2
Overview: Genetics/Genomics
An important part of the genomic risk assessment is having a basic knowledge and understanding of genetics, genomics, and patterns of inheritance. It is well known that genomics impacts all aspects of health and illness across the life span. It is important to know the basic elements of an individual’s deoxyribonucleic acid (DNA) and how variations or alterations in the structure of one’s DNA can impact health and lead to a variety of diseases, whether due to inherited or complex/multifactorial issues including the combination of genetics and environmental factors. Understanding how variation in genes, like that of a mutation, impacts disease is important in understanding inherited disorders like sickle cell disease, hereditary breast and colon cancer syndromes, and numerous other genetic disorders.
Objectives
1. Discuss common terms related to genetics
2. Differentiate between autosomes and sex chromosomes
3. Discuss gene sequencing and its significance in health and illness
Most individuals are born with a set of 46 normal chromosomes, obtaining 23 each from their mother and father during conception (Figure 2.1). The chromosomes are often depicted in alignment as pairs (one received from the mother, the other from the father) from largest to smallest, with the first 22 pairs referred to as autosomes; these are the same for both male and female. The last pair contains chromosomes that determine one’s gender and are considered the sex chromosomes; if an individual has two X chromosomes, this indicates female gender; in contrast, one X and one Y chromosome indicate male gender. This alignment is depicted in a karyotype (Figure 2.1). The number, size, and structure of the chromosomes are important because changes in any of these qualities may result in potential problems as these chromosomes contain important genetic information. For example, individuals who have an extra chromosome 21 (e.g., 47 chromosomes), specifically three chromosomes instead of two, will have a condition known as trisomy 21 or Down syndrome.
10
Figure 2.1 Forty-six (46) human chromosomes containing 22 pairs of autosomes and one pair of sex chromosomes; this image denotes a male.
Source: U.S. National Library of Medicine.
The 46 chromosomes are tightly coiled by protein substances called histones to form nucleosomes. These DNA-packaged structures are the basis of each person’s hereditary information, the material of which is 11located in the nucleus of every cell of the body (Figure 2.2) with the exception of red blood cells (RBCs). Reproductive cells or gametes (e.g., egg and sperm), however, only have 23 chromosomes (not paired) for conception and normal human development.
In simplest terms, the DNA molecule contains four important chemical bases known as adenine, guanine, cytosine, and thiamine, often abbreviated or represented as A, G, C, and T, respectively, that bind together like rungs of a ladder. The two sides of the ladder are composed of alternating sugar and phosphate units, and each such unit has a nucleotide base attached to it. Hydrogen bonding between the bases holds the two strands together, forming the rungs of the ladder (Figure 2.3). One of the bases is larger, a purine, and the other is smaller, a pyrimidine. The purines are adenine and guanine (A and G), and the pyrimidines are cytosine and thymine (C and T). The pyrimidine thymine (T) always pairs with the purine adenine (A) and the pyrimidine cytosine (C) always pairs with the purine guanine (G). This consistent pairing is essential when the DNA replicates itself during cell division and when decoding takes place during transcription and translation to form proteins. In the human body, the DNA consists of approximately 3 billion pairs of A, G, C, and T bases. Alignment of these bases on the chromosome is known as a gene—the basic physical and functional unit of heredity. Specifically, the sequence of the A, T, C, and G makes up a gene that can vary from a few hundred to 2 million base pairs; this sequence of base pairs is approximately 99% the same for all people. The human body has approximately 20,000 to 25,200 genes.
FIGURE 2.2 Structure of DNA showing a chromosome, nucleosome, histone, gene, and nucleotide base pairs: guanine, cytosine, adenine, and thymine. Also shown are a cell and its nucleus.
Source: © 2015 Terese Winslow, LLC, U.S. Govt. has certain rights. Used with permission.
12
FIGURE 2.3 Example of a sequence of bases in a portion of a DNA molecule. This forms a gene that carries the instructions needed to assemble a protein.
Source: www.genome.gov/glossary/index.cfm?id=1. Courtesy of the National Human Genome Research Institute; Darryl Leja.
Genetic sequencing of the base pairs (A, T, C, and G) is important because it forms encoded proteins that are the blueprint instructions for the structure and functioning of the human body. The genetic code obtained through the sequence of the A, T, C, and G goes through an intricate process of transcription—DNA copied into a single strand of messenger ribonucleic acid (mRNA; Clancy & Brown, 2008a). In the transcription process, base pairing of A with T is replaced by A pairing with U—uracil 13(U) replacing thymine (T) (Figure 2.4). The base pair sequence (A, U, C, and G) of the mRNA molecule now forms a new three-base sequence that corresponds to a specific amino acid—this process is known as translation (Clancy & Brown, 2008b). The cell reads the sequence of the three bases, known as codons, that relates to specific amino acids or as a stop signal for further protein synthesis (Figure 2.5). There are 64 different codons, with 61 specifying amino acids. The remaining three are used as stop signals, with some trinucleotide sequence codons producing the same amino acids (Berg, Tymoczko, & Stryer, 2002). The codons are integral to producing the 21 amino acids—the building blocks of proteins that are essential for the body’s structure and function and the overall process of life (Lutvo, 2009). See Figures 2.3, 2.4, and 2.5 for the depiction of transcription, translation, and codons, and Table 2.1 for the genetic codon.
