12 Ventilation refers to the cyclical exchange of air in the lungs during inspiration and expiration and is crucial for gaseous exchange to occur. If ventilation is inadequate, then carbon dioxide can accumulate in the blood (hypercapnia) and oxygen levels fall (hypoxaemia). The nurse must be able to appreciate the factors that determine effective ventilation to be able to recognise when this is compromised, and take appropriate action. Inspiration is a mechanical event clearly visible when counting respirations. Whilst this can be voluntary, breathing is regulated unconsciously by the respiratory centre situated in the medulla oblongata (Figure 12.1). Inspiration is initiated by rising levels of CO2 in arterial blood. CO2 diffuses into the cerebrospinal fluid (CSF) of the brain (cerebral ventricles), generating hydrogen (H) ions (Figure 12.1). Hydrogen ions stimulate the central chemoreceptors in the respiratory centre of the medulla oblongata. Peripheral chemoreceptors in the carotid artery and aorta also stimulate breathing but are more sensitive to falling PaO2. Motor impulses generated by the respiratory centre travel along cervical, thoracic and diaphragmatic nerves initiating contraction of the diaphragm and external intercostal muscles, initiating inspiration (Figure 12.1). Thoracic expansion occurs as the rib cage is pulled upwards and outwards and the diaphragm descends (Figure 12.2). As intrapulmonary volume is increased the pressure becomes less than atmospheric pressure (<101 kPa) and air moves into the lungs by bulk flow. Expiration is passive as muscles relax and the lungs recoil inwards (Figure 12.2). In breathless patients, muscle contraction becomes excessive both during both inspiration and expiration. Pulmonary ventilation (VE) airways Pulmonary or minute volume is expired total ventilation (VE) per minute. At rest the volume inhaled with each breath is approximately 500 mL and is called the tidal volume (VT). With a respiratory rate of around 12/min at rest, then the VE is 6000 mL/min (500 × 12). However, not all this air is available for gaseous exchange as the last 150 mL of the VT remains in the anatomical dead space (VD) (conducting zone, Figure 12.3), airway generations 0–16. VE can be increased considerably when required (to as much as 70 L) by increasing respiratory rate and VT, but this utilises more energy and therefore more oxygen. This is the volume of air which is available for gaseous exchange in the respiratory bronchioles, where alveolar ducts and sacs are found. This respiratory zone consists of airway generations 17–23. VA is calculated by first subtracting from the VT the anatomical dead space volume (500 – 150 mL = 350 mL) and then multiplying by the respiratory rate (12): Effective VA ensures PaCO2 and PaO2 remain within normal range. Changes in tidal volume and/or respiratory rate will alter VA. Where tidal volume decreases, for example as in hypoventilation, increased PaCO2 will contribute to a fall in PaO2. When VA is halved then PaCO2 doubles! Additional dead space may occur where, despite air entering an alveolus, there is no corresponding perfusion (no pulmonary capillary blood) in the area. This is called alveolar dead space and represents wasted ventilation and added to the anatomical dead space is called physiological dead space. This may increase considerably in pathological states such as pulmonary embolus or shock Normal breathing in health, with diaphragmatic contraction, requires very little ventilatory effort, accounting for only 3% of total energy expenditure. Work of breathing refers to the work/effort requires to breathe, and is made up of: During inspiration, work is needed to drive air through the airways from the nose to respiratory bronchioles. Air flow through the airways meets varying degrees of resistance where narrowed airway lumen (reduced diameter/increased resistance) requires greater muscular effort to drive air through. Although airway resistance is lower in the small bronchioles (generations 12–23) due to the large cross-sectional area, resistance can be increased by mucus, inflammation and bronchoconstriction. Airway narrowing can cause problems during both inspiration and expiration. Compliance is defined as the volume change per unit of pressure change across the lungs. During inspiration, effort is needed to overcome the density of the lung tissue and chest wall. The greater the compliance the easier it is to move the chest wall and lungs outwards making inspiration easier. Conversely, a reduction in compliance results in stiffness restricting ability of the chest wall and/or lung tissue to expand. Where resistance is increased and/or compliance reduced then the work of breathing is increased. This results in accessory muscle use (e.g. sternocleidomastoid, pectorals, trapezius, internal intercostal and abdominal) with increased energy expenditure and possible muscle fatigue leading to ventilatory failure. In clinical practice, a knowledge and sometimes measurement of various lung volumes can be helpful both in diagnosis and to evaluate therapeutic interventions (Figure 12.4). Volumes such as tidal volume, vital capacity (maximal inspiration followed by maximum expiration) can be easily measured at the bedside with spirometry and peak expiratory flow rate (PEFR) with a peak flow meter (Chapter 14). Alveolar ventilation, residual volume (volume at the end of forced expiration) and function residual capacity (FRC) requires more elaborate methods in a laboratory. Functional residual capacity (FRC) is the volume in the lungs at the end of normal expiration. It is made up of the expiratory reserve volume (ERV) and residual volume (RV). FRC is important in maintaining good oxygenation; if this reduces due to poor lung expansion or positioning, SpO2 will fall. Practitioners should take steps to maximise FRC to minimise alveolar collapse and optimise effective oxygenation. FRC can be reduced by as much as 30% when patients are lying down. Therefore positioning is important; sitting the patient upright (Figure 12.5), or high side-lying, or sitting in a chair are preferable positions. Early mobilisation is effective at helping maintain lung volumes and maximise respiratory status. In high-dependency areas nurses and physiotherapists work collaboratively to ensure that FRC is optimised.
