Physiologic Changes of Pregnancy
Susan Tucker Blackburn
The pregnant woman experiences dramatic physiologic changes to meet the demands of the developing fetus, maintain homeostasis, and prepare for birth and lactation. Maternal adaptations during pregnancy result from the interplay of multiple factors, including the influences of reproductive and other hormones, growth factors, cytokines, and other signaling proteins, as well as mechanical pressures exerted by the growing fetus and enlarging uterus. An understanding of the normal physiologic changes of pregnancy is essential for discriminating between normal and abnormal states. Laboratory values and physical findings considered normal in the nonpregnant woman may not be normal for women during pregnancy. This chapter reviews physiologic changes during pregnancy to provide baseline information to guide the perinatal nurse in conducting an accurate and thorough assessment of the pregnant woman.
HORMONES AND OTHER MEDIATORS
Many of the physiologic changes during pregnancy are mediated by hormones. Hormones are responsible for maintaining homeostasis, regulation of growth, and development and cellular communication. They are transported by the blood from the site of production to their target cells throughout the pregnant woman’s body and are responsible for many physiologic adaptations to pregnancy. During pregnancy, the placenta serves as an endocrine gland, secreting many hormones, growth factors, and other substances. The major hormones produced by the placenta are human chorionic gonadotropin (hCG), human placental lactogen (hPL), estrogen, and progesterone. However, the placenta also produces pituitary-like and gonad-like hormones (e.g., placental corticotropin, human chorionic thyrotropin, placental growth hormone), hypothalamus-like releasing hormones (e.g., human chorionic somatostatin, corticotropin-releasing hormone [CRH], gastrointestinal-like hormones (e.g., gastrin, vasoactive intestinal peptide), and parathyroid hormone-related protein (PTHrP) (Blackburn, 2012; Liu, 2009). The placental hormones are critical for many of the metabolic and endocrine changes during pregnancy. For example, PTHrP mediates placental calcium transport and fetal bone growth, and CRH release of prostaglandins (PGs) and has a major role in initiation of myometrial contractility and labor onset.
The placenta, membranes, and fetus also synthesize peptide growth factors such as epidermal growth factor, nerve growth factor, platelet-derived growth factor, transforming growth factor-β (TGF-β), skeletal growth factor, and insulin-like growth factor-I (IGF-I) and II (IGF-II) (Liu, 2009). These growth factors stimulate localized hormone release, regulate cell growth and differentiation, and enhance metabolic processes during pregnancy (Blackburn, 2012). For example, IGF-I and IGF-II enhance amino acid and glucose uptake and prevent protein breakdown, thus helping to regulate cell proliferation and differentiation to maintain fetal growth. TGF-β stimulates cell differentiation and is important in embryogenesis and neural migration and differentiation (Liu, 2009).
HUMAN CHORIONIC GONADOTROPIN
hCG is a glycoprotein with alpha and beta subunits. In early pregnancy, the beta subunits predominate, whereas the alpha units are more prevalent in late pregnancy (Cole, 2010). Assessment of free beta subunits is a component of first- and second-trimester maternal serum screening for fetal genetic disorders
(Nicolaides, 2011). hCG is secreted primarily by the placenta. The major function of hCG is to maintain progesterone and estrogen production by the corpus luteum until the placental function is adequate (about 10 weeks postconception). hCG may enhance uterine growth, maintenance of uterine quiescence, and immune function during pregnancy. hCG is also thought to have a role in fetal testosterone and corticosteroid production and angiogenesis (Cole, 2010). hCG is found in the blastocyst prior to implantation and is detected in maternal serum and urine around the time of implantation (7 to 8 days after ovulation) (Liu, 2009). Levels increase to peak about 60 to 90 days after conception, then decrease rapidly after 10 to 12 weeks (when the placenta becomes the main producer of estrogens and progesterone and the corpus luteum is no longer needed) to a nadir at 100 to 130 days (Liu, 2009). Maternal urine pregnancy testing assesses hCG levels and positive results are found by 3 weeks after conception (about 5 weeks after the last normal menstrual period) (Moore, Persaud, & Torchia, 2011). hCG levels are elevated in multiple and molar pregnancies and low with ectopic pregnancy or abnormal placentation (Liu, 2009).
