What
Goal
First line imaging
Special considerations
Brain tumor
Diagnosis and treatment/therapy/planning/posttreatment follow-up
MRI brain with and without contrast
Strongly consider obtaining spine imaging to rule out any metastatic disease
Spine tumor
Diagnosis and treatment/therapy/planning/posttreatment follow-up
MRI spine with and without contrast
Strongly consider obtaining brain imaging
Hemorrhage
Diagnosis and treatment
CT head without contrast
Consider 3D reconstruction to evaluate for skull fracture
Ventriculomegaly/hydrocephalus
Diagnosis and treatment/follow-up
Acute – CT head without contrast
CISS/CINE to evaluate anatomy that might favor an ETV
Non acute – MRI brain without contrast
HASTE MRI – follow-up imaging
Chiari malformation
Diagnosis and treatment/follow-up
MRI brain without contrast
CISS sequence to assess CSF flow around cervicomedullary junction
Syrinx
Diagnosis and treatment/follow-up
MRI spine without contrast
Vascular malformation
Diagnosis and treatment
CT and CTA or MRI and MRA
Craniosynostosis
Confirm diagnosis (initial diagnosis usually made on physical examination)
CT head without contrast
Abscess
Diagnosis/treatment/follow-up
MRI brain or spine with and without contrast
Non-accidental trauma
Diagnosis and management
CT head without contrast
Spine trauma
Diagnosis and management
CT spine without contrast
Patients and their families who are undergoing imaging will need an explanation of the procedure, as well as what to expect before, during, and after. There may be a great amount of anxiety of the unknown from these patients and families, as the purpose is often to obtain a diagnosis. Although some explanation of the procedure will be given by the ordering provider, there will be a need for proper education from nurses with knowledge of the tests, as well as emotional support before and after the imaging. As more and more imaging is being completed on an outpatient basis, it can be challenging to provide the necessary education to families. If education cannot be provided in person, it should be done through a telephone call or by written material.
18.3 Radiation
Medical imaging has become a primary tool in diagnosis of injury and illness in children. However, it is important to remember that the decision to obtain imaging tests needs to be based on the potential benefits versus the potential risks. Among those risks is exposure to ionizing radiation. Common neuroimaging techniques that use radiation include x-ray (plain films), angiogram, radionuclide CSF shuntogram, proton emission tomography (PET) scan, and the CT scan. MRI does not use ionizing radiation but generally involves longer imaging times, requiring greater patient cooperation that can necessitate sedation. CT has the advantage of speed and greater sensitivity in evaluation of some pathologies, particularly in trauma.
We are all exposed to small amounts of radiation on a daily basis. There are risks involved with radiation exposure, the greatest concern being the risk of developing cancer. The low levels of radiation patients are exposed to during medical tests can be measured in units called millisieverts (mSv). There have been no published studies that show a link between radiation exposure of less than 10 mSv and cancer. However, there have been studies done on atomic bomb survivors, as well as workers who are exposed to radiation, which suggest that radiation exposure levels of 10–100 mSv can increase the risk of developing cancer (Miglioretti et al. 2013; Pearce et al. 2012). For comparison, a child who receives multiple CT scans during their lifespan can easily exceed 10 mSv. Modern CT scanners have substantially reduced the dose required for imaging.
