Chapter 35 Screening and assessment
Learning outcomes for this chapter are:
1. To explain the concept of screening during pregnancy
2. To describe the tests, examinations and other procedures available to women
3. To discuss the known benefits and disadvantages of screening procedures
4. To discuss the ethical, legal and social dilemmas accompanying the use of innovative medical technologies in antenatal screening.
This chapter builds on the information in Chapter 22. It follows on from the assessments that will have been made during the antenatal visits and discusses in depth the screening and assessment techniques currently undertaken in New Zealand and Australia. The chapter is not intended as a ‘cookbook’ guide on when and how to advise women about screening. You will be aware of the different screening policies at the national and area healthcare service levels. This chapter is intended to introduce the student midwife to current debates and information appropriate to practice.
INTRODUCTION: SCREENING GUIDELINES
Three Centres Consensus Guidelines
The Three Centres Consensus Guidelines on Antenatal Care are available online at www.health.vic.gov.au/maternitycare/anteguide.pdf.
• Visits 1 and 2 are in the first trimester. From the caregiver’s perspective, first-trimester visits are primarily to assess maternal and fetal wellbeing, particularly the risk of complication, to date the pregnancy, take a comprehensive history, discuss smoking behaviour and establish care options. The visits are scheduled in order to offer screening tests recommended for asymptomatic bacteriuria, syphilis, hepatitis B and C viruses (HBV and HCV), HIV and Down syndrome.
• Visits 3 and 4 are in the second trimester. Second-trimester visits are primarily scheduled to monitor fetal growth, maternal wellbeing and signs of preeclampsia. If ultrasound is routinely offered, it should be included as part of a visit at 18–20 weeks. If women have glucose screening, this should be part of a visit at 24–28 weeks.
• Visits 5 to 8 are in the third trimester. Third-trimester visits are primarily to monitor fetal growth, maternal wellbeing, signs of preeclampsia, and to assess and prepare women for admission, labour and going home. These visits may include bacteriological screening for Group B Streptococcus (GBS) (at 35–37 weeks), and preparations for admission, labour and ‘going home’, consistent with other guidelines.
NICE Guidelines
The NICE (National Institute for Health and Clinical Excellence) Guidelines are available on their website —see ‘Antenatal care: routine care for the healthy pregnant woman’ at www.nice.org.uk/CG006.
Midwives Handbook for Practice
The New Zealand College of Midwives’ Midwives Handbook for Practice (NZCOM 2008) says that the first decision point in pregnancy (within the first 16 weeks of pregnancy) is timed to allow for a comprehensive assessment to be made according to the best available evidence. It is an ideal time to discuss the role that both the midwife and the woman will undertake in the following pregnancy and birth. It will also ensure that there is time to make appropriate decisions regarding treatment and decisions to intervene in the pregnancy if this is what eventuates following the screening procedures that may be undertaken. It is important that the midwife adequately explains to a pregnant woman what screening tests are meant to detect, how they are conducted, possible risks to her and her fetus, the type of results that will be reported (e.g. probability, risk), the likelihood of false-positive or false-negative results, and the choices she will face once results are obtained (NICE 2008).
INFORMATION SHARED
Chapter 22 covered in detail how to do a booking and the regular observations that midwives attend to in the antenatal period. This chapter discusses the contemporary screening options offered to women, and discusses the appropriateness of screening for each condition.
Review of current and past maternity history
At this time you will ascertain the number of pregnancies a woman reports (gravida) and the number of births (parity). Note: The definitions of mortality differ between Australia and New Zealand. In both Australia and New Zealand, a live birth is recorded if the fetus is 20 weeks gestation or over, and weighs 400 g or more at the time of birth (AIHW 2004). The perinatal death rate in Australia includes stillbirth (or fetal death) and death up to 28 days after birth; in New Zealand, a perinatal death is a stillbirth (fetal death) or a death up to 7 days after birth. The denominator for perinatal death rate is per 1000 births: both live and stillborn over these time periods.
Definition of terms
• Parity refers to the total number of pregnancies continued beyond 20 weeks gestation, regardless of the outcome (i.e. live birth or stillbirth) or the number of fetuses in the uterus (i.e. parity is not influenced by multiple births—plural pregnancies are counted as one).
• A nulliparous woman has never given birth to a viable infant, or, in other words has never completed a pregnancy beyond 20 weeks gestation.
• The term primiparous refers to a pregnant woman who has given birth for the first time and has had no previous pregnancy before this birth resulting in a live birth or stillbirth.
• Multipara refers to a pregnant woman who has had at least one previous pregnancy resulting in a live birth or stillbirth before this one; in other words, a woman having completed at least one pregnancy beyond 20 weeks.
• Grand multipara refers to a pregnant woman who has had four or more previous pregnancies resulting in a live birth or stillbirth (i.e. this is her fifth (or more) pregnancy).
SCREENING TESTS
A good screening test should be able to discriminate clearly between those who are at high risk and those who are not. It should be safe, have a reasonably defined cut-off level, and be both valid and reliable. Although these latter two terms are often used interchangeably, they are distinct. Validity is the ability of a test to measure what it sets out to measure, usually differentiating between those with and without the disease. By contrast, reliability indicates repeatability (Grimes & Schulz 2002, p 882). It refers to a method of measurement that consistently gives the same results (NICE 2008).
