 growth centers known as synchondroses and ossification centers. In the upper cervical spine, C1 (Atlas) forms from three primary ossification centers: the anterior arch and two neural arches (Fig. 11.14). These don’t fuse until between 3 and 6 years of age. Similar to ossification centers in long bones being a point of weakness because they are not hard bone yet, ossification centers in the spine are also a weak point for trauma. C2 (axis) develops from five primary ossification centers. The body of the peg-like odontoid (also known as dens) and two neural arches typically fuse to the bone between 3 and 6 years of age as well. This makes odontoid injuries in younger children much more prevalent due to the weakness through this ossification center (Khanna and El-Khoury 2006).


Fig. 11.14
Axial reconstructed CT images of C1 (atlas) (a) 1 month old: ossification centers of both the neural arches are present, but the ossification center for the anterior arch is not yet seen (because it is still cartilage and not turned to bone). (b) 1-year-old: ossification centers of the anterior arch and both neural arches are seen but are still open and not yet fused. (c) 3-year-old: the neural arches in the back are almost fused, while the growth centers (synchondroses) of the anterior arch are still open. (d) 6-year-old: anterior arch and neural arches are completely fused (Khanna and El-Khoury 2006)
Other anatomic features that increase the mobility of the pediatric spine involve the shape of certain parts of the spinal column. The vertebral bodies are wedge-shaped in children, allowing for slippage anteriorly during flexion. With age, the vertebral bodies mature. They evolve through four basic types: type I, oval immature; type II, rounded upper corner; type III, anterior wedging; and type IV, rectangular, more mature (Eubanks et al. 2006) (Fig. 11.15). Also, the facet joints are much more horizontally oriented in the pediatric spine in comparison to the adult spine. This again allows for translation of the vertebral bodies as the spine is flexed forward or extended back. Further, the uncinate processes, which serve to limit spinal mobility at C3–C7 and T1, particularly as to rotational and lateral movement, are underdeveloped in children under age 10 (Brockmeyer 2006). By contrast, in the adult spine, they are hooklike, projecting upward and articulating with the vertebral level above.