(Nicolaides, 2011). hCG is secreted primarily by the placenta. The major function of hCG is to maintain progesterone and estrogen production by the corpus luteum until the placental function is adequate (about 10 weeks postconception). hCG may enhance uterine growth, maintenance of uterine quiescence, and immune function during pregnancy. hCG is also thought to have a role in fetal testosterone and corticosteroid production and angiogenesis (Cole, 2010). hCG is found in the blastocyst prior to implantation and is detected in maternal serum and urine around the time of implantation (7 to 8 days after ovulation) (Liu, 2009). Levels increase to peak about 60 to 90 days after conception, then decrease rapidly after 10 to 12 weeks (when the placenta becomes the main producer of estrogens and progesterone and the corpus luteum is no longer needed) to a nadir at 100 to 130 days (Liu, 2009). Maternal urine pregnancy testing assesses hCG levels and positive results are found by 3 weeks after conception (about 5 weeks after the last normal menstrual period) (Moore, Persaud, & Torchia, 2011). hCG levels are elevated in multiple and molar pregnancies and low with ectopic pregnancy or abnormal placentation (Liu, 2009).
HUMAN PLACENTAL LACTOGEN
hPL, also known as human chorionic somatomammotropin, is produced by the syncytiotrophoblast tissues of the placenta. Maternal serum hPL levels increase parallel to placental growth and peak near term. hPL is critical to fetal growth because it alters maternal protein, carbohydrate, and fat metabolism and acts as an insulin antagonist (Liu, 2009). This hormone increases free fatty acid availability for maternal metabolic needs and decreases maternal glucose uptake and use. Thus, glucose (the major fetal energy substrate) is reserved for fetal use, and free fatty acids are used preferentially by the mother (Blackburn, 2012). This preferred breakdown of free fatty acids increases levels of ketones and the risk of ketosis with significant decreased maternal food intake.
ESTROGENS
Estrogens (estrone, estradiol, and estriol) are steroid hormones secreted by the ovaries during early pregnancy and the placenta for most of pregnancy. Estrogen prevents further ovarian follicular development during pregnancy. Both luteinizing hormone (LH) and follicle-stimulating hormone (FSH) are inhibited by high concentrations of progesterone in the presence of estrogen. Estrogen affects the renin-angiotensin-aldosterone system and stimulates production of hormone-binding globulins in the liver during pregnancy. Estrogen also helps prepare the breasts for lactation, increases blood flow to the uterus, stimulates the growth of the uterine muscle mass, enhances myometrial activity, and is involved in the timing of the onset of labor (Cunningham, Leveno, Bloom, & Hauth, 2009).
Estriol is the primary estrogen produced by the placenta during pregnancy. Production of estriol involves interaction of the mother, fetus, and placenta. The mother provides cholesterol and other precursors, which are metabolized by the placenta. These metabolites are sent to the fetus for further processing by the fetal liver and adrenal gland to produce dehydroepiandrosterone sulfate, which is sent back to the placenta to produce estriol. Maternal serum and urinary estriol levels increase throughout pregnancy, with a rapid rise during the last 6 weeks. This late increase in estriol alters the local (uteroplacental area) ratio of estrogens to progesterone, which is a factor in the onset of labor (Cunningham et al., 2009; Norwitz & Lye, 2009).
PROGESTERONE
Progesterone is initially produced by the corpus luteum in early pregnancy and later primarily by the placenta. Progesterone maintains decidual secretory activities required for implantation and helps to maintain myometrial relaxation by acting on uterine smooth muscle to inhibit PG production and down-regulating contraction-associated proteins such as gap junctions and PG and oxytocin receptors (Blackburn, 2012). Progesterone acts on smooth muscle in other areas of the body as well, especially in the gastrointestinal (GI) and renal system; relaxes venous walls to accommodate the increase in blood volume; alters respiratory center sensitivity to carbon dioxide; and aids in the development of acini and lobules of the breasts in preparation for lactation (Cunningham et al., 2009). Progesterone also mediates changes in immune function during pregnancy, which helps prevent rejection by the mother of the fetus and placenta as foreign antigens (Challis et al., 2009).
RELAXIN
Relaxin is secreted by the corpus luteum and later by the myometrium and placenta. Relaxin interacts with other mediators to inhibit uterine activity, thereby maintaining myometrial quiescence during pregnancy and diminishing the strength of uterine contractions. Relaxin also plays a role in cervical ripening and may help suppress oxytocin release during pregnancy (Goldsmith & Weiss, 2009).