Screening differs from the traditional clinical use of tests in several important ways. When we believe there is something wrong with us, it prompts us to consult an expert (perhaps a doctor) about the complaint or problem, and this in turn prompts testing to confirm or exclude a diagnosis. By contrast, screening engages apparently healthy women who are not seeking medical help (and might prefer to be left alone). In addition, the occurrence of false-positive results and true-positive results may lead to (dangerous) interventions. Although the anxiety induced by a correct diagnosis may be overwhelming, those incorrectly thought to have a problem suffer as well. For example, although failing to diagnose sexually transmitted diseases can have important health implications, incorrectly labelling people as infected can wreck marriages and damage lives (Grimes & Schulz 2002).
Assessing the effectiveness of a test
There are four indices of the validity of a test: sensitivity, specificity, and predictive values of positive and negative (see Fig 35.1).
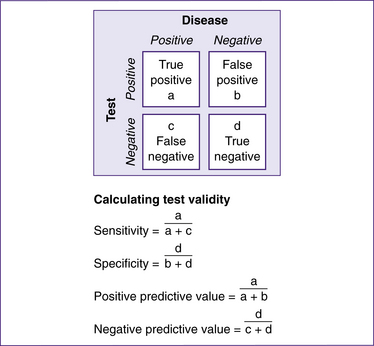
Figure 35.1 Assessing the effectiveness of a screening or diagnostic test
(based on Grimes & Schulz 2002)
Sensitivity
Sensitivity is sometimes termed the ‘detection rate’. It describes the ability of a test to find those with the disease (Grimes & Schulz 2002). In diagnostic testing, sensitivity refers to the chance of having a positive test result, given that you have the disease. A 100% sensitivity means that all those with the disease will test positive, but this is not the same the other way around. A woman could have a positive test result but not have the disease—this is called a ‘false-positive’. The sensitivity of a test is also related to its ‘negative predictive value’ (true-negatives): a test with a sensitivity of 100% means that all those who get a negative test result do not have the disease. To fully judge the accuracy of a test, its specificity must also be considered (NICE 2008, glossary).
Specificity
Specificity denotes the ability of a test to identify those without the condition (Grimes & Schulz 2002). In diagnostic testing, this refers to the chance of having a negative test result, given that you do not have the disease. A 100% specificity means that all those without the disease will test negative, but this is not the same the other way around. A woman could have a negative test result, yet still have the disease—this is called a ‘false-negative’. The specificity of a test is also related to its ‘positive predictive value’ (true-positives): a test with a specificity of 100% means that all those who get a positive test result definitely have the disease. To fully judge the accuracy of a test, its sensitivity must also be considered (NICE 2008, glossary).
For the purposes of this chapter, it is important to understand the concepts of sensitivity and specificity broadly, so that you understand how screening works in real life. For example, the trade-off between sensitivity and specificity (see Fig 35.2) is demonstrated by the following. For any continuous outcome measurement (e.g. blood glucose level), the sensitivity and specificity of a test will be inversely related. If we place the cut-off for abnormal blood glucose level at a very low point, thereby producing perfect sensitivity (i.e. we manage to identify all those women with diabetes), the low cut-off identifies all those with diabetes. However, the trade-off is poor specificity—that is, those women who also have blood glucose at the level specified but are part of the ‘healthy distribution’ (i.e. at one end of ‘normal’) are incorrectly identified as having abnormal values. Placing the cut-off higher yields the opposite result: all those who are healthy are correctly identified (perfect specificity), but the cost here is missing a proportion of women who do have diabetes. Selecting a cut-off point is a compromise, mislabelling some healthy people and some people with diabetes. Where the cut-off should be depends on the implications of the test.
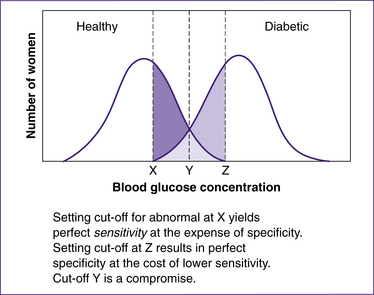
Figure 35.2 Hypothetical trade-off between sensitivity and specificity
(based on Grimes & Schulz 2002)
the view of the Institute, which was arrived at after careful consideration of the evidence available. Health professionals are expected to take it fully into account when exercising their clinical judgment. The guidance does not, however, override the individual responsibility of health professionals to make decisions appropriate to the circumstances of the individual patient, in consultation with the patient and/or guardian or carer (NICE 2008).
APPOINTMENT TIMES FOR SCREENING
First appointment
• give information to enable informed decision-making about screening tests
• identify women who may need additional care
• check blood group and Rhesus D (RhD) status
• offer screening for anaemia, red-cell alloantibodies, hepatitis B virus, HIV, rubella susceptibility and syphilis
• offer screening for asymptomatic bacteriuria (ASB)
• offer screening for Down syndrome
• offer early ultrasound scan for gestational age assessment
• offer ultrasound screening for structural anomalies (20 weeks).
