2 Specialist Practitioner in Children’s Community Nursing, Nottingham Children’s Hospital
- understand the importance of adequate oxygenation and its effects on the respiratory system in children
- learn about the methods used for and the significance of monitoring oxygen levels
- learn about the causes of low oxygen levels
- become familiar with home oxygen therapy and monitoring
- understand the importance of community nursing support.
Pathophysiology of oxygenation
The word oxygen (derived from Greek oxys acid, genes producer) is required for oxidative metabolism to produce energy for the tissues and the product of this is carbon dioxide (CO2), an acidic gas (Davies and Carl 2003). The purpose of the lung is gas exchange and its primary function is to allow oxygen from inspired air to move into and carbon dioxide to move out of the blood, as discussed in Chapter 1. Problems can lead to low oxygen levels which will be discussed next.
Hypoxaemia/hypoxia
The terms ‘hypoxia’ and ‘hypoxaemia’ are sometimes used interchangeably but they represent different concepts. Hypoxia is an inadequate amount of oxygen available at the tissue level (this cannot be measured) whereas hypoxaemia is an inadequate amount of oxygen in the blood (this can be measured). Hypoxia occurs for a variety of reasons.
Pulmonary causes
- Alveolar hypoventilation, when there is inadequate respiratory effort secondary to respiratory depression or neuromuscular disease, for example
- Diffusion defects at the alveolar-capillary level, for example in interstitial lung disease where the diseased interstitium is preventing adequate gas exchange
- Ventilation-perfusion (V/Q) mismatch is the most common cause. This occurs (1) when areas of the lung that are receiving the blood supply, known as perfusion, are not moving the air in and out, known as ventilation, and (2) there are areas of the lung that are better ventilated than perfused, due to airway obstruction
Non-pulmonary causes
- Reduced blood flow: myocardial infarction, shock and anaemia
- Non-functioning haemoglobin (the element of the blood that transports oxygen): haemoglobin can become bound to other substances, such as carbon dioxide and cyanide. In order to cope with this, the body has compensatory mechanisms to prevent and correct hypoxia. The respiratory system will increase the minute ventilation by increasing the respiratory rate and/or the tidal volume, and will change the blood flow to optimize the V/Q ratio. The heart rate and contractility will increase, and selective vasoconstriction and vasodilation will take place to pump oxygenated blood to the priority organs.
With the exception of the kidneys’ response, all the compensatory mechanisms to correct hypoxemia increase the tissue demand for oxygen, thus increasing workload, which can result in respiratory failure.
Respiratory failure
Respiratory failure exists when the respiratory system cannot transfer oxygen into or CO2 out of the blood. This can happen acutely, for example with severe respiratory syncytial virus or bronchiolitis, or more insidiously in a child with neuromuscular disease. In the latter category, an intercurrent illness may tip these children over into acute or chronic respiratory failure.
It is helpful to consider respiratory failure as arising from various situations (Box 6.1).
Oxygen therapy is required for hypoxemic respiratory failure. For the purposes of this chapter, only long-term oxygen supplementation in a non-acute setting will be considered.
Long-term oxygen therapy
Long-term oxygen therapy (LTOT) is the provision of oxygen therapy for continuous use at home for patients with chronic hypoxaemia (due to any cause), in order to maintain oxygen saturation (SpO2) at or above 92%. Measurement depends on the type of oximeter or arterial oxygen tension (PaO2) above 8 kPa. LTOT may be required 24 h a day or during periods of sleep only. Any child likely to require continuous or intermittent oxygen therapy for more than 2–3 weeks should be considered for discharge home on LTOT. The principal aims of LTOT are to prevent harm from chronic hypoxaemia and to improve any relevant symptoms (Box 6.2) (Balfour-Lynn et al. 2009).
When considering LTOT, it is useful to include the following five fundamental questions, the first two of which will be discussed next. The last three questions will be covered in the section below on home oxygen guidelines.
- Failure of control of respiration: central nervous system diseases, e.g. conditions such as congenital central hypoventilation syndrome, drugs, encephalitis
- Failure of the pump: due to diseases of the spinal cord, e.g. spinal muscular atrophy, nerves, e.g Guillain–Barré syndrome, chest wall deformities, e.g. severe kyphoscoliosis
- Lung diseases: infections, e.g. severe bronchiolitis, pneumonia, upper or lower airway obstruction, e.g. acute laryngotracheobronchitis (croup) or acute severe asthma
- Congenital lung disease: e.g. pulmonary hypoplasia, diaphragmatic hernia
- Chronic neonatal lung disease
- Other oxygen-dependent neonatal lung conditions
- Pulmonary hypertension
- Infants and children who have recurrent cyanotic-apnoeic episodes, severe enough to require cardiopulmonary resuscitation
- Interstitial lung disease, obliterative bronchiolitis
- Hypoxic children with cystic fibrosis
- Obstructive sleep apnoea
- Hypoventilation (assuming the child is optimally ventilated)
- Sickle cell disease
- Palliative care
- Why give oxygen?