Fig. 11.15
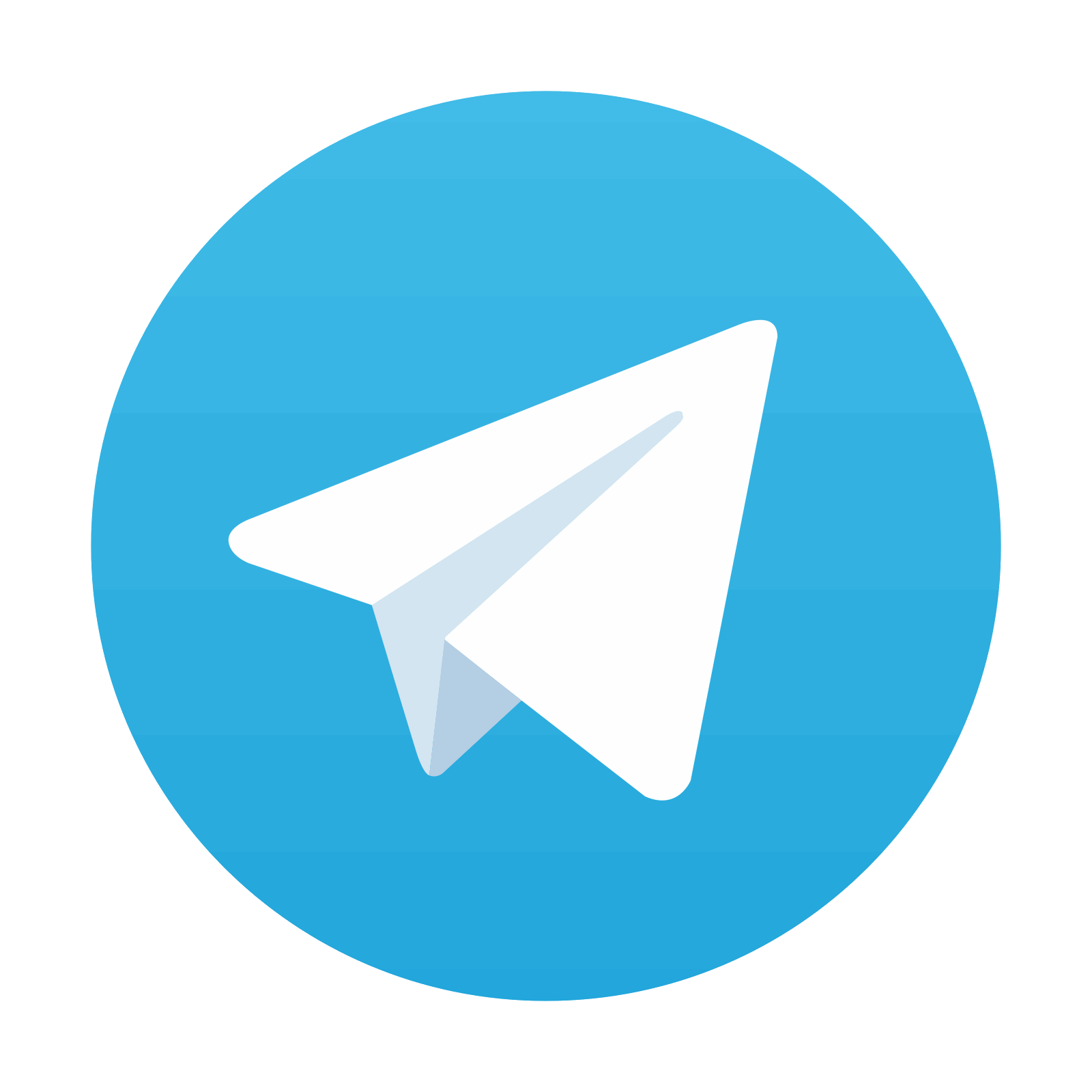
Vertebral shapes (Eubanks et al. 2006)
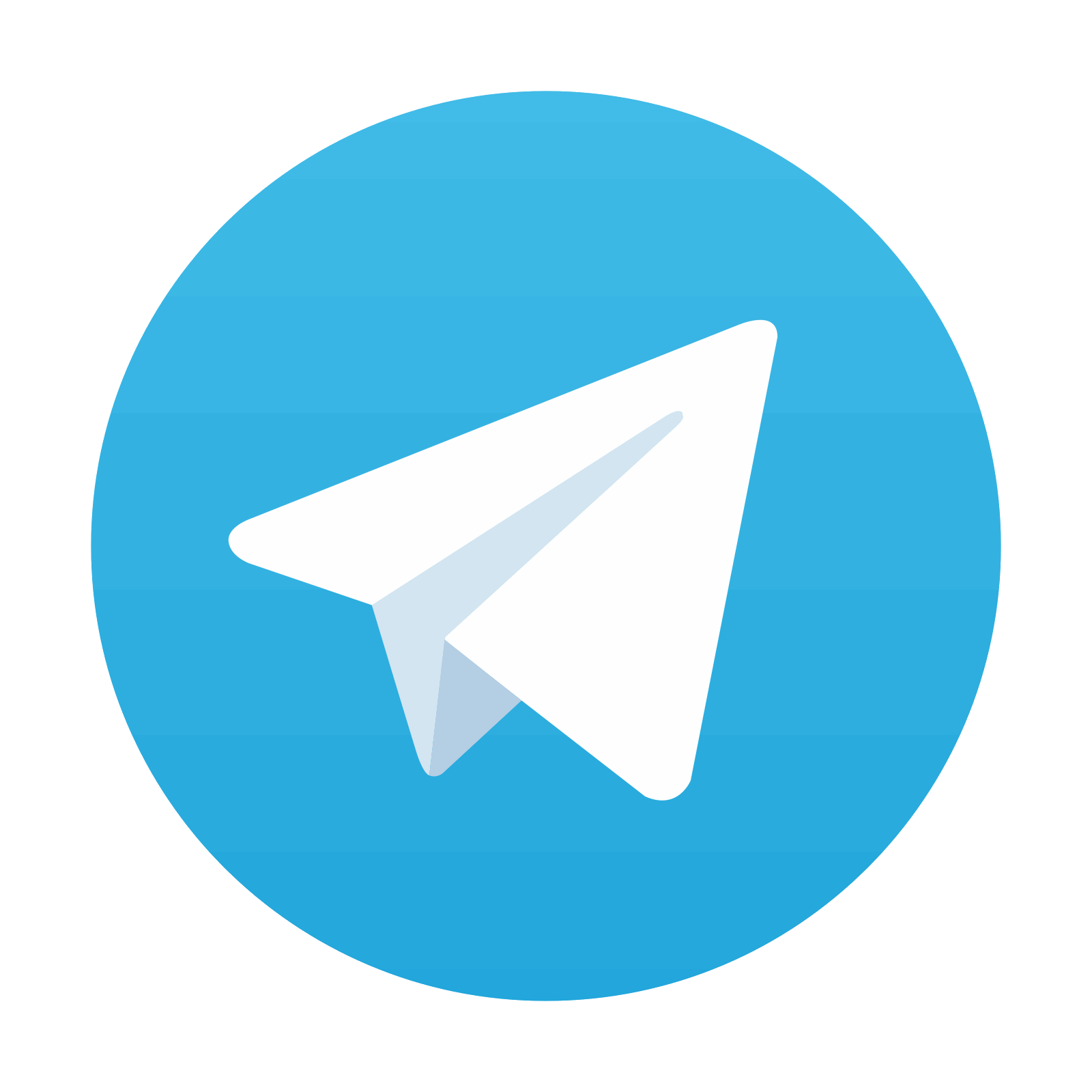
Stay updated, free articles. Join our Telegram channel

Full access? Get Clinical Tree
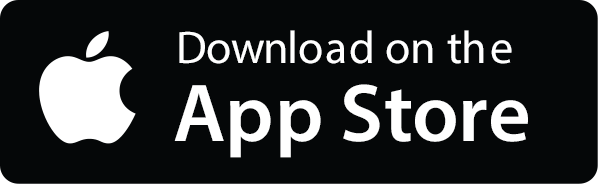
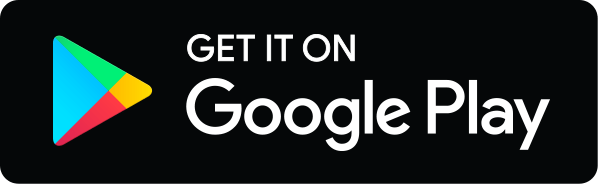
Get Clinical Tree app for offline access