After the first (and possibly second) appointment, for women who choose to have screening, the following tests should be arranged before 16 weeks of gestation (except serum screening for Down syndrome, which may occur up to 20 weeks of gestation).
GESTATIONAL AGE ASSESSMENT
The information in this section is from the NICE Guidelines (2008, p 77).
Ultrasound scans
Early in pregnancy (usually around 10–13 weeks), the first ultrasound scan may be offered. It is used to estimate when the baby is due and to check whether there is more than one fetus. If you don’t see women this early, it is appropriate to offer the scan at the earliest time. Between 18 and 20 weeks appears to be the optimum time to offer a second scan to check for physical abnormalities in the fetus. Beyond these it is not recommended that women have any further routinely offered scans, as they have not been shown to be useful (NICE 2008).
Individual clinicians, maternity units and geographic regions have adopted widely varying strategies for combining LMP and early ultrasound (EUS) estimates of gestational age (GA). Some still use LMP, others rely exclusively on EUS, while many base GA on EUS only when the discrepancy with LMP exceeds a given limit, such as ±7, 10 or 14 days (Blondel et al 2002).
Table 35.1 shows the effect of estimating the GA in a fetus using the various methods of estimation in a study in 44,623 pregnancies in France. Note the discrepancies between post-term dates using the LMP method and the EUS method. Some authors recommend ultrasound estimation as the first choice for dating all pregnancies; others have voiced reservations about exclusive use of EUS estimates. Because of these different approaches to resolving discrepancies in LMP and EUS estimates, both among and within countries, geographical and temporal trends in preterm and post-term birth remain difficult to interpret (Blondel et al 2002).
Table 35.1 Rates of preterm and post-term birth, estimated from LMP or EUS, according to six algorithms for combining the two estimates (n = 44,623)
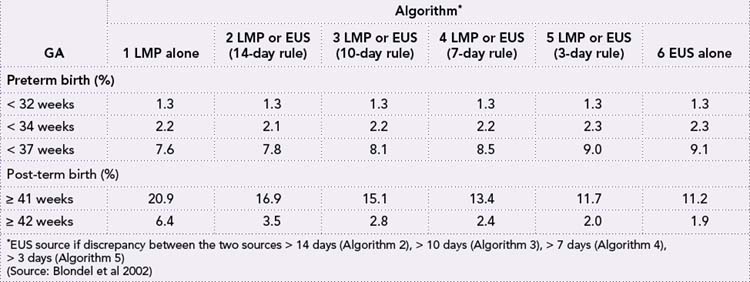
Estimates of gestational duration based on the timing of the last normal menstrual period (LNMP) are dependent on a woman’s ability to recall the dates accurately, the regularity or irregularity of her menstrual cycles and variations in the interval between bleeding and anovulation. Crowther and colleagues (1999) estimate that between 11% and 42% of GA estimates from LMP are reported as inaccurate. Ultrasound measurements are based on standard growth curves, but fetal growth may deviate from the standard, even early in gestation. Fetuses that grow faster than the standard curve will be given a biased, longer gestational length, and therefore an increased probability of being classified as post-term, even when they are not (Olesen et al 2004). The opposite will be seen for slow-growing fetuses. Lower early ultrasound-based GA estimates therefore seem attributable in some cases to slower fetal growth (Olesen et al 2004). This observation was also reported by Smith et al (1998).
In a study that examined the determinants and consequences of fetal growth restriction as a function of differences in GA estimates, Morin et al (2005) found that several maternal and fetal characteristics influenced the magnitude of the discrepancy between the GA estimates. These included socioeconomic differences and potential determinants of early fetal growth restriction (Morin et al 2005). Small differences in growth may also depend on fetal sex, maternal age, parity and smoking (Olesen et al 2004). The NICE guideline group conclude, however, that there is thought to be little variation in fetal growth rate up to mid-pregnancy and, therefore, estimates of fetal size by ultrasound scan provide estimates of GA that are not subject to the same human error as LNMP (NICE 2008).
Ultrasound assessment of GA at 10–13 weeks is usually calculated by measurement of the crown–rump length. For pregnant women who present in the second trimester, GA can be assessed with ultrasound measurement of biparietal diameter or head circumference (NICE 2008). Ultrasound measurement of biparietal diameter is reported to provide a better estimate of date of delivery for term births than first day of the LMP (NICE 2008, p 77). Routine ultrasound before 24 weeks is also associated with a reduction in rates of intervention for post-term pregnancies. A 1998 Cochrane Review (Neilson 1998) found that, compared with selective ultrasonography, routine prenatal ultrasonography before 24 weeks gestation provided better GA assessment and earlier detection of multiple pregnancies and fetal malformations. This review reported, in a long-term follow-up of Norwegian and Swedish children, no adverse influence on school performance or neurobehavioural function as a consequence of antenatal exposure to ultrasound; however, fewer of the ultrasound-exposed children are right-handed (Neilson 1998). The ‘Alesund’ trial, which was reported in 2000 (Eik-Nes et al 2000), claimed the possible benefits of the routine use of ultrasound screening in pregnancy as a lower incidence of induced labour due to apparent post-term pregnancies—approximately 70% lower in the ultrasound-screened group. Inductions from all causes were also less frequent among ultrasound-screened women. Among the controls, three pairs of twins remained undiagnosed until the mothers were admitted to the hospital in labour at between 36 and 38 weeks gestation. The authors concluded that for women who were screened with ultrasound, obstetricians were less likely to induce labour due to apparent post-term pregnancy than for women who were not screened (Eik-Nes et al 2000).