PROSTAGLANDINS
PGs are found in high concentrations in the female reproductive tract and in the decidua and fetal membranes during pregnancy. PGs are part of a family of
substances called eicosanoids that are synthesized from arachidonic acid, which is present in plasma membrane phospholipids. This family includes PGs, prostacyclins (PGI2s), thromboxanes, and leukotrienes. Eicosanoids are released quickly with plasma membrane stimulation and act near the site of release. PGs affect smooth muscle contractility. The interplay between thromboxanes and PGI2 is believed to contribute to hypertensive disorders in pregnancy. PGI2 release is mediated by nitric oxide, which regulates vascular tone and is an important mediator of reduced vascular resistance and myometrial relaxation during pregnancy (Blackburn, 2012).
substances called eicosanoids that are synthesized from arachidonic acid, which is present in plasma membrane phospholipids. This family includes PGs, prostacyclins (PGI2s), thromboxanes, and leukotrienes. Eicosanoids are released quickly with plasma membrane stimulation and act near the site of release. PGs affect smooth muscle contractility. The interplay between thromboxanes and PGI2 is believed to contribute to hypertensive disorders in pregnancy. PGI2 release is mediated by nitric oxide, which regulates vascular tone and is an important mediator of reduced vascular resistance and myometrial relaxation during pregnancy (Blackburn, 2012).
PGs mediate the onset of labor, myometrial contractility, and cervical ripening. Throughout most of pregnancy, myometrial PG receptors are down-regulated (Norwitz & Lye, 2009). Changes in receptor responsivity and increases in PG receptors and levels of stimulatory PG near the end of pregnancy are mediated by CRH, fetal cortisol, and uterine stretch (Romero & Lockwood, 2009). PGs play a critical role in labor onset via a variety of mechanisms, including formation of gap junctions (needed to transmit action potentials) and oxytocin receptors in the myometrium, increasing frequency of action potentials, stimulating myometrial contractility, and enhancing calcium availability (calcium is essential for smooth muscle contraction) (Norwitz & Lye, 2009; Romero & Lockwood, 2009). The physiologic roles of endogenous PGs have led to the use of various PGs for cervical ripening and induction of labor (Kelly, Malik, Smith, Kavanagh, & Thomas, 2009).
PROLACTIN
Prolactin is released from the anterior pituitary. This hormone is responsible for the increase in and maturation of ducts and alveoli in the breasts and for initiation of lactation after birth. During pregnancy, there is a marked increase of prolactin secondary to the effects of angiotensin II, gonadotropin-releasing hormone, and arginine vasopressin (AVP) on the pituitary. However, the high estrogen levels throughout pregnancy inhibit initiation of lactation. After birth, this inhibition quickly disappears with removal of the placenta, the major source of estrogen during pregnancy. The anterior pituitary begins to produce larger amounts of prolactin, which stimulate the breast to begin lactation. The serum prolactin concentration begins to rise in the first trimester and by term may reach 10 times the nonpregnant concentration (Liu, 2009). After birth, prolactin levels rise rapidly, returning to prepregnancy levels by 7 to 14 days in nonbreastfeeding mothers (Molitch, 2009). In lactating women, baseline prolactin levels are elevated further during sucking; the baseline decreases over the first months of lactation.
CARDIOVASCULAR SYSTEM
The cardiovascular system undergoes numerous and profound adaptations during pregnancy (Table 3-1). Cardiovascular anatomy, blood volume, cardiac output, and vascular resistance are altered to accommodate the additional maternal and fetal circulatory requirements. Increased ventricular wall muscle mass, an increased heart rate, cardiac murmurs, and dependent peripheral edema are evidence of these anatomic and functional changes. Physical symptoms may occur during pregnancy in response to normal cardiovascular changes. Some women report palpitations, light-headedness, or decreased tolerance for activity. Cardiovascular adaptations have a significant impact on all organ systems. Women with normal cardiovascular function are generally able to accommodate the dramatic cardiovascular changes associated with pregnancy. Women with cardiovascular disease are at increased risk for complications during pregnancy, labor, or the immediate postpartum period, in part because of alterations in blood and plasma volume and cardiac output (Curry, Swan, & Steer, 2009).
HEART
The position, appearance, and function of the heart change during pregnancy. As the growing uterus exerts pressure on the diaphragm, the heart is displaced upward, forward, and to the left, to lie in a more horizontal position. The first-trimester increase in ventricular muscle mass and the second- and early third-trimester increase in end-diastolic volume cause the heart to undergo a physiologic dilation (Monga, 2009). The point of maximal impulse is deviated to the left at the fourth intercostal space. During the first few days postpartum, the left atrium also appears to be enlarged because of the increased blood volume that occurs with removal of the placenta.