- Who needs home oxygen?
- How much oxygen should be given?
- What equipment is required?
- When is it no longer necessary?
Why give oxygen?
Poets (1998) reviewed the literature to ascertain if infants required supplentary oxygen, suggesting that chronic hypoxaemia can lead to pulmonary hypertension. This is initially a reactive phenomenon and can be reversible. If chronic hypoxia persists, structural changes happen in the pulmonary vasculature leading to permanent changes and established pulmonary hypertension.
Babies with chronic neonatal lung disease (CNLD) tend to have more reactive pulmonary vasculature, and small changes in oxygenation seem to produce significant changes in pulmonary artery pressure. Also repeated episodes of hypoxia seem to have a greater effect than isolated episodes. Pulmonary hypertension can develop in any case of chronic hypoxaemia, including severe advanced cystic fibrosis, sickle lung disease or obstructive sleep apnoea. It is important to recognise and correct chronic hypoxaemia in these disorders to prevent the occurrence of pulmonary hypertension.
As previously mentioned, CNLD infants have an increased risk of this complication. Teague et al. (1998) discussed increased airway resistance in CNLD babies. When SpO2 of 89% on room air was corrected to 94–96% with supplemental oxygen, airway resistance decreased, dynamic compliance improved and work of breathing decreased. It is well documented that decreased oxygen levels have adverse effects on cognition and behaviour in babies with CNLD. For older children, growth and development will also be affected in the long term.
It may be difficult to separate out the effects of the stormy neonatal course and associated complications related to prematurity and the effects of chronic hypoxaemia on neurocognition and behaviour. Sleep-disordered breathing can lead to poor neurocognitive outcomes. It can be associated with gas exchange abnormality but there is also sleep fragmentation and both can lead to learning difficulties.
An increased risk of apparent life-threatening events in babies with CNLD occurs when target oxygen saturations are maintained at below 90%; failure to grow is another consequence of this. However, growth velocity improves and catch-up growth occurs when babies are supplemented with oxygen while the growth rate declines if oxygen is discontinued. Also, maintaining target oxygen saturation less than 90% leads to poor sleep quality in babies with CNLD, and this improves when supplemental oxygen is given.
Who needs home oxygen?
Current British Thoracic Society (BTS) guidelines make recommendations in relation to best practice for children who require supplemental oxygen (Balfour-Lynn et al. 2009). It should be remembered that the clinical conditions for which infants and children require home oxygen are very different from adults though there is an overlap between older children and young adults. The important factors that need to be considered are that the prognosis in infancy is usually good and many children only need oxygen for a limited period, although this may be for some years. As previously discussed, growth and neurodevelopment are important. Assessment of this is different at different gestational ages and consideration needs to be given particularly to the difficulty of arterial blood sampling as part of the assessment process prior to discharge home.
Monitoring oxygen therapies
The amount of oxygen in the blood can be measured in three different ways: the partial pressure of oxygen (PaO2), oxygen content (CaO2), and direct measurement of oxygen in the blood (SaO2). SpO2 involves an indirect measurement of the oxygen saturation level using a probe and pulse oximeter. SpO2 obtained from the pulse oximeter is approximately equal to SaO2 (arterial haemoglobin saturation from a blood gas).
- PaO2 is the pressure (P) exerted by oxygen dissolved in the arterial blood (a). 30% total oxygen.
- CaO2 is the number of millilitres of oxygen carried by 100 mL of whole blood.
- SaO2 is the percentage (%) of oxygen that the haemoglobin is carrying.
The greater portion of oxygen (about 97%) is chemically bound to haemoglobin as oxyhaemoglobin. The PaO2 reflects gas exchange in the lung and is the driving force behind haemoglobin saturation.
In children, PaO2 is obtained by performing an arterial blood gas measurement though this is usually only done when the child is on the intensive care unit and has an indwelling arterial line. A normal range for PaO2 on room air is 70–100 mmHg. This measurement can be affected by age. As people age, their ‘normal’ PaO2 decreases. Also, the higher the altitude, the lower the pressure to push oxygen into the blood.