Accurately assessing the GA also permits optimal timing of antenatal screening for Down syndrome and fetal structural anomalies. Reliable dating is important when interpreting Down syndrome serum results, as it may reduce the number of false-positives for a given detection rate (NICE 2008).
Side-effects of ultrasound
The use of ultrasonography for evaluating the developing embryo/fetus continues to rise, although the potential risks from exposure are uncertain (Barnett et al 2000; Marinac-Dabic et al 2002; Miller et al 1998). An effect of ultrasound on intellectual performance cannot, at present, be ruled out. Researchers from Sweden who published their work in the journal Epidemiology in 2005 concluded that further action to evaluate possible neurotoxic effects of ultrasound should be taken, particularly because ultrasound exposure in the published studies looking at long-term effects of exposure was low compared with present levels of exposure (Kieler et al 2005).
Prenatal exposure to ionising irradiation in the second trimester has been found to affect the developing brain, resulting in intellectual impairment (Schull & Otake 1999). Whether other forms of radiation, particularly non-ionising radiation such as ultrasound, have similar neurotoxic effects is not yet known (Ziskin & Barnett 2000). Ultrasound has the potential to damage tissue by heating, cavitation or streaming, and the brain is most susceptible to environmental effects during its development (Barnett 1998; Miller et al 1998). Modern ultrasound machines report Mechanical Index (MI) and Thermal Index (TI) as real-time feedback to the operator of the potential for fetal effects. Tissue temperature elevations become progressively greater from b-mode to colour Doppler to spectral Doppler applications, and the precautionary principle of as little exposure as possible while obtaining needed clinical information is recommended. Although epidemiological research on possible adverse effects of ultrasound is sparse, there have been published reports of an association between
non-right-handedness/left-handedness in males and exposure to prenatal ultrasound. The significance of ‘handedness’ studies is the higher risk of an association of left-handedness in infants with lowered intellectual abilities (Kieler et al 2005). However, in a study examining the association between prenatal ultrasound exposure and intellectual performance, Kieler et al (2005) failed to demonstrate a clear association between ultrasound scanning and intellectual performance.
SCREENING FOR DOWN SYNDROME AND FETAL STRUCTURAL ANOMALIES
Screening in Australia and New Zealand
To date, there has been an ‘ad hoc’ approach to serum screening in Australia and New Zealand. In Australia and New Zealand, prenatal screening tests are available to identify pregnancies at increased risk of chromosome anomalies such as trisomy 21, trisomy 18 and some structural anomalies such as neural tube defects. Ultrasound and maternal serum screening tests identify fetuses with an increased likelihood of having one of these conditions. Sometimes these conditions are not compatible with live birth, some are associated with
Box 35.3 Ultrasound past and present
From Sarah Buckley’s book Gentle Birth (2005)
This new technology spread rapidly into clinical obstetrics. Commercial machines became available in 1963 (De Crepigny 1996) and by the late 1970s ultrasound had become a routine part of obstetric care (Oakley 1986). Today, ultrasound is seen as safe and effective, and scanning has become a rite of passage for pregnant women in developed countries. In Australia, it is estimated that 99% of babies are scanned at least once in pregnancy—usually as a routine prenatal ultrasound (RPU) at 4 to 5 months. In the US, where this cost is borne by the insurer or privately, around 70% of pregnant women have a scan (Martin et al 2002), and in European countries, it is estimated that 89% of pregnant women have an ultrasound, usually once in each trimester (third) of pregnancy (Levi 1998). However, there is growing concern as to its safety and usefulness. UK consumer activist Beverly Beech has called RPU ‘the biggest uncontrolled experiment in history’ (Beech 1993) and the Cochrane Collaborative Database—the peak scientific authority in evidence-based medicine—concludes that ‘no clear benefit in terms of a substantive outcome measure like perinatal mortality [number of babies dying around the time of birth] can yet be discerned to result from the routine use of ultrasound’. For those considering its introduction, the benefit of the demonstrated advantages would need to be considered against the theoretical possibility that the use of ultrasound during pregnancy could be hazardous, and the need for additional resources (Neilson 1998).
(Source: Buckley 2005; references within this box are those cited by Buckley)
long-term and serious morbidity, and some require neonatal investigation or treatment. There is usually no intrauterine fetal therapy (RANZCOG 2009).
Policies that detail the aims and operations of antenatal screening programs are available online for New South Wales, South Australia, Victoria and Western Australia. The Royal Australian and New Zealand College of Obstetricians and Gynaecologists (RANZCOG) also produces national policy statements on prenatal screening (RANZCOG 2009).