The Pediatric Emergency Care Applied Research Network (PECARN) published a study that evaluated over 42,000 children cared for in 25 different emergency rooms, who had experienced head trauma within the previous 24 h and presented with a Glasgow Coma Scale of 14–15. The primary goal of this study was to identify children who had a minimal risk of developing a clinically important traumatic brain injury (ciTBI) after a head injury and, therefore, decrease the number of unnecessary CT scans that are performed on these children. For the purpose of the study, a ciTBI was defined as death from a TBI, need for a neurosurgical intervention following a TBI, intubation for more than 24 h following a TBI, or hospital admission for two or more nights associated with evidence of TBI on CT scan. From this very large study, an algorithm was developed to help guide whether or not a child with a traumatic head injury should undergo a head CT. The PECARN algorithm has guidelines for children younger than 2 years of age and for those 2 years and older. Children who are younger than 2 years should undergo a CT if they have a GCS of less than 14, altered mental status, or a palpable skull fracture. A CT should be considered in children younger than 2 years who have an occipital, parietal, or temporal hematoma, loss of consciousness (LOC) for greater than 5 s, a severe mechanism of injury, or an abnormal behavior reported by parents. In children 2 years and older, a head CT should be obtained for children with a GCS of less than 14, altered mental status, or signs of a basilar fracture. A head CT should be considered in this population if there has been vomiting, LOC, and severe mechanism of injury or the child self-reports a severe headache (Kuppermann et al. 2009). If this algorithm is applied, it can result in a reduction in the number of CT scans being done on children with minor head injuries.
Nurses should have a basic knowledge of the risks involved with radiation exposure, since the increasing media attention on the subject will likely result in questions and concerns from parents. Nurses must know how to educate patients and families about those risks while also explaining the necessity of the imaging. Nurses should be vigilant about reviewing medical records to ensure that patients are not receiving duplicate images that would expose them to additional radiation. It is important to consider that children are more sensitive to radiation than adults. It is essential that the “as low as reasonably achievable” (ALARA) principle be used in pediatric patients whenever safely possible. The goal of the ALARA principle is to achieve the best imaging using the least amount of radiation by utilizing weight-based protocols, improved shielding, consideration of alternative imaging methods (including MRI), use of limited view and reduced dose images, and avoidance of repeated images (Strauss and Kaste 2006).
The simplest way for us to think of medical radiation exposure is to compare it to the radiation we are exposed to on a day-to-day basis. Some examples of different levels of radiation exposure are listed on Table 18.2.
Table 18.2
Radiation exposure in mSv
Exposure source | Level of radiation |
---|---|
Natural radiation per year | 3.0 mSv |
Cross country flight | 0.04 mSv |
Single chest x-ray | 0.01 mSv |
Spine x-ray | 1.5 mSv |
CT scan – head | 2–4 mSv |
Low-dose CT scan – head | 0.5–1.0 mSv |
CT scan – spine | 6–10 mSv |
18.4 Contrast
Dense parts of the body such as bone are easily imaged using an x-ray or CT, but soft tissue structures (including tumors) are often difficult to see. Intravenous contrast agents can assist with visualization of these structures and improve the accuracy of diagnosis prior to any surgical intervention. For example, contrast can help evaluate different types, sizes, and locations of brain or spine tumors for diagnosis and surgical planning. Contrast can also help characterize infections and vascular anomalies. After diagnosis and treatment of an infection, it can be used to determine response to the therapy and monitoring over the long term. It can also be used to follow vascular anomalies such as arteriovenous malformations (AVMs) or cavernous malformations over time and also to detect new anomalies before symptoms occur.
Iodinated (iodine-containing) contrast is most commonly used with CT. It can greatly enhance the clarity of vascular structures and provide improved visibility of tumors or abscesses. Gadolinium-based contrast has magnetic properties that are used to enhance brain and spine images created by MRI.
Prior to the administration of any contrast agent, nurses should consider the degree of renal function in children with known renal disease and whether they are receiving nephrotoxic medications like chemotherapy or some antibiotics. Use of iodinated contrast needs to be carefully considered for children who are dehydrated or who have impaired kidney function. Gadolinium contrast should be avoided in the child with significantly impaired kidney function.