Haemoglobin carries oxygen around the body. The haemoglobin molecule consists of two pairs of polypeptide chains (α and β) and each of the polypeptide chains has a haem group attached. Each haem group has one iron atom attached. Oxygen is attached to and detached from the haem groups during its transport around the body. As these haem groups ‘fill up’ with oxygen, the amount of light they absorb changes. This is why arterial blood tends to be bright red and venous blood tends to be darker. This is the basic principle used in pulse oximetry.
Pulse oximetry
Pulse oximetry measures the oxygen saturation of the arterial blood flow through the extremity on which the probe has been placed (Figure 6.1). A pulse oximeter will display oxygen saturation (SpO2) and heart rate, with a plethysmographic waveform (Chandler 2000) showing the pulsatile nature of the arterial blood flow through the extremity. A pulse oximeter also displays a motion indicator showing the signal quality and whether the displayed saturation and heart rates can be considered accurate.
The basic principle of pulse oximetry is that a light is passed through the arteriolar bed (feet in small babies or an ear lobe) and measured on the other side. Therefore it is important that the light source and detector are opposite each other.
Correct positioning of the probe is very important in obtaining an accurate measure of the oxygen saturation. An appropriate probe for the size of the patient should be placed in an appropriate position (Table 6.1). It can run on battery or mains power and will have audible alarms which can be set with both an upper and a lower limit.
Red and infrared light are transmitted alternately from the light source. The absorption of light by haemoglobin varies with the level of oxygenation. Pulse oximetry requires a pulsatile flow between the light source and photodetector. There is a relationship between SpO2 and PaO2 (Table 6.2) and it is important to understand this, especially when trying to understand the lower normal limits during emergency situations.
The benefits of SaO2 monitoring are that it is non-invasive, it is accurate with a SaO2 greater than 70% and can allow continuous monitoring. However, it has limitations. Inaccurate readings will be obtained in many situations; for example, excessive movement, known as motion artefact, will limit accuracy of monitoring. With conditions such as hypotension and shock, monitoring is affected by a poor pulsatile signal.
Balfour-Lynn et al. (2009) highlight the associated problems with monitoring, such as malpositioning of the probe which may let some of the light straight through to the photodetector and give falsely high readings. Outside light interference and nail varnish in dark colours can affect the readings through the nail bed. High bilirubin levels, such as in liver failure, also affect accurate monitoring.
There are many different systems in place for monitoring infants at home on oxygen. The controversy around the use of oxygen saturation monitoring at home is not a new phenomenon. For many years there has been widespread debate as to whether oxygen-dependent infants should go home with pulse oximeters in order for parents to monitor their child’s oxygen saturation levels.
The provision of home saturation monitors should not be recommended as routine because there is no evidence of benefit or harm for their use. Some problems related to their use are increased anxiety and undue parental reliance on the monitor, with some parents finding it difficult to accept the need to discontinue their use, when oxygen therapy is weaned. Other problems include unnecessary minor adjustments of flow rates, false reassurance of respiratory status, false alarms (less with newer motion-resistant technology) and cost. Nevertheless, some clinicians and parents may find this provision helpful in certain circumstances.
Figure 6.1 Oxygen saturation monitor. Reproduced with permission from Nottingham University Hospital.
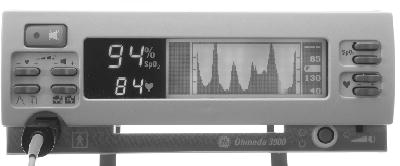
Table 6.1 Pulse oximeter position/type of probes
Age | Site | Type of probe |
Neonate/infant | Big toe, foot outer area | Adhesive single-patient use, size varies according to weight |
Child | Across hand/finger Big toe | Adhesive single-patient use |
Adolescents | Finger index | Clip reusable |
Table 6.2 SpO2 and PaO2 levels
Home oxygen guidelines
In June 2003 the Department of Health announced plans to modernise the domiciliary oxygen service. The aim was to improve patient access to a wider range of modern technologies and to reduce the number of patients who receive oxygen therapy inappropriately (Godfrey 2004).
The home oxygen database and service commenced in October 2005 following new written guidelines issued by the Department of Health (2004) which have been updated in 2013. The new service ensures that oxygen is available on prescription as part of an integrated service (i.e. cylinder, concentrator and ambulatory oxygen) delivered directly to patients in their own homes. Oxygen equipment and servicing are all supplied and carried out by the oxygen provider.