There does not appear to be a consistent view on whether screening should be offered to all women or targeted to women of a particular age group. Best estimates of the rates of participation in either first- or second-trimester screening tests varies between 39% (Queensland) and 80% (South Australia), with much lower uptake (17%) in the Northern Territory. Most states had between 50% and 70% participation rates, with the majority of screening tests performed in the first trimester. The states with higher rates of participation were also those with policies that provided information and guidance to both healthcare professionals and the community (O’Leary et al 2006). This is despite the introduction in Australia of a specific Medicare item number (MBS 66321) and rebate for the maternal serum screen. Only the New South Wales, South Australian and Western Australian governments have policies for antenatal screening for Down syndrome and other fetal anomalies (O’Leary et al 2006).
Recommendations for antenatal screening for Down syndrome in Australia are as follows:
Pre-screening counselling must be given by appropriately trained staff and should be specific to the age of each woman.
If after counselling women choose to proceed with screening tests for Down syndrome:
i. Screening should include accurate pregnancy dating by ultrasound, preferably in the first trimester.
ii. Screening should be by either second trimester biochemistry or by nuchal translucency (alone or in combination with first or second trimester biochemistry).
iii. Women should be notified of their screening result, irrespective of the risk, in a format that they understand.
iv. Women who have an increased risk of Down syndrome should be offered further counselling and diagnostic testing within 72 hours or as soon as possible (Three Centres Consensus Guidelines 2001).
In New Zealand, antenatal screening for Down syndrome has developed rapidly over the past 15 years. There is now a range of screening tests available, including:
• a first-trimester blood test (not yet widely available in New Zealand), and/or
• a second-trimester blood test (the Triple Test), a combination of three tests that measure quantities of various substances in the blood, usually done between 15 and 20 weeks of gestation (available but women pay $70 for this test), and/or
• an ultrasound scan at about 12 weeks for nuchal translucency (publicly funded). Women identified as ‘high risk’ following screening are offered invasive procedures, for example, chorionic villus sampling (CVS) or amniocentesis. Both these diagnostic tests carry a small risk of fetal loss.
Development of diagnostic testing methods
When the first screening test for Down syndrome was introduced in the United Kingdom, approximately 5% of pregnant women were 35 years of age or older, and 30% of all Down syndrome births were related to those women (Haddow 1998). The vast majority of babies do not have Down syndrome. However all women, whatever their age, have a small risk of delivering a baby with a physical and/or mental disadvantage. The majority of children with Down syndrome are born to women who are younger than 35 years old. The identification of younger women with an increased risk became possible after Merkatz et al (1984) reported an association between a low alpha-fetoprotein level and fetal aneuploidy, launching an era of maternal serum screening to assess the risk of fetal Down syndrome. Subsequent studies showed that the maternal serum levels of three markers—alpha-fetoprotein (AFP), beta human chorionic gonadotrophin (β-hCG), and unconjugated oestriol (uE3)—occurring between 15 and 22 weeks of gestation could be used to make adjustments to the risk based on maternal age alone (Mennuti & Driscoll 2003).
Antenatal screening for Down syndrome became possible because two components of diagnostic testing had developed to the level of clinical applicability. The first was a sampling procedure where cells were taken from the amniotic fluid (amniocentesis). Cells in amniotic fluid are fetal in origin and can be cultured for chromosome analysis. Amniocentesis was originally offered during the early second trimester and carried about a 1% risk of spontaneous fetal loss (Tabor et al 1986). The second diagnostic component that had reached clinical significance was cell culture and chromosomal analysis via karyotype. Karyotyping is still the preferred test method for most pregnancies, ascertained either through a screening program for Down syndrome or for other referral reasons. Karyotyping detects a range of numerical and structural chromosome abnormalities in addition to the common autosomal trisomies—13 (Patau’s syndrome), 18 (Edwards’ syndrome) and 21 (Down syndrome)—and sex-chromosome abnormalities.
However, since amniotic fluid and chorionic villus cells are cultured before analysis, delays of up to 14 days or longer can occur before a result is issued in the case of rare anomalies (Caine et al 2005). The results of CVS for Down syndrome are usually available within in three days of the test in Australia (Fetal Medicine Foundation 2009). Notwithstanding that, this is currently recognised as among the most reliable diagnostic tests in clinical medicine (Haddow 1998). Culture failure is uncommon, and erroneous results from maternal cell contamination are rare (Haddow 1998). This degree of reliability is crucial, since it forms the basis for decisions that have far-reaching consequences. Chromosome analysis via karyotype, however, continues to be the cornerstone of Down syndrome diagnosis (Haddow 1998).
After the landmark discovery in 1984 that AFP concentrations in maternal serum were lower in the presence of Down syndrome and could be used as a screening test (Cuckle et al 1984), screening began to be offered to pregnant women younger than age 35. The efficacy of screening with AFP was comparable to that based on maternal age (a 20%–25% detection rate for a 5% false-positive rate), and since AFP was already being measured as a screening test for open spina bifida or neural tube defect, adding an interpretation for Down syndrome risk represented a negligible cost (Haddow 1998). Further progress in screening was achieved later in the 1980s with the discoveries of β-hCG and uE3. Measures of these markers were combined with that for AFP to produce a ‘triple’ test, capable of detecting 60% of Down syndrome pregnancies, at a 5% false-positive rate (Wald et al 1988). This substantial step-up in detection led many women over the age of 35 to choose serum screening rather than amniocentesis. The addition of a fourth serum marker, inhibin, has been shown to boost detection of Down syndrome to about 75% (Haddow 1998).
