Chapter 11 Musculoskeletal Health Breakdown
When you have completed this chapter, you will be able to
THE MUSCULOSKELETAL SYSTEM
BONE: STRUCTURE AND FUNCTION
The four main functions of the skeletal system are
Bone is highly vascular and remains active metabolically throughout the life span.
HEALTHY BONE
Collagen fibres make up 90–95% of the organic composition of bone. These fibres run the length of bones and give it tensile strength. The cellular components of bone include1,2
To gain a clearer understanding of how these cells work together, see Figure 11.1.
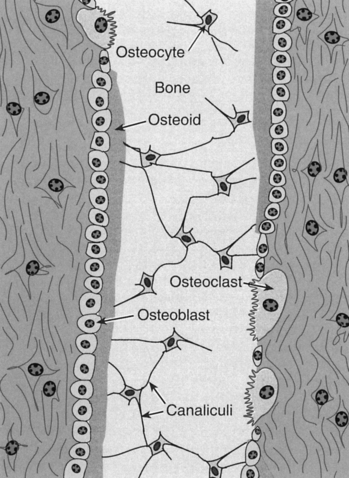
FIGURE 11.1 Microscopic detail of principal bone cells: three principal cells important to bone metabolism
Source: Rhoades, RA & Tanner, GA (eds.). 2003; Medical Physiology 2E, Lippincott Williams & Wilkins, Philadelphia, Fig. 36.3, p. 638.
The Haversian system is the basic structural unit within bone. Central to the system is the Haversian canal, which contains blood vessels and nerves. Around each Haversian canal are deposited the bony matrix as concentric rings (lamellae) where osteoblasts become osteocytes (see Figure 11.1). Extensions from the osteocytes, known as canaliculi, run as smaller canals linking lamellae to the Haversian canal. This system is important for transport of ions and nutrients to and from the blood to bone tissue.
BONE GROWTH
Major skeletal growth occurs in childhood. In a growing long bone, the epiphyses and the metaphysis are separated by a layer of cartilage (growth plate) where longitudinal growth takes place3. From infancy to adolescence, bones are actively growing in length, width, thickness and density (see Table 11.1). The epiphyseal plate gradually becomes less responsive to the hormones that stimulated them throughout childhood and adolescent growth. As hormones and tissues decline with age so too the status of the musculoskeletal system.
TABLE 11.1 SUMMARY OF BONE GROWTH
Bone Growth | |
---|---|
Infancy | Rapid dimensional growth as calcium is added to cartilage-like bones. Calcium content in relation to body size increases faster than any other stage of life3 |
Childhood | Dimensional growth: skeletal height, length and width4 in bone continue. Bone density and thickness increase. Bone deposition is greater than resorption |
Dimensional growth accelerates, bone growth peaks around ages 12–17 years in boys and 11–15 years in girls. Bone density and thickness increase while bone deposition outpaces resorption4 | |
Middle adulthood | Bone mass loss after people reach 35 years5 in both men and women |
Later life | Loss of height due to osteoporosis and intervertebral disc thinning for both men and women. Metabolic changes (menopause) and reducedactivity also contribute to osteoporosis. |
Cartilagenous thickening and continuing growth creates distance between the epiphyses and metaphysis1. Numerous hormones contributing to bone growth are summarised in Table 11.2.
TABLE 11.2 EFFECT OF HORMONES ON BONE GROWTH2
Other factors affecting bone growth are
CALCIUM, PARATHYROID HORMONE AND VITAMIN D
The level of calcium in the blood must be maintained within a narrow range to perform the regulatory functions of this ion. When serum levels of calcium are low, parathyroid hormone (PTH) is released in greater amounts from the parathyroid gland2, causing an increase in calcium absorption from the gastrointestinal tract, and further resorption by the kidneys into bone. When serum calcium levels are high, PTH secretion and activity are inhibited. PTH also regulates the synthesis of the active metabolites of vitamin D, which then increases gastrointestinal reabsorption. During infancy, bones grow rapidly as calcium is added to the cartilage-dominated bones and calcium content increases in relation to body size3.
Vitamin D is necessary for normal bone mineralisation because of its role in calcium and phosphorous metabolism. The processes by which this vitamin reaches its final biological effects are summarised in Figure 11.2. Two sources of vitamin D contribute to serum calciferol: photochemically produced vitamin D from skin 7-dehydrocholesterol6, known as cholecalciferol; and secondly, dietary vitamin D from plants and yeast. The second source, known as vitamin D2 (ergocalciferol), is produced by ultraviolet ray exposure of plants7, which we ingest.
CALCIUM HOMEOSTASIS AND REGULATION
Calcium homeostasis is regulated mainly by the effects of PTH and 1,25–(OH)2–D3 (see Figure 11.2) on intestinal absorption, renal tubular absorption and bone resorption. Dietary calcium deficiency is rarely a major cause of bone disease in that absorption of calcium increases in states of calcium deficiency. Calcium fluxes between the gut plasma, bone and kidney. The circulating pool of calcium (approximately 12 mmol/L) is small when comparing the body reservoirs and daily fluxes of calcium levels8,9.