FIGURE 2.4 Transcription is the process of making a ribonucleic acid (RNA) copy of a gene sequence denoted in the RNA transcript, as depicted in the image. This copy, called a messenger RNA (mRNA) molecule, leaves the cell nucleus and enters the cytoplasm, where it directs the synthesis of the protein, which it encodes.
Source: www.genome.gov/glossary/index.cfm?id=197. Courtesy of the National Human Genome Research Institute; Darryl Leja.
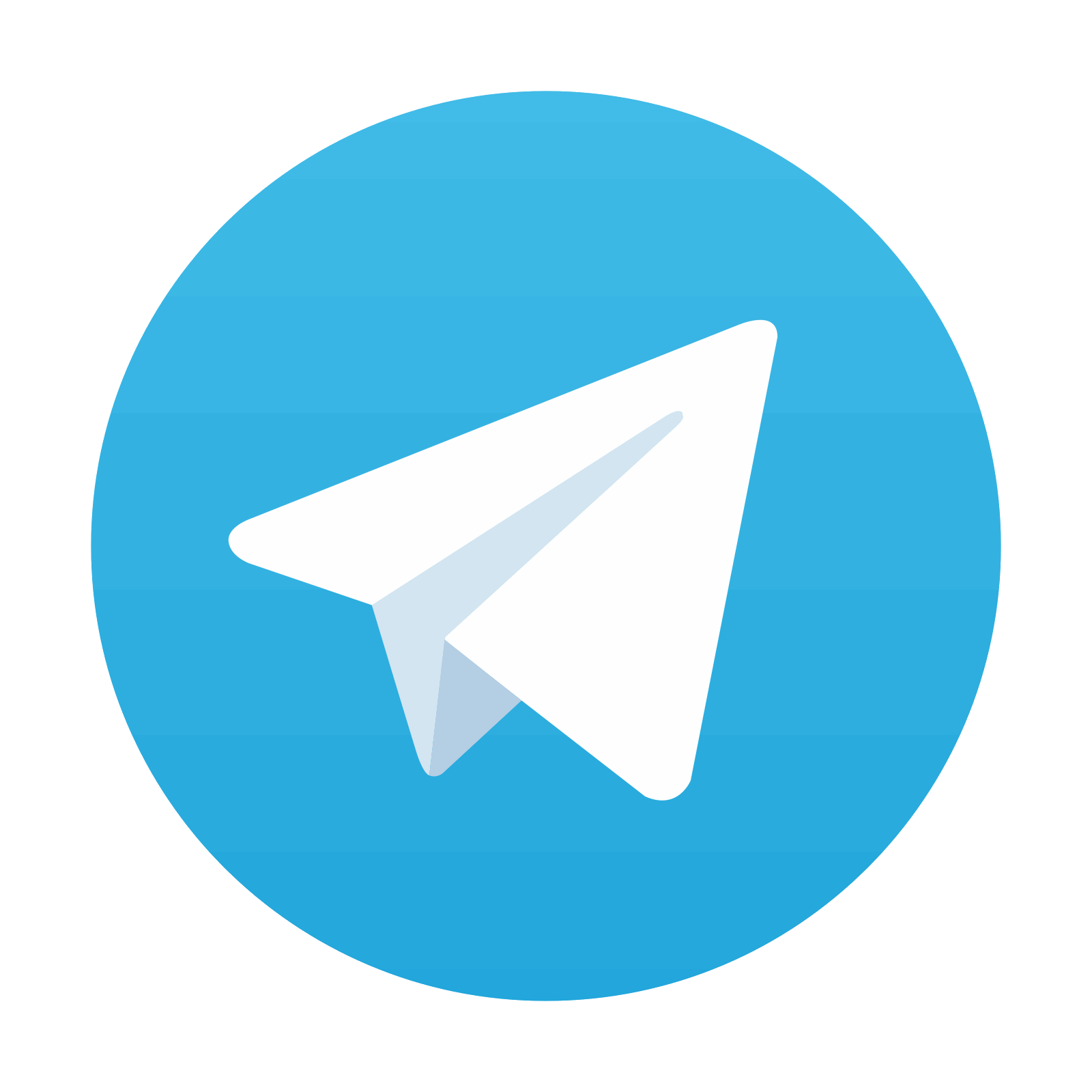
Stay updated, free articles. Join our Telegram channel

Full access? Get Clinical Tree
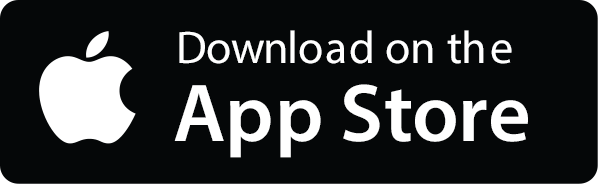
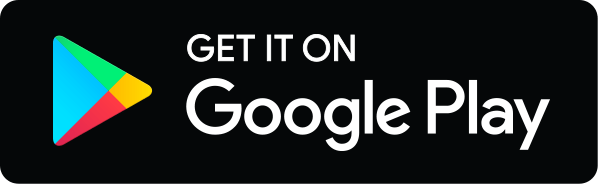