With the advent of biochemical markers in combination with ultrasound, considerable attention was given in the 1990s to providing maternal serum screening earlier in gestation. Measurements of two markers—pregnancy-associated plasma protein A (PAPP-A) and free β-hCG detected between 10 and 13 weeks gestation—have become nearly as efficient as the second-trimester ‘triple’ test (Haddow 1998).
Studies in the 1990s showed an association between Down syndrome and increased nuchal translucency, a sonolucent space evident at the back of the fetus’s neck in the first trimester. In 1995, Wald et al demonstrated the feasibility of first-trimester serum screening for Down syndrome (Wald et al 1995).
Current practice
• amount of fluid behind the neck of the fetus (nuchal translucency)
• presence or absence of the fetal nasal bone
• blood flow through the tricuspid valve of the fetal heart
• blood flow through the ductus venosus in the fetal liver
• presence or absence of any physical abnormalities
• level of two hormones (free β-hCG and PAPP-A) in the mother’s blood (Fetal Medicine Foundation 2009).
Screening combinations
The NICE Guidelines recommend the following screening combinations for Down syndrome:
The NICE Guidelines (2008) also state that:
Table 35.2 Expected detection rates and false-positive rates for various second-trimester protocols applied to 1999 US pregnant population
Screening protocol | Detection rate (%) | False- positive rate (%) |
---|---|---|
Maternal age alone (≥ 35 years) | 49 | 13.1 |
Second trimester, maternal age plus: | ||
MSAFP + hCG | 73 | 9.6 |
MSAFP + uE3 + hCG | 78 | 7.8 |
MSAFP + hCG + INH-A | 82 | 6.9 |
First trimester, maternal age plus: | ||
NT | 74 | 5.1 |
PAPP-A + free β-hCG | 75 | 8.6 |
NT + PAPP-A + free β-hCG | 86 | 4.2 |
First and second trimester, maternal age, plus: | ||
PAPP-A + MSAFP + uE3 + hCG + INH-A | 87 | 4.9 |
NT + PAPP-A + MSAFP + uE3 + hCG + INH-A | 93 | 2.6 |
(Source: Benn 2003)
risk of carrying a Down syndrome baby at around 10 to 14 weeks. Nuchal translucency may be used with or without two first-trimester maternal serum markers, human chorionic gonadotrophin (β-hCG) and pregnancy-associated plasma protein (PAPP-A), i.e. the combined test, or as part of the integrated test.
Results are ‘positive’ or classified as ‘high risk’ if the risk is equal to or greater than a locally agreed cut-off level. This is often expressed numerically to indicate the likelihood that a woman has a baby with Down syndrome when a positive screening result is returned; e.g., a 1/250 chance that a pregnant woman is carrying an affected baby. When a high-risk screening result is returned, a woman will usually be offered a diagnostic test, such as amniocentesis, which has an excess fetal loss rate of 1%. (NICE Guidelines 2008)
Some women undergo CVS during the first trimester rather than screening or amniocentesis during the second trimester. Some women, such as those carrying multiple fetuses, are not candidates for second-trimester serum screening. Others decline screening because they do not wish to undergo prenatal diagnosis or would not consider pregnancy termination on the basis of the results. The anxiety experienced by friends or relatives who had a positive screening test followed by normal findings on amniocentesis deters some women from undergoing screening. Women who are 35 years of age or older increasingly opt to undergo serum screening and ultrasonography before deciding about amniocentesis. A range of other ultrasound features such as nasal bone length and echogenic heart areas are currently being investigated as indicators (NICE 2008). With the emergence of alternative approaches earlier in pregnancy, decisions about screening are becoming more complex.
The RANZCOG also provides a comprehensive guide to screening that is updated as new evidence comes to hand (RANZCOG 2007).
Summary: screening for Down syndrome
• The screening tests involved in early or first-trimester pregnancy screening for Down syndrome (DS) when the fetus is between 11 and 13 weeks gestation involve a nuchal translucency (NT) screen, which takes place within the context of a detailed ultrasound scan in addition to maternal serum screening.
• DS screening in the second trimester using a variety of combinations of maternal serum markers such as the so-called ‘triple test’ (hCG, uE3 and AFP) has become an established but controversial part of antenatal care over the past decade (Vassy 2005).
• Increasingly, however, first-trimester NT screening, particularly in combination with maternal serum screening with biochemical markers, is being proposed.
• ‘Nuchal translucency’ refers to the subcutaneous space between the skin and the cervical spine of the fetus. An increased NT is associated with an increased risk of aneuploidy, particularly trisomies 21 (Down syndrome), 18 (Edwards’ syndrome) and 13 (Patau’s syndrome).
• With the advent of DS screening in the first trimester and the application of nuchal translucency measurements (NT screening) at 10–14 weeks gestation, the potential impact of combining maternal age with fetal nuchal translucency thickness and maternal serum free β-hCG and PAPP-A seems to be increasing.
Figure 35.3 illustrates the rates of use of various diagnostic tests for Down syndrome.