SKELETAL MUSCLE: STRUCTURE AND FUNCTION
Approximately 40% of body weight is attributable to skeletal muscle10. The four main functions of muscle are to
SKELETAL MUSCLE CONTRACTION
A skeletal muscle is composed of many muscle fibres. Within each fibre are numerous muscle cells bundled together by a sarcolemma into fasciculi. Skeletal muscle is striated, meaning it has a microscopic appearance of being striped. This is due to the specific organisation of contractile proteins, actin and myosin10. Myosin is the thick filament, the thinner filament being actin. Fasciculi that lie parallel to each other, as found in striated muscle, have the combined functions of strength and extensive range of motion at the associated joint.
Free intracellular calcium is essential to muscle contraction. The myosin heads have a high affinity to actin filaments, but they are prevented from interacting by tropomyosin protein attachment to actin. The position of the tropomyosin on actin is controlled by troponin9,10. When calcium ions are not available, the actin binding sites are covered by tropomyosin (which is stimulated by troponin). When calcium ions do become available through their release from the sarcoplasmic reticulum (a large storage for calcium ions) or extracellular space, the actin binding sites also become available as tropomyosin moves to another position. This allows actin and myosin to quickly interact to form a cross-bridge, the essential activity of muscle contraction9.
ELECTROMECHANICAL COUPLING
To initiate contraction, a nerve impulse travels along the axon from the nerve cell body to muscle. The distal axon will divide many times, and these divisions will end on numerous motor end-plates. This nerve ending is positioned close to the sarcolemma. Acetylcholine is released to ensure depolarisation of the sarcolemma, which then spreads to the sarcoplasmic reticulum. When the action potential reaches the sarcoplasmic reticulum, calcium is released into the myofibril. This free calcium then stimulates chemical contraction, as the actin binding sites become available to bind with myosin. As a state of repolarisation is reached, the calcium ions return to the sarcoplasmic reticulum, and actin and myosin binding is reversed9,10.
Muscle is attached by tendons (fibrous tissue) or aponeuroses (flat sheets of connective tissue) to muscle and bone2. Ligaments (also fibrous connective tissue) bind bone to bone and are responsible for joint stability.
JOINTS: STRUCTURE AND FUNCTION
Synarthroses are joints that are very inflexible or fused joints2 such as skull bones or innominate bones (ileum or ischium) due to the presence of cartilage and fibrous tissue between bones. The symphysis pubis joint is an amphiarthrosis meaning the fibrous cartilage joining of the two pubic bones of the pelvis becomes flexible at specific times, for example, during the hormonal conditions of late pregnancy4. Diarthroses are those joints possessing a synovial sheath or joint capsule (examples are the hip or knee joint).
Most significant joints of the body are synovial joints. Articular cartilage covers the points at which bone may articulate with bone. The synovial membrane secretes synovial fluid into the joint cavity to lubricate and cushion activity. Synovial fluid also acts as a medium for nutrient/waste movement in and out of the joint1. Articular cartilage at the ends of bones that articulate with each other, are smooth, shiny and able to cope with stress induced by body movement. The larger surface of the bone functions to
Articular (hyaline) cartilage has no blood vessels, lymph or innervation. As a consequence, any defect in the cartilage will result in limited recuperation and disturbance to joint activity. Hyaline cartilage is 70% water and inorganic salts, lipids and glycoproteins1. The chondrocytes (cartilage secreting cells) produce the organic matrix of cartilage. During any health breakdown of the joint, the maintenance of articular cartilage can be severely altered, reducing its capacity to buffer against pressure and shearing forces. Uncharacteristic joint movement potentially disrupts natural joint activity. Therefore, a complex joint such as the knee, presenting with numerous articulations with complex ligament and tendon attachment, can potentially present with various joint health breakdowns.
NURSING IMPLICATIONS
Understanding the mineral requirements of bones, muscles and joints gives nurses a greater appreciation of how the electrical and chemical environments of these structures work. For example, the balance of calcium (and other associated ions, such as phosphate) is necessary for conditions of growth and regeneration. Nurses need to assist patients to optimise these chemical microenvironments. Therefore a musculoskeletal health breakdown requires nursing consideration of the following potential issues: pain, sleep disturbances, fatigue, changes in mobility (with associated risk for disuse syndrome) and linked to changes in ability to attend to ADLs5. To assist regeneration and repair of bone and muscle, adequate nutrition is required. These broad nursing implications will assist in refining specific implications for each case study.
