Chapter 8 Pharmacokinetics and pharmacodynamics
INTRODUCTION
Patients rely on nursing staff and pharmacists to ensure that medicines are administered appropriately. It is essential that nurses have a sound understanding of what happens to a drug following administration. Pharmacokinetics is the term used to describe how the body handles a drug over a period of time, including how the body absorbs, distributes, metabolises and excretes the drug (Fig. 8.1). These processes influence the effectiveness of the drug because, in order for a drug to be effective, it must be available at the site of action in the correct concentration. In the simplest terms, pharmacokinetics can be described as what the body does to the drug, as opposed to pharmacodynamics, which is what drugs do to the body.
ABSORPTION
Except for the intravenous route, a drug must be absorbed across cell membranes before it enters the systemic circulation. The oral route is the one most commonly used for drug administration. Most drugs are absorbed by diffusion through the wall of the intestine into the bloodstream, which is aided by the very large surface of the gut wall. The rate of absorption also depends on the lipid-solubility of the drug. Drugs are normally formulated to make them as lipid-soluble as possible to enable them to cross the cell membranes of the intestinal wall. However, sometimes a drug’s low lipid-solubility is used to good effect, because it reaches the colon largely unabsorbed, for example aminosalicylates for use in ulcerative colitis and the antibiotics vancomycin and neomycin. In these examples, the aim is to get the drug into the lumen of the colon for therapeutic purposes while avoiding systemic absorption. A few drugs are absorbed by active transport processes. Iron, levodopa and fluorouracil are examples of drugs actively transported across the intestinal mucosa.
DISTRIBUTION
Drugs diffuse out of the plasma into tissue spaces, and some enter cells and spread through the total water of the body. The total body water represents about 0.55 L/kg. Therefore the more widely a drug diffuses, the lower will be the concentration produced by a given dose. Factors that affect the rate and extent of distribution are cardiac output and regional blood flow. If the patient is nursed in a warm environment, this will help to maintain a better blood circulation and improve drug distribution, an important factor in patients receiving antibiotics. Similarly, inflamed tissues have increased vascularity and permeability, which lead to an increased rate of passage of drugs, especially antibiotics.
TRANSFER BARRIERS
The central nervous system is surrounded by a specialised membrane consisting of the blood–brain and blood–cerebrospinal fluid barriers (Fig. 8.2). This membrane is highly selective for lipid-soluble drugs; for example, the penicillins diffuse well into body tissues and fluids but penetration into the cerebrospinal fluid is poor, except when the meninges are inflamed. Chloramphenicol, because of its lipid-solubility, is one of the few antibiotics that reach the cerebrospinal fluid in appreciable concentrations. Dopamine in the treatment of Parkinson’s disease cannot be given in this form, because it does not cross the blood–brain barrier. It is administered orally as the precursor, levodopa, which is absorbed, crosses the blood–brain barrier and is broken down to dopamine by the enzyme dopa decarboxylase.
METABOLISM AND EXCRETION
Most drugs are very lipid-soluble, and this enables them to cross cell membranes or the blood–brain barrier in order to reach their site of action. If a lipid-soluble drug is filtered by the kidney, it is largely reabsorbed from the distal tubule (Fig. 8.3) and retained in the body. Metabolism increases the water-solubility of the molecule and aids its elimination. Most metabolism takes place in the liver, but it can also be carried out in other organs, including the gut wall, lungs and kidney, and in the plasma.
RENAL DISEASE
The severity of renal impairment is expressed in terms of glomerular filtration rate, usually measured by creatinine clearance (Cr Cl). Creatinine is an end product of muscle metabolism and is eliminated from the body by the kidney. Cr Cl is obtained by measuring the plasma creatinine concentration in a 24-h collection of urine. When this is difficult to obtain, the serum Cr Cl is used. Normal Cr Cl is 100–120 mL/min for both men and women. Renal impairment is divided into three grades, as shown in Table 8.1. Renal function declines with age, and many elderly patients have a glomerular filtration rate of less than 50 mL/min.
Table 8.1 The three grades of renal impairment
Grade | Glomerular filtration rate (mL/min) |
---|---|
Mild | 20–50 |
Moderate | 10–20 |
Severe | < 10 |
The Cockcroft–Gault equation aims to predict Cr Cl from knowledge of serum creatinine, age and weight (ideal body weight, IBW):
Weight should be lean body mass. Estimate lean body mass for extremes of size.
DOSE–EFFECT RELATIONSHIP
After administration of a drug, its plasma level rises; the more rapidly the drug is absorbed, the faster its plasma level rises (Fig. 8.4).
HALF-LIFE OF DRUGS
Half-lives of different drugs vary widely; for example, the half-life of theophylline is 3 h, that of aspirin, 6 h; of metronidazole, 9 h; of digoxin, about 36 h; and of phenobarbital, about 5 days. A short half-life may result from extensive tissue uptake, rapid metabolism or rapid excretion, and a long half-life may be the consequence of extensive plasma protein binding, slow metabolism or poor excretion. The knowledge of half-lives of drugs is essential in determining the intervals between drug doses.