What to tell women
Rowe et al (2006) have found that Australian women participating in prenatal genetic screening are inadequately informed regarding aspects of testing, including the management of pregnancy in the event of an ‘increased risk’ result. Whether or not decisions were informed was not associated with differences in measures of depression and anxiety. They suggested that strategies to improve the quality of education for clinicians so that they are able to convey the necessary complex information effectively and sensitively, and the development of participant information that meets diverse learning capacities and takes into account the effects of pregnancy-related elevations in anxiety, are needed (Rowe et al 2006).
• Explain to women that Down syndrome is caused by the presence of an extra chromosome in a baby’s cells. It occurs by chance at conception and is irreversible. Women are offered screening tests to check whether their baby is likely to have Down syndrome.
• As a midwife you must be able to spend time explaining the implications of Down syndrome, the tests you are offering and what the results may mean. Each woman has the right to choose whether to have all, some or none, of these tests.
• Each woman must be aware that she is under no obligation to have any test and that the decision to have them must be her and her family’s decision.
• It should be explained how to ‘opt out’ of the screening process at any time if she wishes.
• Screening tests will only indicate that a baby may have Down syndrome.
• If the screening test results are positive, the woman should be offered further diagnostic tests to confirm whether her baby does in fact have Down syndrome.
• The time at which the woman is tested will depend on what kinds of tests are used.
• Screening tests for Down syndrome are not always right. They can sometimes wrongly show as positive, suggesting that the baby does have Down syndrome when in fact it does not. This type of result is known as a ‘false-positive’. The number of occasions on which this happens with a particular test is called its ‘false-positive rate’.
• The screening tests available at present have a false-positive rate of less than 5 out of 100 and detect at least 60 out of 100 cases of Down syndrome.
• In Australia, current estimates are that maternal serum screening for biochemical markers of chromosomal abnormality in the second trimester detects about 85% of fetuses with Down syndrome, for a false-positive rate of 6.8% and a positive predictive value of 2%. Approximately 97% of test results are normal (Jaques et al 2006).
Use of maternal age as a primary criterion for offering amniocentesis results in very high rates of use of this invasive test and is a suboptimum use of resources. Every woman’s choice to accept or reject chorionic villus sampling or amniocentesis should be based on counselling that uses the best possible estimate of her personal risk for fetal aneuploidy. Policy advisory groups in other countries should follow the UK initiative, and abandon obsolete guidelines that have advocated offering amniocentesis to all women aged 35 or more, without routinely incorporating serum and ultrasound screening into their risk assessment (Benn 2003; Rowe et al 2006).
Birth anomalies remain a significant public health problem in Australia and are a major reason for admission to hospital during infancy and childhood. They often result in disabilities and handicaps and, in some cases, death (AIHW 2002). An estimated 5% of all Australian births and terminations of pregnancy have a major birth anomaly (Stanley et al 1995), and birth anomalies account for about 13% of the disease burden for children aged 0–14 years (AIHW 1999). Birth anomalies are also a leading cause of infant mortality in Australia, with 25% of infant deaths in 2000 caused by them (Al-Yaman et al 2002).
Birth anomalies are caused by genetic (including chromosomal), environmental and unknown factors, or combinations of these factors. It is estimated that the cause of about 65%–75% of birth anomalies is unknown. About 15%–25% of birth anomalies have a genetic cause and about 10% have an environmental cause (AIHW 2004; Brent 2001).
Neural tube defects
Neural tube defects (NTDs) represent a common group of severe congenital malformations that result from a failure of closure of the embryonic neural tube during early development. Their aetiology is quite complex, involving environmental and genetic factors, and their underlying molecular and cellular pathogenic mechanisms remain poorly understood (Kibar et al 2007). The syndrome includes abnormality of the brain and spinal cord. It can
Box 35.5 Serum markers
Congenital heart defect (CHD) is more prevalent than Down syndrome, with a reported worldwide frequency of 8 of every 1000 births (Ray et al 2005). There is no clear consensus on the definition of CHD (Ray et al 2005); however, it is defined pragmatically as a cardiac defect that could potentially require surgical intervention, intensive medical therapy or prolonged follow-up after birth. Ray and colleagues (2005) observed that less than one-half of cases with increased nuchal translucency have chromosomal abnormality. This is an area of screening in its infancy at present. However, as more biomarkers are established and our preoccupation with reproductive perfection continues, the possibilities are unimagined.
be an isolated abnormality or a part of a genetic syndrome. Genetic studies of NTDs have focused mainly on folate-related genes, based on the finding that perinatal folic acid supplementation reduces the risk of neural tube defects by 60%–70% (Kibar et al 2007) or by 50%–70% (Van der Linden et al 2006).
Neural tube defects are among the most common congenital malformations in humans, affecting 1–2 infants per 1000 births; their incidence varies among different populations (Copp et al 2003).
