Chapter 7 Renal Health Breakdown
When you have completed this chapter you will be able to
WHAT MAKES UP THE RENAL AND URINARY SYSTEM?
The main organs of the renal system are the kidneys, through which blood plasma is processed and waste products are eliminated as urine via the urinary (accessory) organs, which include the ureters, urinary bladder and urethra (as shown in Figure 7.1). Waste products are formed from the metabolism of nitrogen (food protein) and muscle breakdown (body protein). Nitrogenous waste products, such as urea and creatinine, can be detected in blood and other fluids1. Other major functions of the kidneys include the maintenance of fluid and electrolyte balance (particularly sodium and potassium), acid-base balance, regulation of blood pressure and the production of hormones and enzymes.
The kidneys are located outside the peritoneum on either side of the vertebral column (level of T12 to L3). In each kidney there are approximately one million microscopic functioning units called the nephrons (see Figure 7.2), which are named according to their location in the kidney. Approximately 85% of nephrons are found in the outer cortex region (cortical nephrons) and 15% of nephrons arise in the inner medullary region (juxtamedullary nephrons)2. A network of peritubular capillaries that assist in reabsorption and excretion surrounds each nephron.
REGULATORY FUNCTIONS
GLOMERULAR FILTRATION
The cortex contains the renal corpuscle, which consists of the glomerulus and the surrounding Bowman’s capsule (see Figure 7.2). The glomerulus is composed of a coil of capillaries that are supplied by the afferent arterioles and drained by the efferent arterioles. Each minute approximately 1–1.5L of blood (a quarter of cardiac output) is passed through the two million glomeruli where ultrafiltration takes place3. The speed and pressure of blood flow is determined by hydrostatic pressure (blood pressure). This process of filtration prevents larger molecules such as red blood cells and plasma protein from entering the glomerular filtrate but does include solutes (creatinine, urea, nitrogen and glucose), water and electrolytes1. Each day about 180 L of glomerular filtrate is formed and a normal glomerular filtration rate (GFR) averages about 120–125mL/min in adults in normal conditions. The next part of the process involved in urine formation is reabsorption and occurs in the tubular system, where more than 99% of all filtered water is reabsorbed into the body again by the tubules.
TUBULAR REABSORPTION
Urine formation involves tubular reabsorption that occurs by both active and passive transport mechanisms. The tubular system extends from the glomerulus and consists of three parts: a proximal tubule, the loop of Henle and a distal tubule, all of which are critical to kidney function. The proximal convoluted tubule descends from the renal corpuscle down into the medulla of the kidney and is responsible for increasing the surface area available for reabsorption of almost 70% of the sodium, potassium and water contained in the glomerular filtrate and approximately 50% of urea4. Other substances that are reabsorbed include chloride, glucose, amino acids, phosphate and bicarbonate5. The reabsorbed filtrate is transported by either active or passive mechanisms into the peritubular capillary network. The proximal tubular system leads on to the first section of the Henle’s loop in the medulla.
Henle’s loop consists of a descending and an ascending limb and is an important part of the renal tubule because of its role in the production of either concentrated or dilute urine. This process occurs because of the kidney’s countercurrent mechanism that is caused by the contents in the descending limb flowing in an opposite direction to the contents in the ascending limb. The main functions of the Henle’s loop have to do with the reabsorption of water that occurs in the thicker portion of the descending loop. The active reabsorption of sodium and chloride occurs in the thinner portion of the ascending loop2. This reabsorption of sodium makes the tubule fluid more dilute. Urea is removed by diffusion in the descending loop. Henle’s loop joins into the distal tubule that is situated in the renal cortex, where further concentration of the filtrate occurs5.
The cells in the distal tubule and their collecting ducts, allow some sodium to be removed by active transport with the assistance of aldosterone (a hormone of the adrenal cortex), but not water6. If this were allowed to continue the urine would become too dilute (hypotonic) and lead to dehydration. In order to prevent this from occurring the body’s regulatory mechanism produces antidiuretic hormone (ADH) that causes these cells to become more permeable to water. This means that the water is able to pass into the interstitial fluid and so maintain equilibrium.