Table 3-1. CARDIOVASCULAR CHANGES DURING PREGNANCY | ||||||||||||||||||||
---|---|---|---|---|---|---|---|---|---|---|---|---|---|---|---|---|---|---|---|---|
|
Maternal heart rate begins to increase at 4 to 5 weeks’ gestation and peaks at about 32 weeks at 10 to 20 beats/min above baseline (Hegewald & Crapo, 2011; Monga, 2009). Heart rate and atrial size return to normal prepregnancy values in the first 10 days postpartum, whereas left ventricular size normalizes after 4 to 6 months (Cunningham et al., 2009; Monga, 2009).
Between 12 and 20 weeks, a change in heart sounds and a systolic murmur is heard in approximately 90% to 95% of pregnant women because of the increased cardiovascular load (Monga, 2009). Ninety percent of pregnant women have a wider split in the first heart sound (which also becomes louder) and an audible third heart sound. Around 30 weeks’ gestation, the second heart sound also demonstrates an audible splitting. About 5% of pregnant women also have an audible fourth heart sound. Systolic murmurs are auscultated in more than 95% of pregnant women during the last two trimesters. However, systolic murmurs greater than grade 2/4 and any type of diastolic murmur are considered abnormal (Monga, 2009). Systolic murmurs can be best auscultated along the left sternal border and result from aortic and pulmonary artery blood flow (Blackburn, 2012).
BLOOD VOLUME
Blood volume increases approximately 30% to 50% beginning as early as 6 weeks and peaking at 28 to 34 weeks (Monga, 2009). Blood volume then plateaus or decreases slightly to term, returning to prepregnant values by 6 to 8 weeks postpartum or sooner (Monga, 2009). The increased blood volume is necessary to provide adequate blood flow to the uterus, fetus, and maternal tissues; to maintain blood pressure (BP); to assist with temperature regulation by increasing cutaneous blood flow; and to accommodate blood loss at birth (Blackburn, 2012). Failure of blood volume to increase is associated with altered fetal and placental growth. Blood volume is greater in multiple gestations and increases proportionally according to the number of fetuses (Monga, 2009).
Changes in blood volume are due to increases in both plasma volume and red blood cell (RBC) mass. Plasma volume increases approximately 50% (range, 40% to 60% or about 1,200 to 1,600 mL) by term, and red cell mass increases 20% to 30% (250 to 450 mL) (Monga, 2009). The rapid increase in plasma volume and later rise in RBC volume results in relative hemodilution. Even with increased RBC production, there is a decrease in both hemoglobin and hematocrit values during pregnancy.
One proposed mechanism for expansion of blood volume is hormonal stimulation of plasma renin activity and aldosterone levels. Increases in the renin-angiotensin-aldosterone system stimulate renal tubular reabsorption of sodium and a subsequent increase of 6 to 8 L in total body water (extracellular and plasma fluid volume) (Blackburn, 2012). Changes in systemic vascular resistance (SVR) decrease venous tone and increase the capacity of the blood vessels to accommodate the extra blood volume without overloading the maternal system.
The extra blood volume helps protect the woman from shock with the normal blood loss at birth. To prevent hemorrhage immediately after childbirth, the uterus contracts, shunting blood from uterine vessels into the systemic circulation and causing an autotransfusion of approximately 1,000 mL. Although up to 500 mL (10%) of blood may be lost with a vaginal birth and 1,000 mL (15% to 30%) with a cesarean birth, average loss is usually less. These changes are accompanied by a postpartum diuresis that further reduces the plasma volume during the first several days postpartum. Plasma volume returns to prepregnancy levels by 6 to 8 weeks and perhaps as early as 2 to 3 weeks postpartum (Blackburn, 2012).
CARDIAC OUTPUT
Cardiac output is the product of heart rate times stroke volume, both of which increase during pregnancy. Cardiac output is also influenced by blood volume, cardiac contractility, vascular resistance, and maternal position. Cardiac output increases 30% to 50% during pregnancy when measured in the left lateral recumbent position. This increase begins early, with approximately half of the increase occurring by 8 weeks’ gestation; peaks in the second trimester; and then plateaus until term (Monga, 2009). In early pregnancy, the increase in cardiac output primarily results from an increase in stroke volume. Stroke volume increases by as early as 8 weeks, peaks at 16 to 24 weeks, and then declines to term. The increase in cardiac output results initially from the increase in both heart rate and stroke volume, but by late pregnancy is due primarily to the changes in heart rate, which continues increasing to term (Monga, 2009). Cardiac output increases are greater in multiple pregnancies, especially after 20 weeks’ gestation (Blackburn, 2012).