Respiratory physiology: ventilation
Inspiration and expiration
Alveolar ventilation (VA)
Work of breathing
Lung volumes
Functional residual capacity
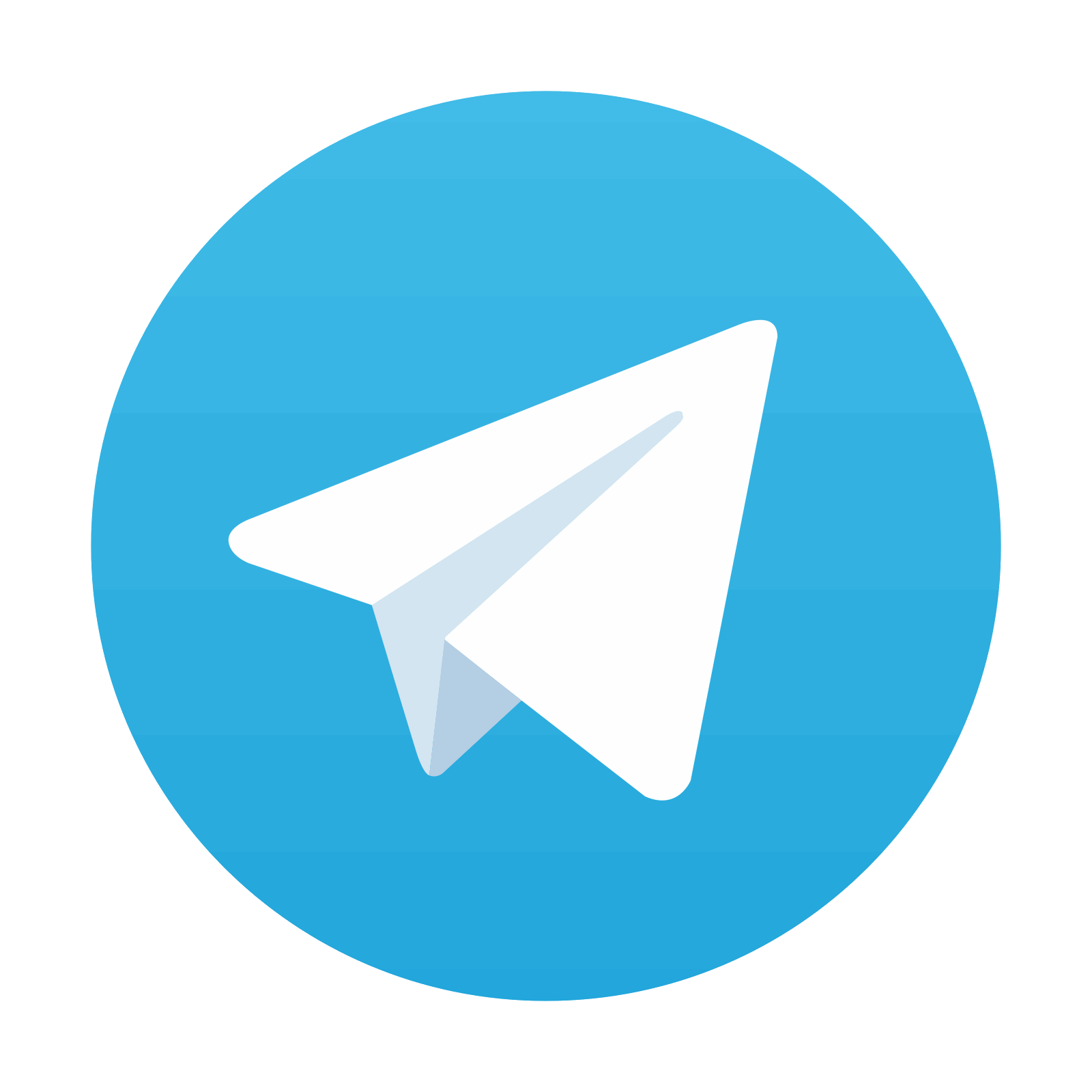
Stay updated, free articles. Join our Telegram channel

Full access? Get Clinical Tree
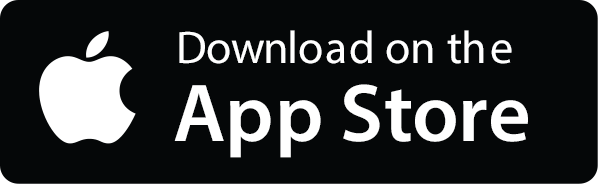
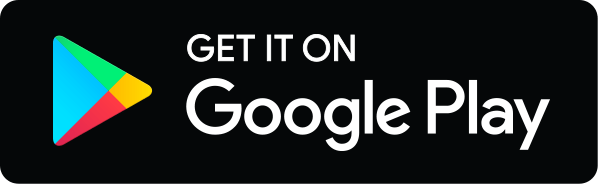