TUBULAR SECRETION
A third mechanism involved in the formation of urine is tubular secretion, which is the opposite process to reabsorption. During this process substances (ammonium ions, hydrogen, and potassium ions) move from the peritubular capillaries through the cells that line the tubule wall into the tubular fluid. Potassium or hydrogen is actively transported from the blood to the tubular fluid in exchange for sodium that diffuses back into the blood. The secretion of potassium increases when the concentration of aldosterone, in the blood increases, which is an important process in the removal of excessive levels of potassium. Aldosterone, is the only hormone involved in the regulation of potassium4. A high level of aldosterone in the plasma causes the tubules to increase their reabsorption of sodium and water, which then means that less sodium and water is eliminated in the urine. If the aldosterone level in the plasma is low then the excretion of sodium and water is increased. Certain drugs, such as penicillin, are also secreted from the tubular cells6. The distal tubules then combine to form collecting ducts.
FURTHER FUNCTIONS OF THE KIDNEYS
Aside from removing toxins from the blood, eliminating waste products and regulating fluid and electrolyte balance, the kidneys also have an important role in the regulation of blood pressure, vitamin D and calcium and the production of red blood cells.
REGULATION OF VITAMIN D AND CALCIUM
The kidneys regulate calcium and phosphate balance by converting the inactive form of vitamin D to an active form. Activated vitamin D regulates the secretion of parathyroid hormone and calcium concentration. Parathyroid hormone increases calcium absorption and decreases phosphate3.
PRODUCTION OF RED BLOOD CELLS
The kidneys produce over 80% of erythropoietin (EPO) a glycoprotein hormone, which has a major role in red blood cell production and the prevention of anaemia. In a hypoxic state, the kidneys secrete EPO, which then directs the bone marrow to produce proerythroblasts, which then develop into erythrocytes7. The production of EPO is decreased when the oxygen supply to the tissues is corrected.
SUMMARY OF KEY CONCEPTS
ACUTE RENAL FAILURE
WHAT DOES IT MEAN?
Acute renal failure (ARF) is a rapid and sudden deterioration of renal function and results in the retention of metabolic wastes (azotaemia) and impaired fluid and electrolyte balance5. It usually develops over hours or days and usually follows severe, prolonged hypotension or hypovolaemia or exposure to a nephrotoxic agent. ARF is usually accompanied by oliguria (urine output less than 400mL in 24 hours), although some people have nonoliguric ARF and maintain urine output of greater than 400mL in 24 hours. Rarely does anuria (urine output less than 100 mL in 24 hours) occur. Unlike chronic renal failure, ARF is potentially reversible if the precipitating factors can be removed or corrected before permanent kidney damage has occurred. Despite advances in renal replacement therapies, ARF continues to have a mortality rate of approximately 50%8.
WHAT IS THE PATHOPHYSIOLOGY?
Acute renal failure can be caused by many types of conditions, including a reduction in blood flow to the kidney; toxic injury within the kidney; and obstruction of urine outflow from the kidney. The causes of ARF are commonly categorised as prerenal (55–60%), intrarenal (35–40%), and postrenal (<5%)8.
PRE-RENAL FAILURE
Prerenal ARF results from any external factors that cause a sudden and severe reduction in blood flow to the kidneys and subsequent reduction in glomerular perfusion and filtration rate. The causes of prerenal failure can be broadly classified as conditions causing hypovolaemia, reduction in cardiac output, vasodilation, and obstruction of renal blood vessels. Table 7.1 summarises the causes of prerenal ARF.
TABLE 7.1 CAUSES OF PRERENAL ACUTE RENAL FAILURE
Hypovolaemia/Fluid Volume Loss |
Inadequate Cardiac Output |
Vasodilatation |
Obstruction of Renal Blood Vessels |
Normally the kidneys receive approximately 20% of the cardiac output, and this is required to efficiently remove metabolic waste products and to regulate body fluids and electrolytes. Several protective mechanisms are in place to minimise the effect of reduced renal blood flow on renal tissue. These include the kidney’s ability to: 1) autoregulate blood flow to preserve renal perfusion; 2) activate the renin-angiotensin-aldosterone mechanism to promote sodium retention; and 3) stimulate the secretion of anti-diuretic hormone from the pituitary gland to encourage the distal convoluted tubules and collecting ducts to conserve water. It is the ability of the kidney to retain sodium and water that allows prerenal failure to be separated from intrarenal and post-renal ARF9.
In prerenal ARF, the reduction in renal blood flow is so profound it renders these protective mechanisms as ineffective, and severely diminishes the ability of the glomeruli to filter these wastes. Prolonged renal hypoperfusion also decreases the availability of nutrients and oxygen needed for basic cellular function and this can lead to tubular ischaemia (see acute tubular necrosis).