Maternal position can greatly influence cardiac output, most dramatically during the third trimester. Cardiac output is optimized in the lateral position, somewhat decreased in the sitting position, and markedly decreased in the supine position (Tsen, 2005). In the supine position, pressure exerted on the inferior vena cava from the gravid uterus decreases venous return and results in decreased cardiac output. This position may lead to supine hypotension with diaphoresis and possible syncope.
Cardiac output rises progressively during labor (Harris, 2011; Monga, 2009). Changes in cardiac
output during the intrapartum period depend on maternal position, type of anesthesia, and method of birth. During the first stage of labor, approximately 300 to 500 mL of blood are shunted from the uterus into the systemic circulation with each contraction. This results in a progressive and cumulative rise in cardiac output during the first and second stage of labor. Epidural anesthesia causes a sympathectomy and a marked decrease in peripheral vascular resistance that may cause a decrease in venous return, resulting in decreased cardiac output. An intravenous fluid bolus before epidural placement may mitigate these effects.
output during the intrapartum period depend on maternal position, type of anesthesia, and method of birth. During the first stage of labor, approximately 300 to 500 mL of blood are shunted from the uterus into the systemic circulation with each contraction. This results in a progressive and cumulative rise in cardiac output during the first and second stage of labor. Epidural anesthesia causes a sympathectomy and a marked decrease in peripheral vascular resistance that may cause a decrease in venous return, resulting in decreased cardiac output. An intravenous fluid bolus before epidural placement may mitigate these effects.
Immediately after birth, cardiac output is 60% to 80% higher than during prelabor levels, declining rapidly after 10 to 15 minutes to stabilize at prelabor values after 1 hour (Monga, 2009). As a result of these hemodynamic changes, the intrapartum and immediate postpartum periods are times of increased vulnerability in women with cardiovascular disease. Cardiac output remains high for 24 to 48 hours after birth and then progressively decreases and returns to nonpregnant levels by 6 to 12 weeks postpartum in most women (Blackburn, 2012).
DISTRIBUTION OF BLOOD FLOW
Most of the increase in blood volume during pregnancy is distributed to the uterus, kidneys, breasts, and skin. The uterus accommodates one third of the additional blood volume at term. The kidneys receive approximately 400 mL/min. Glandular growth, distended veins, and tissue engorgement reflect the increased blood flow to the breasts, which may lead to a sensation of heat and tingling. Hyperemia of the cervix and vagina is also evident. Blood flow to the maternal skin increases to compensate for the heat loss created by fetal and placental metabolism as well as increases in maternal metabolic rate. This increased blood flow can result in alterations in nail and hair growth, increased nasal congestion, rhinitis, increased risk of nosebleeds, and sensations of warm hands and feet (Blackburn, 2012; Kumar, Hayhurst, & Robson, 2011).
BLOOD PRESSURE
Maternal position during BP measurement significantly affects BP values. Sitting or standing BP measurement shows minimal change in systolic blood pressure (SBP) throughout pregnancy. Diastolic blood pressure (DBP), measured while in sitting or standing positions, gradually decreases by approximately 10 to 15 mm Hg over the first-trimester values, with lowest values seen at 24 to 32 weeks, followed by a gradual increase toward nonpregnant baseline values by term (Monga, 2009). Accurate comparison of BP values depends on consistent techniques of measurement and consistent maternal positioning. Changes in BP are thought to be related to the vasodilatory effects of nitric oxide, PGI2, and relaxin that mediate a decrease in SVR (Monga, 2009).
SYSTEMIC VASCULAR RESISTANCE
SVR decreases by 20% to 30% (Hegewald & Crapo, 2011). Changes in SVR are related to the increased capacity of the uteroplacental blood vessels; the effects of progesterone, nitric oxide, and PGI2 on vascular smooth muscle and vasodilation; and the softening of collagen fibers (Schrier, 2010). The uteroplacental vascular system is a low-resistance network that accommodates a large percentage of maternal cardiac output. Uterine vascular resistance also decreases during pregnancy and enhances uterine blood flow. SVR decreases by 5 weeks’ gestation, is lowest at 16 to 34 weeks’ gestation, and gradually increases by term, when the mean SVR may approximate nonpregnant values (Monga, 2009).
HEMATOLOGIC CHANGES
To meet additional oxygen requirements of pregnancy, RBC volume increases approximately 20% to 30% (Monga, 2009). However, because plasma volume increases to a greater degree than the erythrocyte volume, the hematocrit decreases approximately 3% to 5%. This decrease is most obvious during the second trimester, after blood volume peaks.