In very rare cases, children can have an allergic reaction to both iodinated and gadolinium-based contrast, although allergic reactions, especially anaphylaxis, are much less common with gadolinium than to iodinated contrast. The current literature shows that there is no relationship between a shellfish or iodine allergy and an allergy to contrast agents (Schabelman and Witting 2010). Patients should be asked if they have any allergies or have had a previous reaction to contrast (Schabelman and Witting 2010). Patients who have had a reaction to contrast in the past, such as hives, pruritus, or shortness of breath, should be premedicated if contrast use cannot be avoided (e.g., steroids, antihistamine). When a patient has had a previous anaphylactic reaction, such as airway edema or difficulty breathing, alternative imaging should be considered.
18.5 Sedation
One of the most important things about obtaining quality images in pediatric patients is ensuring that they stay still during the procedure. Every consideration should be made to avoid the use of sedation, as there are risks involved, most commonly respiratory depression. Additionally, there is some concern for the possible effects of sedation on the developing brain. There have been many studies done on rodents and primates demonstrating that there can be an effect on neuronal development when anesthesia is administered during the critical period of brain growth (Reddy 2012).
There is no consensus on how these animal studies translate to humans, and specifically children, undergoing anesthesia. A study of 2,608 children in Australia, of whom 321 had been exposed to anesthesia before age 3, found that children in the exposed group had an increased long-term risk of deficits in language and abstract reasoning at age 10 compared to unexposed children (Ing et al. 2012). In contrast, the Pediatric Anesthesia and Neurodevelopment Assessment Study (PANDA) was recently published and showed no evidence of any link between a single exposure to anesthesia in children below the age 3 and cognitive delays (Sun et al. 2016). Similarly, an international multicenter study also revealed no connection between a short period of general anesthesia (under 1 h) in children under two and neurocognitive delays (Davidson et al. 2016). The level of concern with the use of anesthesia in children is continuously evolving.
The American Academy of Pediatrics (AAP) defines the goals of sedation in the pediatric patient for diagnostic procedures as follows: to ensure the patient’s safety, to reduce physical discomfort and pain, to keep anxiety to a minimum, to limit movement to allow safe completion of the procedure, and to return the patient to their baseline prior to discharge (Charles and Stephen 2007; Coté and Wilson 2016). When a child must be sedated for imaging, it is preferable that it be done under the care of a trained pediatric anesthesiologist (Coté and Wilson 2016).
In most cases, sedation is not needed for a CT scan. Younger children can be safely restrained for the duration of the imaging, and older children are able to cooperate and hold still for the time needed to obtain a CT scan of the head or spine.
Sedation is usually required for an MRI in children younger than 6–8 years of age, with the exception of a half-Fourier acquisition single-shot turbo spin echo (HASTE), also known as a rapid sequence MRI. An MRI can cause a great amount of fear in children, as the machines are very loud and it requires laying still and alone in a small space (Arlachov and Ganatra 2012). Children are, however, less prone to claustrophobia which can be a problem with adults. Most children 8 years and older can be distracted with the use of a movie or music. Neonates can usually be scanned using a fast and feed protocol, where the infant is fed immediately prior to the study and will usually sleep for the duration of the scan. At around 3 months of age, sedation is usually necessary to obtain adequate imaging. With patience on the part of the nursing staff and cooperation from the technicians, nonsedated imaging can be achieved in many cases. Centers around the world are looking at alternative options to sedation, including sleep deprivation, hypnosis, distraction, melatonin, play therapy, and parental involvement (Arlachov and Ganatra 2012). Allowing a family member to be present during the exam can often be a great calming mechanism for children.
Limited imaging techniques that would be performed in a rapid manner are currently under development. These will have various clinical applications such as evaluation for only the presence of hydrocephalus.
18.5.1 Nursing Care
Baseline assessment prior to sedation: respiratory rate, oxygen saturations, blood pressure, pulse, and neurological exam.
Is sedation needed?
Has patient adhered to fasting guidelines? (These instructions should be given to family several days before the procedure if possible.)
Prepare the patient and family, including an explanation of the possible complications.
Has informed consent been obtained from the patient’s parent or legal guardian for both the sedation and the procedure being performed?