INTRA-RENAL FAILURE
Conditions that directly injure functional renal tissue (glomerulus, tubules and interstitium) are classified as intrarenal causes of ARF (see Table 7.2). The most common cause of intrarenal ARF is acute tubular necrosis8. Less common causes are acute types of glomerulonephritis (i.e., Goodpasture’s syndrome), vasculitis, systemic lupus erythematosus and haemolytic uraemic syndrome. Acute pyelonephritis can also be responsible for the development of intrarenal ARF.
TABLE 7.2 CAUSES OF INTRA-RENAL FAILURE
Acute Tubular Necrosis |
Glomerular or Vascular Dysfunction |
Nephrotoxic Substances |
• Drugs (aminoglycosides, amphotericin B, vancomycin, rifampicin, cisplatin, cyclosporin, frusemide, methotrexate, non-steroidal anti-inflammatory agents) |
Miscellaneous |
ACUTE TUBULAR NECROSIS
Acute tubular necrosis (ATN) is a type of intrarenal ARF caused by ischaemia, nephrotoxic effects of drugs, intratubular obstruction (e.g., myoglobin pigments) or toxins released from severe sepsis5,10. Ischaemic and nephrotoxic ATN are responsible for 90% of intrarenal ARF cases9. Those at greatest risk of developing ATN include the elderly, diabetics, and patients who have a history of renal insufficiency11. ATN typically develops following prolonged ischaemia when perfusion to the kidney is considerably reduced. In some patients, ATN can eventuate after only a few hours of hypovolaemia or hypotension. The ischaemia associated with ATN predominantly damages the proximal tubule. The kidney is also susceptible to toxic injury because of its high blood flow, its mechanism for concentrating drugs and toxins, and its cellular structure5. Nephrotoxic agents may cause approximately 20% of all cases of ARF10.
The renal protective mechanisms of autoregulation of renal blood vessels and activation of the renin-angiotensin-aldosterone system are able to increase renal perfusion during the early stages of ARF8. If, however, the blood flow is reduced for longer than one hour, these protective mechanisms begin to weaken, triggering a variety of factors that result in the development of ATN. These factors include: 1) decreased glomerular filtration rate and permeability, 2) medullary tissue hypoxia and cellular oedema, 3) intratubular obstruction, and 4) backleak of filtrate into the interstitium8. All of these factors cause a decrease in urine output.
POST-RENAL FAILURE
Post-renal ARF results from an obstruction of urine outflow from the kidneys (see Table 7.3). The obstruction can occur in the kidneys (e.g., renal cell carcinoma), ureter (e.g., renal calculi), bladder (e.g., cancer), or urethra (e.g., prostatic hypertrophy)12. Obstruction can also result from iatrogenic causes such as blocked or kinked indwelling urinary catheters or from inadvertent surgical ligation of ureters. Prostatic hypertrophy is the most common underlying problem12.
TABLE 7.3 CAUSES OF POST-RENAL FAILURE
Urinary tract obstruction causes an increase in pressure proximal to the obstruction that often leads to dilation of the proximal collecting system (i.e., hydroureter and hydronephrosis). The elevated pressure in the collecting system is transmitted back into the tubular network and eventually stops glomerular filtration. The extent of damage depends on the degree, duration, and location of the obstruction as well as the presence and severity of infection9. Postrenal ARF is a urological emergency, and if the obstruction is not relieved, permanent renal damage can occur.
WHAT ARE THE CLINICAL MANIFESTATIONS?
Prerenal and postrenal ARF resolve relatively easily when they are identified early and treatment is commenced quickly10. Intrarenal failure and ATN have a prolonged course of recovery because actual parenchymal damage has occurred. Clinically, ARF usually progresses through four phases: initiating, oliguric, diuretic, and recovery. In some situations, the patient does not recover from ARF, and chronic kidney disease results.
OLIGURIC PHASE
The most common initial feature of ARF is the development of oliguria (<400mL of urine in 24 hours). Oliguria usually occurs within 1 to 7 days of the causative event. If the cause is ischaemia, oliguria may occur within 24 hours. When nephrotoxic drugs are involved, the onset may be delayed for up to a week. About 50% of patients will not demonstrate oliguria, making the initial diagnosis more difficult. The oliguric phase lasts for approximately 10 to 14 days but can last months in some cases. The longer the oliguric phase persists, the poorer the prognosis for recovery of complete renal function9.