Hemoglobin levels during pregnancy are between 12 and 16 g/dL. With the increase in the number of RBCs, the need for iron for the production of hemoglobin also increases. Serum ferritin levels decrease, with the greatest decline seen at 12 to 25 weeks (Kilpatrick, 2009). Approximately 500 mg of iron is needed for the increases in maternal RBCs, 270 mg by the fetus and 90 mg by the placenta. Total iron needs during pregnancy, including replacement of losses, are estimated at 1 g. Iron needs increase from 0.8 g/day in early pregnancy to 7.5 mg/day by term (Kilpatrick, 2009; Monga, 2009).
The GI absorption of iron is increased during pregnancy, but additional iron supplementation is nonetheless necessary for most women to maintain maternal iron stores. If iron stores are initially low and supplemental iron is not added to enhance the diet, iron-deficiency anemia may result (Kilpatrick, 2009). There is controversy surrounding the efficacy and benefit of prophylactic oral iron supplementation during pregnancy (Peña-Rosas & Viteri, 2009; Sanghvi, Harvey, & Wainwright, 2010). Thus, supplementation may not be needed to prevent iron-deficiency anemia in a woman who has good iron stores prior to pregnancy and a diet during pregnancy that is high in bioavailable
iron. However, many women of childbearing age have marginal iron stores (Monga, 2009). Iron supplementation does not prevent the normal fall in hemoglobin (due to hemodilution) but can prevent depletion of stores and onset of iron-deficiency anemia.
iron. However, many women of childbearing age have marginal iron stores (Monga, 2009). Iron supplementation does not prevent the normal fall in hemoglobin (due to hemodilution) but can prevent depletion of stores and onset of iron-deficiency anemia.
Leukocyte (especially neutrophil) production also increases in pregnancy. The average white blood cell (WBC) count in the third trimester is 5,000 to 12,000/mm3 (Kilpatrick, 2009). Labor and early postpartum levels may reach 20,000 to 30,000/mm3 without an infection. The increase in WBC count begins during the second month, and the level returns to the normal range for nonpregnant women by 6 days postpartum. Slight increases in eosinophil levels and slight decreases in basophil levels have also been reported (Kilpatrick, 2009). Platelet counts range between 150,000/mm3 and 400,000/mm3, with perhaps a slight decrease in the third trimester (McCrae, 2010).
Plasma proteins and other components are also altered during pregnancy. Serum electrolytes and osmolality decrease. Serum lipids, especially cholesterol (needed for steroid hormone synthesis) and phospholipids (needed for cell membranes), increase 40% to 60%. Total plasma protein decreases 10% to 14% due primarily to hemodilution, but with both absolute and relative decreases in serum albumin. This leads to decreased serum oncotic pressure that contributes to the dependent edema seen in many pregnant women. The decrease in albumin also results in less bound and increases in the free fraction of substances such as calcium and some drugs (Blackburn, 2012).
Coagulation and fibrinolytic systems undergo significant changes during pregnancy with alterations in coagulation (precoagulant) factors, coagulation inhibitors, and fibrinolysis. Pregnancy is considered a hypercoagulable state because of increased levels of many coagulation factors and a decrease in factors such as protein S that inhibit coagulation. The most marked increases occur in factors I (fibrinogen), VII, VIII, and X and von Willebrand factor (vWF) antigen (Montagnana, Franchi, Danese, Gotsch, & Guidi, 2010). These changes are partially balanced by alterations in the plasminogen system that enhances clot lysis. Prothrombin time (PT) and activated partial thromboplastin time (aPTT) decrease slightly as the pregnancy comes to term; however, bleeding time and clotting time remain unchanged despite the increase in clotting factors (Blackburn, 2012). The net effect of these alterations places pregnant women at increased risk for thrombus formation and consumptive coagulopathies. After birth, coagulation is initiated to prevent hemorrhage at the placental site. Fibrinogen and platelet counts decrease as platelet plugs and fibrin clots form to provide hemostasis.
RESPIRATORY SYSTEM
Changes in the respiratory system are essential to accommodate increased maternal-fetal requirements and to ensure adequate gas exchange to meet maternal and fetal metabolic needs. The respiratory system must provide an increased amount of oxygen and efficiently remove carbon dioxide. Changes in the respiratory system are due primarily to a combination of mechanical forces (e.g., the enlarging uterus) and biochemical effects, especially the effects of progesterone and PGs on the respiratory center and bronchial smooth muscle. Table 3-2 summarizes the changes in respiratory function during pregnancy.