The process involves obtaining consent from parents for their child’s information to be stored on the database. Without a signed Home Oxygen Consent Form, the oxygen service is unable to supply the patient with the necessary equipment to administer supplementary oxygen. Once the consent form has been signed, a Home Oxygen Order Form (HOOF), updated in 2013, can be completed by any healthcare professional and faxed (not emailed) to the oxygen service.
Oxygen equipment can be delivered in three ways by the supplier. Emergency installation is within 4 h from acknowledgement of the faxed HOOF, next-day installation is available for hospital discharges and all other installations are standard delivery, which is within 3 working days. The cost of this service is funded by the patient’s primary care trust. A nurse advisor from the oxygen company is available to assist with providing an individualised plan of care when initiating oxygen therapy.
How much oxygen should be given?
Balfour-Lynn et al. (2009) suggest that targets should be maintained for children’s oxygen requirement which would be based on individual clinical symptoms.
In CNLD, oxygen therapy should be given to maintain a SpO2 of 93% and above. The American Thoracic Society (ATS) guidelines recommend maintaining a saturation of 95% or more once past the age of oxygen-induced retinopathy (33 weeks postmenstrual or 4 weeks chronological age), as this provides a ‘buffer’ zone against oxygen desaturation that targets of 90% or more do not (ATS 2003). In other conditions, for example sickle cell disease, maintaining SpO2 at 94–96% (to prevent painful crises and stroke) may be appropriate and for cystic fibrosis ≥ 90%. At present there are no data to guide target levels for SpO2 in children with other respiratory conditions.
Generally practice is guided by studies which indicate that less than 5% of total study time should be spent below the saturation of 90%. It is not necessary to regularly assess CO2 levels in infants with CNLD who are at home, but it may be useful in some neonates with other conditions and older children, especially when initiating home oxygen therapy. This would be carried out in hospital.
It is also good practice in infants with CNLD, prior to discharge, to perform an ECG or echocardiogram. This is useful to assess the right heart in order to exclude significant pulmonary hypertension, as previously discussed.
What equipment is required?
The home oxygen process will be discussed below. However, prescription requirements (examples in Table 6.3) may include oxygen concentrators that should be provided for LTOT, unless it is likely that the child will only require low-flow oxygen for a short while. Initially it is important to discuss and consider parent choice. Light-weight cylinders are easier to handle but they empty more quickly. Cylinders should be available for all children as part of the provision of home oxygen unless oxygen is only required at night.
When is it no longer necessary?
Discontinuing supplemental oxygen abruptly or gradually or weaning early or late does not seem to matter as long as weaning is done while maintaining the target oxygen saturations at the same level as when starting oxygen (Askie and Henderson-Smart 2001a,b).
Weaning of supplemental oxygen
This should be guided by overnight oxygen studies while maintaining the target oxygen saturations as previously discussed. Once the oxygen requirement is down to 0.1 L/min, the child may be ready to stop having supplemental oxygen.
Children can be weaned from continuous low-flow oxygen to night time and naps only, or remain in continuous oxygen throughout the 24 h until the child has no requirement at all. Oxygen equipment should be left in the home for at least 3 months after the child has stopped using it. If this is in a winter period, it is usually left until the end of winter. In CNLD, failure to reduce oxygen supplementation after 1 year warrants specialist review to rule out other conditions (see Chapter 11).
Discharge planning
Once it is deemed that the infant/child is ready for discharge and the need for oxygen is the only thing keeping the child in hospital, suitability for home oxygen therapy should be assessed. This should be carried out by a specialist with appropriate experience in the care of the relevant condition. This is usually either a respiratory paediatrician or a neonatologist (but may be a paediatric cardiologist, general paediatrician or palliative care specialist).
The family must also be assessed for their ability to manage home oxygen therapy and cope with all aspects of the child’s care. The discharge planning should put in place a multidisciplinary follow-up to ensure a safe and smooth transition into the community. Parental education should include recognition of worsening clinical status and basic life support.
Before discharge, oxygen saturations must be measured continuously for at least 6–12 h to include periods of sleep, wakefulness and activity such as feeding; one-off measurements are insufficient. Feeding is quite a vigorous exertion for the infant and it is important to include a period of feeding as infants have an increased oxygen requirement during activity and infants with CNLD may develop feeding-related hypoxaemia. In addition, some children may only show evidence of nocturnal hypoxaemia without daytime hypoxaemia.