It is important to distinguish prerenal oliguria from the oliguria of intrarenal ARF during this phase. Measurements of serum urea and creatinine levels are essential in patients who have ARF. If the ratio of urea:creatinine is more than 20:1, a prerenal cause of ARF is most likely13. In addition, urine with a high specific gravity (>1.015) and a low sodium concentration (<20mmol/L) is characteristic of prerenal ARF14. This reflects the protective mechanisms of salt and water retention described earlier. In contrast, oliguria due to intrarenal failure is characterised by urine with a normal or fixed specific gravity (1.010) and a high sodium concentration (>40mmol/L), indicating that the injured tubules cannot respond to protective mechanisms and lose sodium. Similarly, the oliguria of intrarenal failure caused by ATN is characterised by the presence of casts in the urine. These casts are formed from necrotic renal tubular epithelial cells, which detach or slough off into the tubules. The presence of protein on dipstick urinalysis may also be suggestive of intrarenal ARF due to glomerular damage15. It is during this phase that the clinical manifestations of significant renal impairment become apparent. These are described in more detail later in this chapter (see chronic renal failure).
DIURETIC PHASE
Over the next one to three weeks, there is a gradual repair and regeneration of renal tissue9. The diuretic phase begins with a gradual increase in daily urine output from 1L to 3L per day, and the urine volume may reach 3L to 5L or more per day. Although urine output is increasing, the nephrons are still not fully functional. An osmotic diuresis occurs due to salt and water accumulation in extracellular spaces as well as from high urea concentration in glomerular filtrate. The inability of the tubules to concentrate urine results in an increased urine volume. Hypovolaemia and hypotension can occur from massive fluid losses. Large losses of electrolytes, such as sodium, potassium also occur during this phase14. The kidneys have recovered their ability to excrete wastes, but not to concentrate urine. Near the end of this phase the patient’s acid-base, electrolyte, and waste product (urea, creatinine) values begin to normalise.
WHAT SHOULD YOU BE LOOKING AT IN THE LABORATORY AND OTHER TESTS?
A thorough history is essential for diagnosing ARF. Prerenal causes should be considered when there is a history of dehydration, blood loss, or severe heart disease. Intrarenal causes may be suspected if the patient has been taking potentially nephrotoxic drugs5 or has a recent history of prolonged hypotension or hypovolaemia. Postrenal ARF is suggested by a history of changes in urinary stream, stones or benign prostatic hypertrophy.
WHAT IS THE TREATMENT?
ARF is potentially reversible if detected early. The primary goals of treatment are to eliminate the cause, manage the signs and symptoms, and prevent complications while the kidneys recover14. The first step is to determine if there is adequate intravascular volume and cardiac output to ensure adequate perfusion of the kidneys. This is assessed by the administration of volume expanders (Gelofusin®) or bolus amounts of crystalloids along with diuretic therapy, e.g., frusemide. If ARF is already established, forcing fluids and diuretics will not be effective and may, in fact, result in life-threatening fluid overload and pulmonary oedema. Conservative therapy involving dietary (e.g., sodium, potassium and protein) and fluid restrictions (i.e., 500mL plus previous day’s urine output), and medications (e.g., resonium, antihypertensive agents, phosphate binders, sodium bicarbonate supplements) may be all that is necessary to control the multi-systemic effects of ARF until renal function improves8.
Hyperkalaemia, severe uraemia, pulmonary oedema and uraemic pericarditis all indicate that conservative measures are no longer able to control the symptoms of ARF and there is now a need for urgent renal replacement therapy (RRT)16. If RRT is required, three options are available: haemodialysis (HD), continuous venovenous haemodiafiltration (CVVH) and peritoneal dialysis (PD)16. HD is preferred for the hypercatabolic patient and for the individual who has had abdominal or thoracic trauma or surgery. HD is the method of choice when rapid changes are required in a short time especially if life-threatening symptoms have arisen (e.g., hyperkalaemia >6.5mmol/L, pulmonary oedema). HD is technically more complicated because specialised staff, equipment and vascular access are required. In the haemodynamically unstable patient, CVVH provides gradual removal of excess fluid and solutes, and is, therefore, better tolerated by patients with ARF. It is technically similar to HD and is frequently used in the intensive care setting. PD is much simpler than HD, but it carries the risk of peritonitis, is less efficient, requires longer treatment times, and is not commonly used for ARF.
The patient’s overall health, the severity of renal failure, and the number and type of complications influence the outcome of ARF. The majority of patients with ARF have potentially recoverable renal function. At least 60% of patients have full recovery of renal function, approximately 30% have mild to moderate renal impairment and only 6–10% progress to end-stage renal failure and require permanent RRT8. In addition, the older adult patient is less likely to recover full kidney function than the younger patient.