Monitor throughout the procedure and during the recovery period, including respiratory rate, oxygen saturation, blood pressure, and pulse and that the child returns to their pre-sedation neurological baseline.
Educate patient and family about post-sedation side effects, including nausea, vomiting, sleepiness, confusion, chills, and throat pain if the patient needed intubation for general anesthesia. If patient is being discharged after sedation, instructions should be given including phone numbers to call if the family has questions or concerns.
18.6 Neuroimaging Techniques
18.6.1 Computed Tomography (CT) (Figs. 18.1 and 18.2)

Fig. 18.1.
Normal head CT

Fig. 18.2
Normal cervical spine CT
18.6.1.1 How Does It Work/Procedure
A plain film x-ray will depict a skull fracture but is of little value for imaging the brain. Tomography refers to imaging by sections. CT works in a similar way to an x-ray, but with a CT scan, multiple images are taken from multiple angles as the x-ray tube rotates around the patient’s body. A computer then reconstructs the images into cross-sectional images or “slices.” These slices can either be viewed individually or stacked on top of each other to create a 3D image of the brain or spine. The provider then has the ability to rotate and view the images to find the exact location of the abnormality.
The density of the tissue or fluid that the x-ray passes through will determine how it appears on the CT scan. The denser the object, the brighter it will be on the image. Hence, bone will show up on a scan as a very bright white. Acute blood will be white as well. This brightness is referred to as “hyperdense.” Air will be dark, and water or CSF will be relatively dark, all referred to as hypodense. Normal brain shows up as gray (isodense). For viewing, CT images need to be adjusted (referred to as window and level) for the particular range of structural density being assessed, e.g., “brain window” or “bone window.” CT demonstrates less contrast differentiation of soft tissue than MRI, for example, when looking at brain tumors or for infections. CT is also compromised in evaluation of the posterior fossa due to effects of the bone in the skull base (Fig. 18.3 shows a comparison of CT and MRI scans of the same patient with a posterior fossa tumor).


Fig. 18.3
Comparison of CT and MRI of same patient with posterior fossa tumor
If contrast is going to be administered, an IV will either need to be placed before the test begins or it can be given through a butterfly needle during the scan. The patient lies on a moving table which slides inside a circular opening (CT gantry). Restraints may be used with younger children to reduce movement during the procedure. The x-ray will then start to rotate around the patient. A CT scan of the head or spine can be done in about 2–10 sec.
18.6.1.2 Clinical Application
CT scan is the most commonly used imaging in trauma cases. It is the fastest way to obtain images of the brain and spine in a critical period. CT scanners are also widely available and can be found even in many rural facilities. Also because it is quick, CT can be performed without the use of sedation in most cases. Blood and CSF show up well on CT, so it can be used to look for an intracranial hemorrhage (Fig. 18.4) caused by a trauma (both accidental and non-accidental), as well as hydrocephalus (Fig. 18.5). It is also used to evaluate skull and spinal fractures. CT will generally be able to rule out most intracranial abnormalities that would require neurosurgical intervention. A CT scan of the brain and spine can also be used to look for tumors, abscesses/infection, and vascular anomalies that may be responsible for strokes or bleeds in children who present with neurological deficit. A CT scan is often obtained to confirm the diagnosis of craniosynostosis and provide operative planning, although the diagnosis can usually be made on physical exam alone. CT scan can be used to evaluate bony abnormalities in the spine, such as craniovertebral junction abnormalities and vertebral anomalies.



Fig. 18.4
CT head without contrast showing intracranial hemorrhage

Fig. 18.5
CT head without contrast. Hydrocephalus and transependymal flow
CT scans are used for guidance during brain and spine surgery, as they can provide coordinates for the navigation system in the operating room. Such navigation systems allow surgeons to track the location of their surgical instruments in relation to the anatomy of the brain. This helps the surgeon to perform more precise and less invasive surgical interventions.