TRAUMA
FRACTURES
A fracture is defined as morphologic damage to bone continuity or part of the bone, such as the epiphyseal plate or cartilage. An estimated 66% of all injuries affect the musculoskeletal system11 as a range of outcomes such as fractures and soft tissue injury. When fractures occur, tremendous reparation needs to be made to regenerate bone to original status. When a bone fracture occurs, the physical force causing the fracture will also cause damage to adjacent tissues/structures.
Fractures can be described by their anatomical position and arrangement of fragments. A classification of most fractures is described in Table 11.3.
TABLE 11.3 TYPOLOGY OF FRACTURES5
Avulsion | Fracture wherein a fragment of bone is pulled from a main bone by a ligament/tendon |
Comminuted | Fracture has fragmented into many small pieces |
Compound/open | Fracture includes tissue damage such as skin or mucous membranes e.g., compound comminuted fracture is a common combination |
Compression | Fracture in which bone is compressed or squashed |
Epiphyseal | Fracture through the epiphyseal plate |
Greenstick | Incomplete fracture common in children where in the bone is bent and not completely fractured through the bone |
Impacted | Fracture in which bone fragments are driven into each other |
Oblique | Fracture line lies angular to the bone shaft, making the fracture less stable |
Pathological | Fracture may occur with trauma or spontaneously but affects diseased bone such as osteoporotic bone, bone cancer or renal failure (see Chapter 7) |
Simple | Fracture does not break skin or mucous membrane |
Spiral | Fracture spirals through the bone shaft, caused by a twisting action |
Stress | Fracture caused by repeated activity ‘stressing’ the bone and associated muscles e.g., seen in athletes during repetitive training |
Transverse | Fracture line is straight across the affected bone(s) |
The five main stages of bone healing are outlined in Table 11.4, differing in their time frame according to age and state of health.
TABLE 11.4 STAGES OF BONE HEALING
Haematoma formation | Inflammation: 1–3 days |
Fibrocartilage formation | Reparative: up to the first 2 weeks |
Callus formation | Reparative: 2–6 week (may start earlier) |
Ossification | Reparative: up to 6 months |
Remodelling | Up to a year after injury |
DIAGNOSTIC TESTS
Enid had lost blood into the site of the fracture, and because she needed surgery for her fracture, the following investigations were conducted: haemoglobin, white blood cell count, creatinine, and electrolytes, such as sodium and potassium. These blood tests provide baseline data for comparison intraoperatively and after surgery. Also before anyone goes to theatre it is expedient to check that they have the capacity to oxygenate themselves (haemaglobin), have normal kidney function so they can excrete the anaesthetic (creatinine), do not have an infection (white blood cell count), and don’t have electrolyte derangement. Enid’s results showed a slightly lowered haematocrit, probably due to her blood loss, coupled with having intravenous fluids, which had diluted out the red blood cells she still had in her vascular compartment.
PHYSIOLOGY OF THE HIP JOINT
The hip joint is supplied by branches of the deep femoral artery. Most of the vessels traveling to the femoral head need to pass through the neck of the femur, the origin arising from a vascular ring at the neck base. The hip joint is a ball and socket synovial joint that allows movement in a variety of planes. The head of the femur articulates with the acetabulum of the pelvis. This particular joint has a tough, fibrous capsule maintaining femoral head and acetabulum articulation1.
WHAT IS THE PATHOPHYSIOLOGY?
Treatment for people in the older age group, such as Enid, is to surgically replace the joint with a hemiarthroplasty (replacement of fractured bone by a titanium prothesis) determined by the severity and configuration of the fracture11. Avascular necrosis of the head of the femur can occur if the vascular supply to the femoral head is disrupted. Secondary osteoarthritis is also a common complication.
WHAT IS THE TREATMENT?
Enid’s fracture was not displaced, so her fracture was immobilised by internal screwing of her two fractured bone surfaces to each other; hence, she did not need a hemiarthroplasty. The main aim of surgery is to facilitate swiftness of bone healing (see Table 11.3). As Enid has hypertension and therefore is at risk of perioperative myocardial infarction, a femoral nerve catheter was inserted by the anaesthetist, so the screws could be inserted under a regional anaesthetic.
Any procedure such as this, where artificial means are instigated to assist bone healing and a surgical incision is made, calls for prophylactic antibiotics. Enid was treated with an intravenous cephalosporin (cefuroxime, 750 mg eight hourly for two days) and consequently, did not acquire an infection.
The death rate within one year of a fractured neck of femur is between 20–35%12,13. In each case, these are reported as in-hospital mortality, not as deaths in the community.
APPLIED PHARMACOLOGY
As part of the nursing assessment the question needs to be posed, ‘Was Enid on any medications that would cause her to fall?’ For example, was she taking sleeping tablets or other medications that would make her drowsy or impair her balance? Another assessment consideration is to detail possible pharmacological reasons why Enid would have osteoporosis. Had Enid been taking steroids for some time? Over time steroids will generate a negative nitrogen balance hence causing a reduction in growth14.