STRUCTURAL CHANGES
Pressure from the uterus shifts the diaphragm upward approximately 4 cm, decreasing the length of the lungs. To adjust to this decreased length, the anteroposterior diameter of the chest enlarges by 2 cm. Increased pressure from the uterus widens the substernal angle 50%, from 68 to 103 degrees, and causes the ribs to flare out slightly (Bobrowski, 2010; Whitty & Dombrowski, 2009
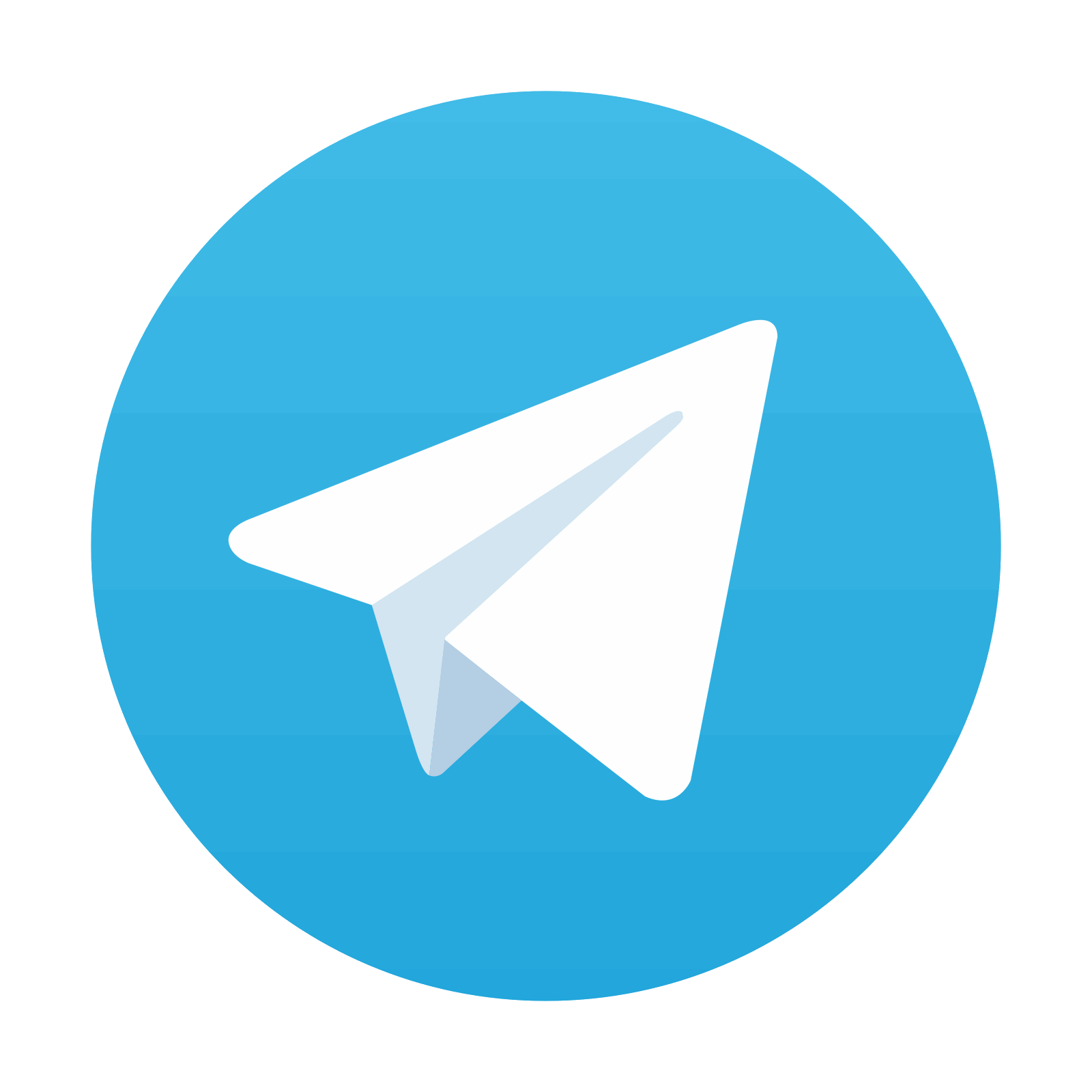
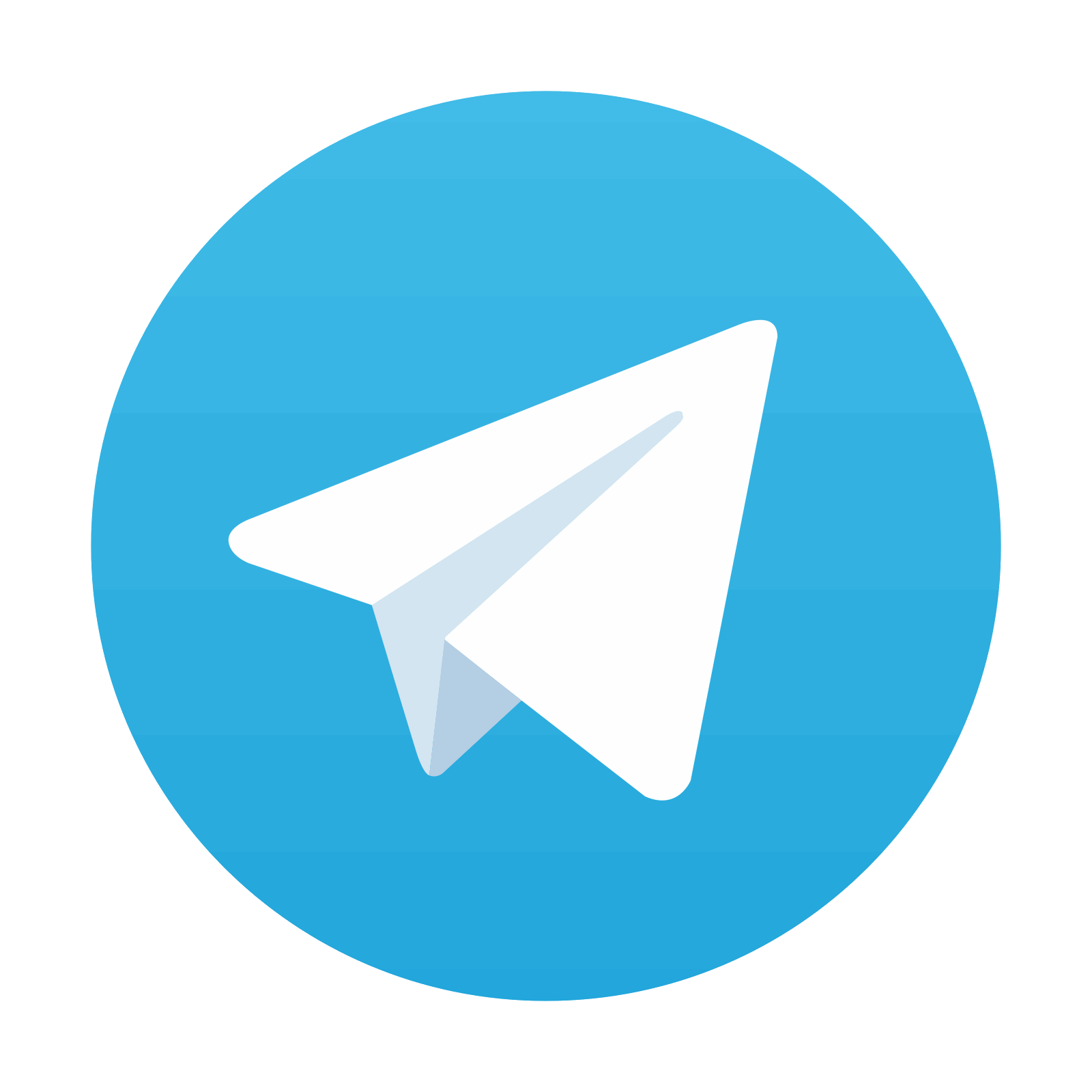
Stay updated, free articles. Join our Telegram channel

Full access? Get Clinical Tree
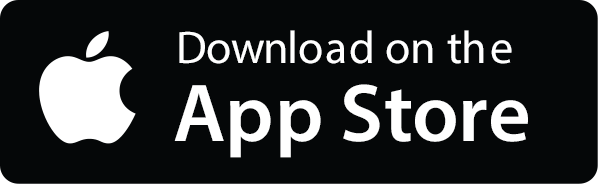
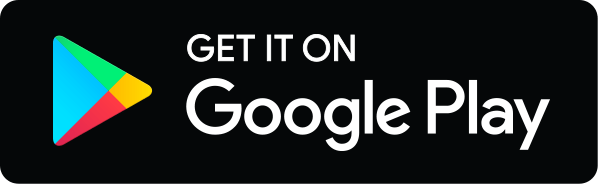