18.6.1.3 Additional Considerations
CT scans use medical radiation, but it is important to remember that the benefit of obtaining a head or spine CT usually outweighs any potential risk. Low-dose protocols are being utilized more frequently in CT scans, especially as providers and radiologists consider the ALARA principle.
18.6.1.4 Nursing Care
Ensure that no metal is worn in the area being imaged during the study, as this can produce artifact and interfere with the accuracy of the imaging.
Sedation is rarely necessary to perform a CT scan of the brain or spine.
An important job of the nurse is to help decrease the patient and family’s anxiety by explaining the procedure and what will happen during the test. They should be assured that CT scan is not painful, but remember that having to lie still in the setting of an injury can be painful and anxiety producing.
If patient is receiving contrast, identify any problems with renal function prior to the scan.
Identify allergies, particularly to contrast.
Tell patient that if contrast is being administered, the contrast may cause a flushing sensation, salty or metallic taste in their mouth, a mild headache, or nausea but that these side effects are usually very short lasting.
Patients do not need to be NPO for CT scanning of the brain or spine even if contrast is being administered, as the contrast is given through an IV and does not need to be ingested.
Patients should be well hydrated following the procedure to ensure proper clearance of the contrast.
18.6.1.5 Special Considerations
3D reconstruction of a head CT can be helpful to further evaluate the bone structure of the head and to evaluate for abnormalities including better characterization of skull fractures (Fig. 18.6).


Fig. 18.6
CT head with 3D reconstruction showing skull fracture
18.6.2 Magnetic Resonance Imaging (MRI)
18.6.2.1 How Does It Work/Procedure
An MRI can provide incredibly detailed images of the brain (Fig. 18.7) and spine (Fig. 18.8) that cannot be seen on plain x-rays or CT scans. It not only permits the identification of abnormalities undetected by other imaging modalities but also facilitates the further evaluation of those that were detected otherwise. MRI is an exceptional imaging technique because it provides information about both anatomy and the actual physiologic process of the body.



Fig. 18.7
Normal brain MRI (T1 sagittal view)

Fig. 18.8
Normal spine MRI (T2 sagittal image)
MRI utilizes a large magnet along with radio waves (pulse) to exploit an atomic phenomena known as magnetic resonance. Since the human body is largely composed of water, hydrogen is a major component of all tissues and bodily fluids. A fractional percentage of it exists as hydrogen ions, which carry a positive charge. The MRI scanner creates a strong magnetic field, causing those hydrogen ions to “line up” along its axes. A radio frequency pulse is used along with manipulation of the magnetic field (through gradient coils) to alter the precession of the ions. Following the pulse, the hydrogen ions “relax” and emit energy which can be detected. The rate of relaxation differs depending upon the type of tissue or fluid they exist in. To oversimplify, MRI maps the differences in the relaxation rates (measured in milliseconds). Indeed, it was the discovery that ions in cancerous tissue generally relaxed at a slower rate than those in healthy tissue that led Damadian to first propose that nuclear magnetic resonance could be used for medical diagnostic purposes (Damadian 1971).