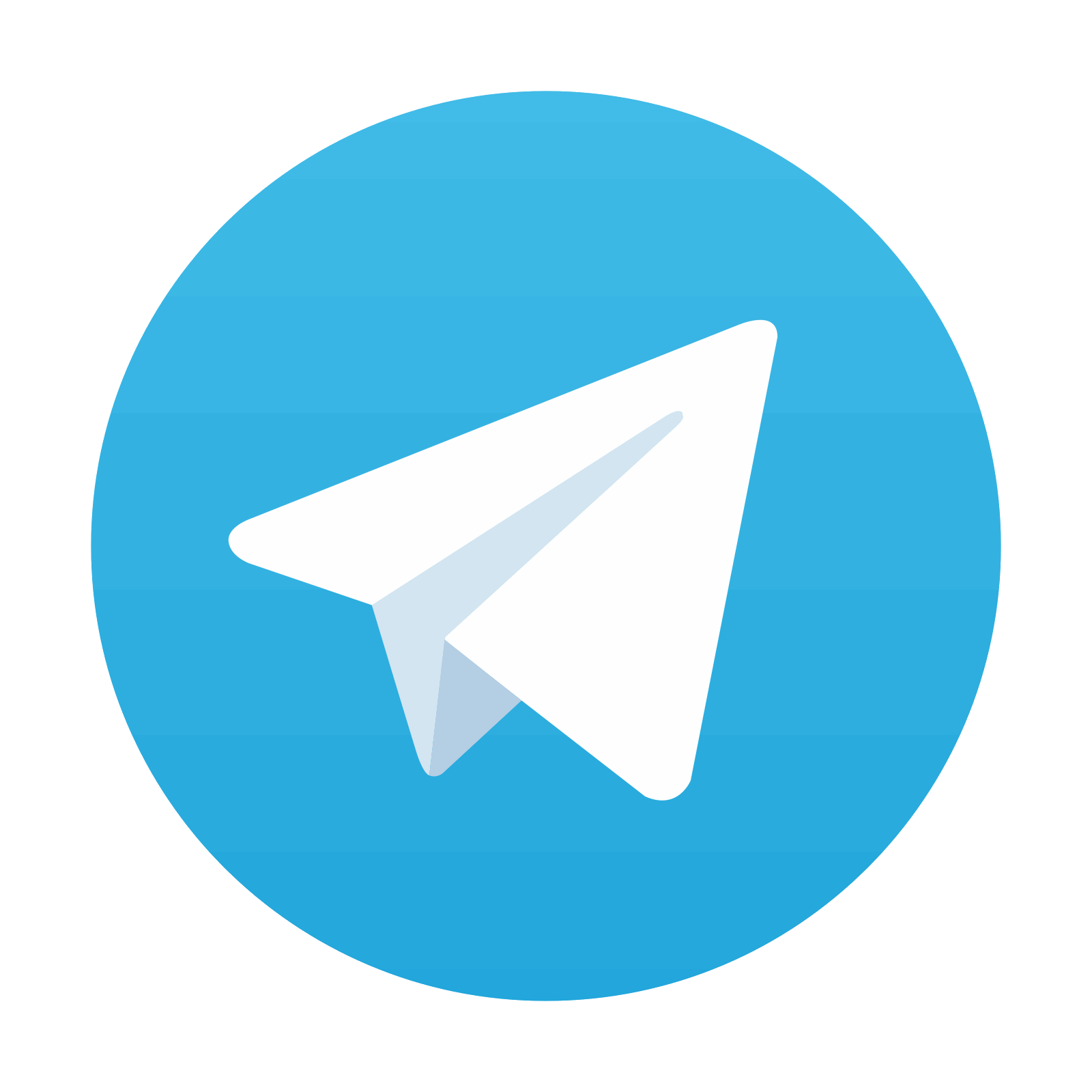
Stay updated, free articles. Join our Telegram channel

Full access? Get Clinical Tree
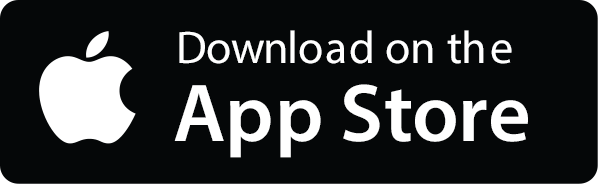
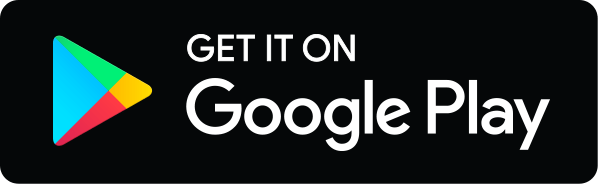