A period of sleep should also be included as clinically unsuspected sleep-related desaturations can occur in babies who are ‘fully saturated’ awake in air (Garg et al. 1998). Children can be discharged from the neonatal unit when their oxygen requirement is stable, with mean SpO2 of ≥ 93% and without frequent episodes of desaturation. The SpO2 should not fall below 90% for more than 5% of the artefact-free recording period. There should be no other clinical conditions precluding discharge and the child must be medically stable.
Follow-up after discharge
The community children’s nurse or nurse specialist should visit the child within 24 h of discharge. In particular, infants such as those with CNLD should have their SpO2 monitored within a week of discharge, with subsequent recordings as clinically indicated (but not usually less often than 3–4 weekly); monitoring should include various activity states. Older children with other conditions who are clinically stable are likely to need home SpO2 recordings performed less often than infants with CNLD.
Older children
For older children with other conditions previously mentioned, overnight oxygen studies inform the process of assessment and are useful in determining how well children will manage with the prescribed oxygen therapy at home. Circumstances will determine this; for example, children requiring oxygen for palliative care will have different physical demands that may be very short term. Children with uncorrected cardiac problems will also have needs that differ. For those with long-term conditions such as cystic fibrosis, oxygen may only be required at night. Therefore the nature of the assessment is very much individualised and disease specific.
Oxygen outside the home
Details surrounding the use of oxygen therapy in various situations outside the home environment will be discussed below. However, an appropriately trained individual should be present whilst the child is using the oxygen, but this does not necessarily have to be a school nurse or health professional. Other considerations include holidays where consideration needs to be given to high altitude, because during airline travel when rising there is a decreased inspired oxygen concentration. In a patient with marginal pulmonary reserves or who is maintained on supplemental oxygen, higher oxygen flows will be required which should be determined by a fitness-to-fly test.
Table 6.3 Equipment for home oxygen administration
Equipment | Use | Environment |
Concentrator | LTOT | Home, school |
Light-weight cylinder, low-flow regulator for some infants | Short term/LTOT | Outside home |
Liquid oxygen | LTOT (> 0.5 L/min only in most areas) | Outside home |
LTOT, long-term oxygen therapy.
Potential disadvantages of home oxygen
It is rare for a child to be retained in hospital because the home environment is not conducive to carrying out the treatment. However, parent/carer smoking must be strongly discouraged. Parents/carers (and older children) must be made aware of the potential hazards of home oxygen, which will be addressed below. Equally, it is critical that parents and carers receive sufficient emotional support from their family, friends and healthcare services, including professionals such as community children’s nurses.
Long-term oxygen therapy
When planning for discharge home, specific equipment is required to allow for low oxygen flow rates that some infants require. Almost all children receiving LTOT require ambulatory oxygen therapy, for example portable cylinders to use outside the home (see Table 6.3). Many children require LTOT overnight only, especially during the weaning stages. This is less than the 15 h that forms part of the adult LTOT definition. Ultimately all children will require supervision from a parent/carer with all this equipment. Another consideration is that provision of oxygen may be necessary at school for school-age children.
Equipment required
Parents have a number of oxygen delivery systems to become familiar with in order to care for their child safely (Allen and Kotecha 2002).
Oxygen concentrators are the most commonly used equipment for LTOT in the home environment (Figure 6.2). Concentrators work by drawing in room air through a series of filters to remove particulate matter (including bacteria) and nitrogen with the resultant oxygen stored in a reservoir before use (Dunbar and Kotecha 2000; McLauchlan 2002).
Two outlets are normally provided, one for the main living area and one for the bedroom (Balfour-Lynn et al. 2005). From these outlets, generous amounts of tubing are supplied to aid mobility to other rooms and if possible to the garden. The oxygen supplier maintains the concentrator as part of their contract. In the interim parents are taught how to change a simple external filter once weekly.
The concentrator runs off electricity and every quarter the cost of this is reimbursed to the family by the oxygen supplier (Barnett 2006). A large oxygen cylinder is supplied to parents as a back-up in case of machine or power failure.
Oxygen conservers are not indicated for young children but can be considered for older children capable of triggering the device. Humidification should be considered for high oxygen flows, when given by facemask. This is helpful especially for children with cystic fibrosis; a cold water bubble-through humidifier may be adequate for this purpose.