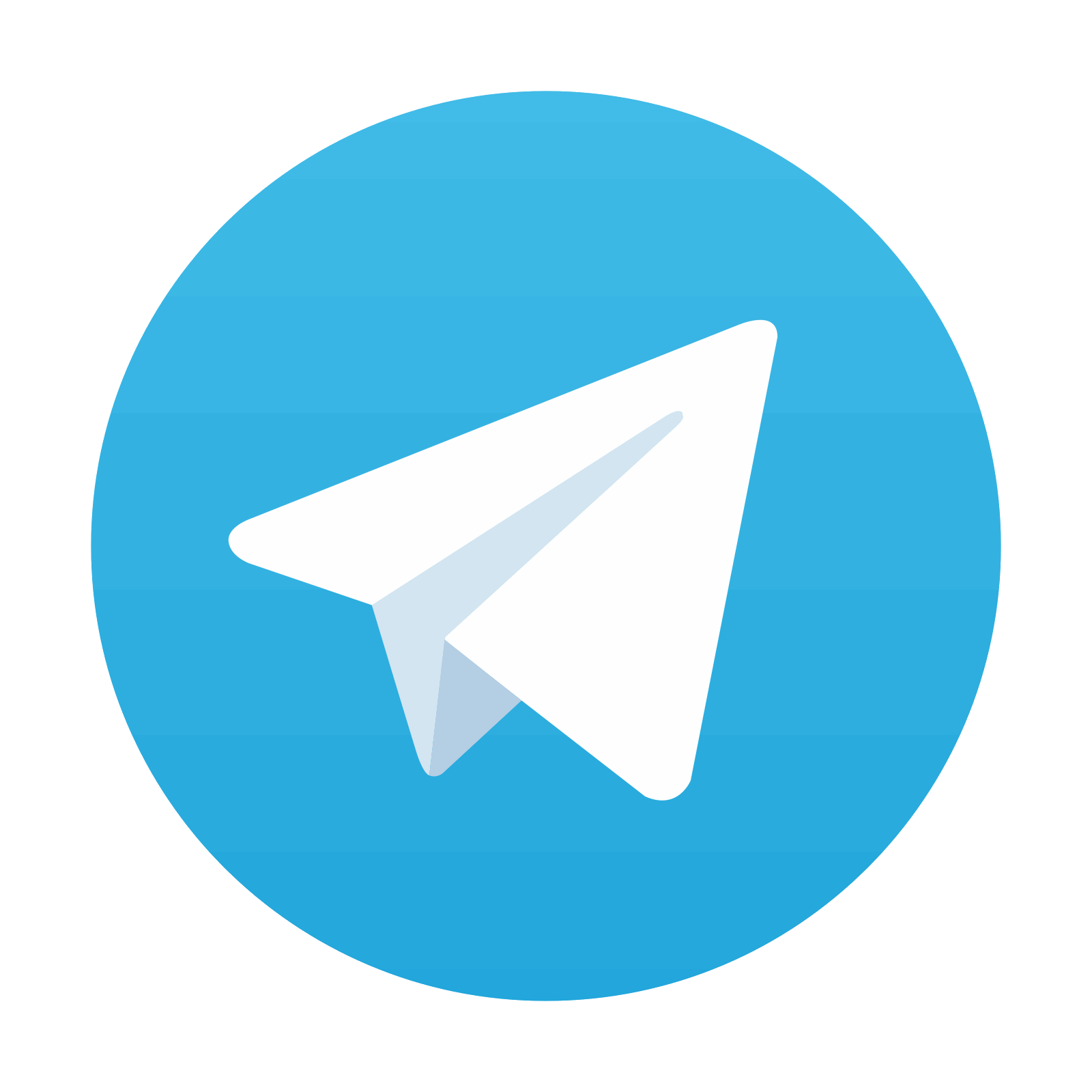
Stay updated, free articles. Join our Telegram channel

Full access? Get Clinical Tree
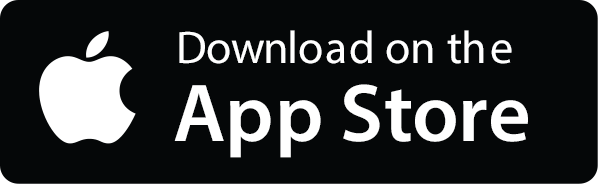
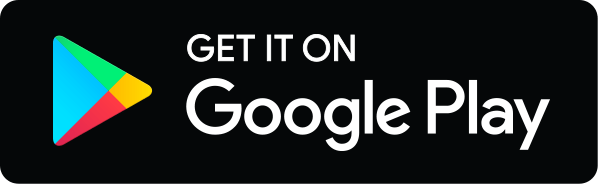