Certain conditions can be treated with a single dose of medication (e.g. analgesics for a headache). Many conditions, however, require continuous drug action (e.g. diabetes mellitus, infections, arthritis). This can be achieved through the administration of repeated doses at regular intervals. In such therapy, the second, third and subsequent doses will add to whatever remains of the previous dose, causing gradual accumulation until stable concentrations are maintained (Fig. 8.5).
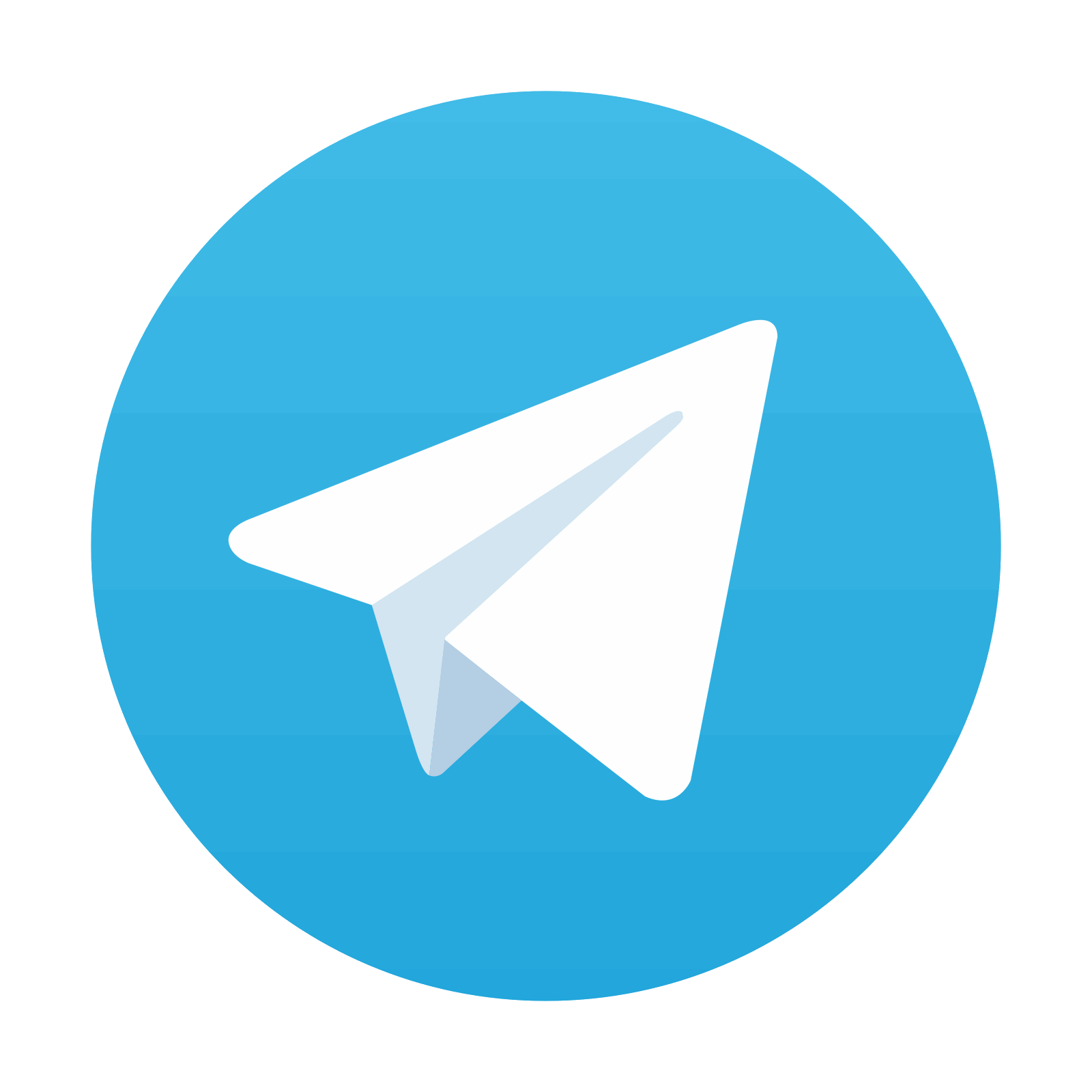
Stay updated, free articles. Join our Telegram channel

Full access? Get Clinical Tree
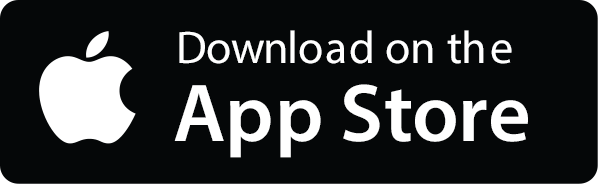
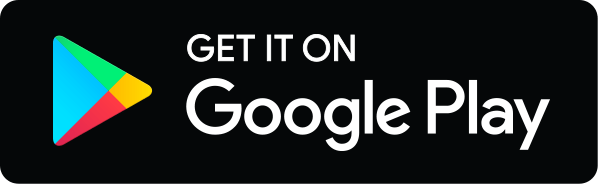