There are known inconsistencies in the Australian and New Zealand data on rates of NTD, with widely accepted best estimates of 1.32 per 1000 total births for 1999–2003 and 2.56 per 1000 births for 1996–2000 for Indigenous Australians (Bower et al 2006; Dalziel et al 2009). Australian and New Zealand guidelines are similar to those elsewhere (Department of Health [UK] 1992; Institute of Medicine, Food and Nutrition Board [US] 1998), and recommend that women planning or capable of becoming pregnant should consume folic acid as a supplement or in the form of fortified foods at a level of 400 mg/day for at least one month before and three months after conception, in addition to consuming food folate from a varied diet (Australian Government and New Zealand Ministry of Health 2006). The use of periconceptional supplements in Australia is estimated to be about 36%, with lower rates for Indigenous Australian women (Conlin et al 2006).
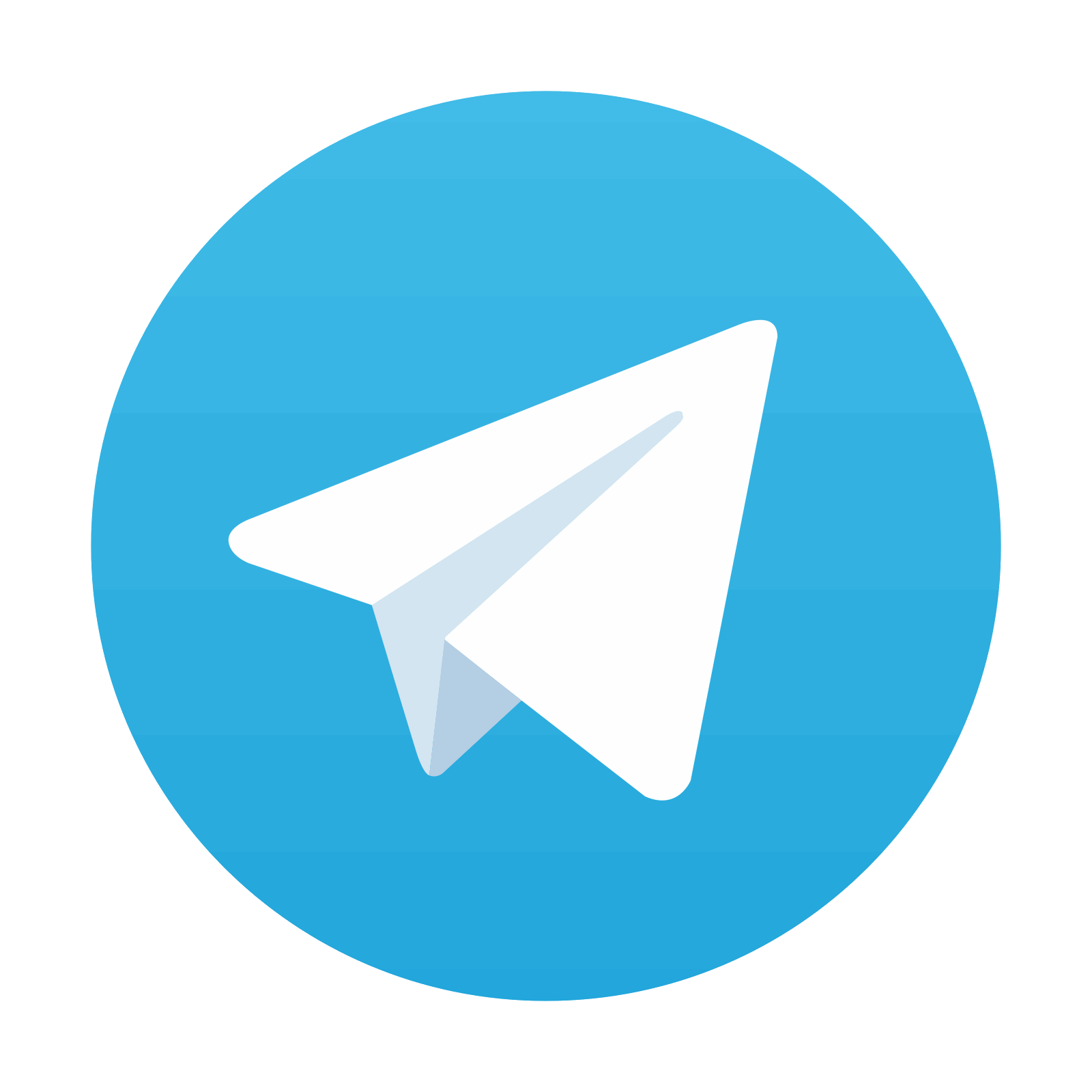
Stay updated, free articles. Join our Telegram channel

Full access? Get Clinical Tree
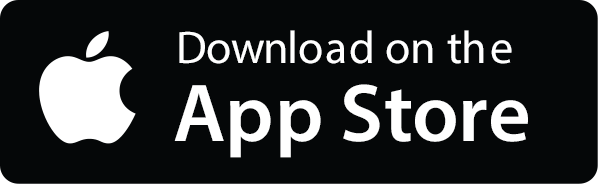
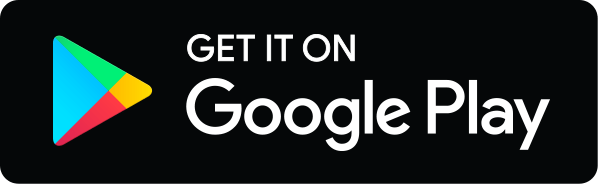