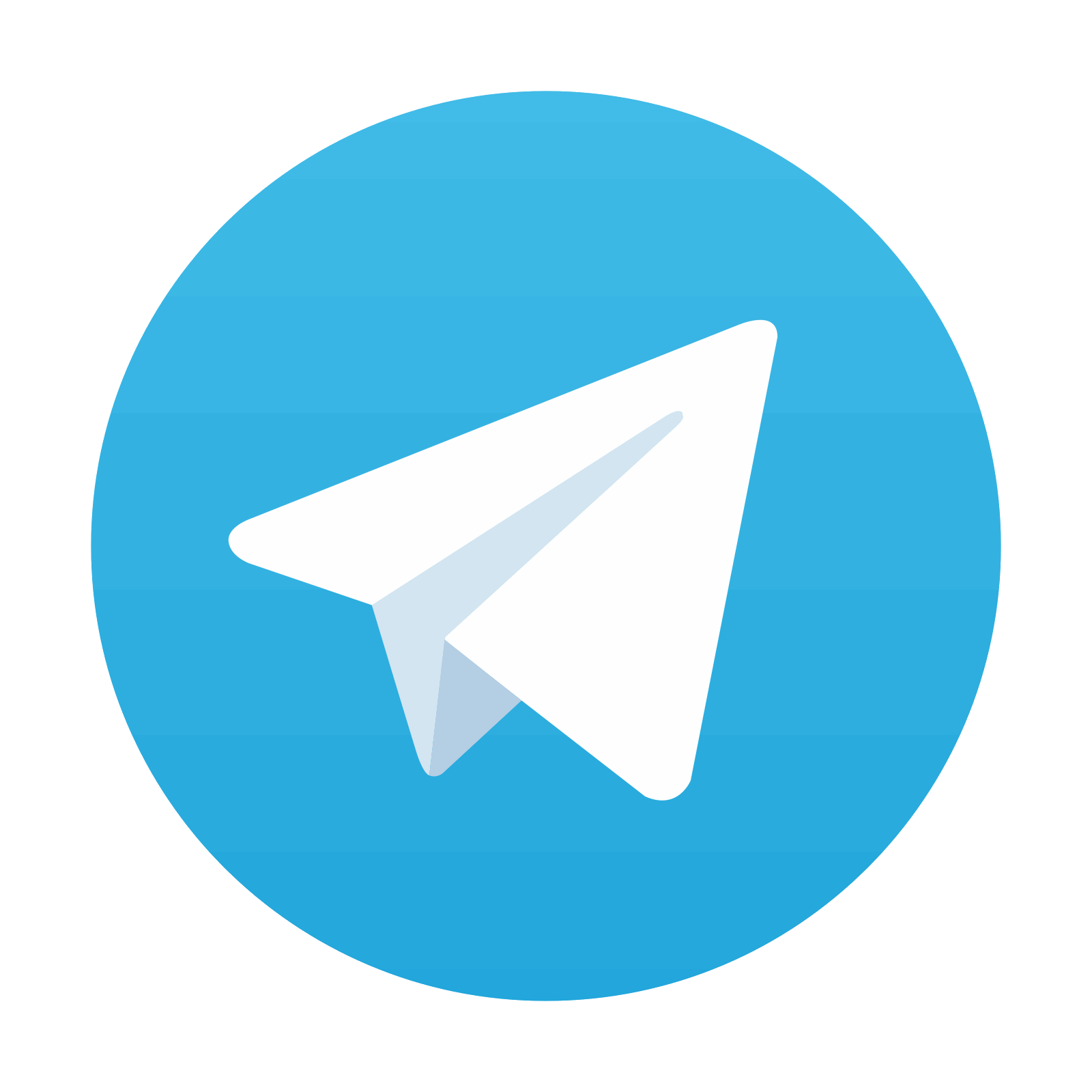
Stay updated, free articles. Join our Telegram channel

Full access? Get Clinical Tree
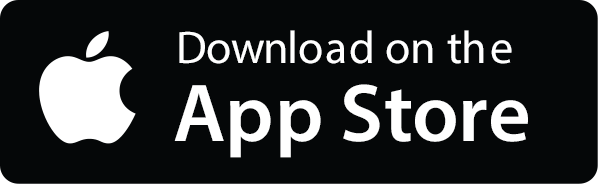
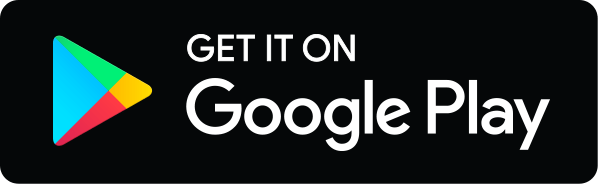