CEREBRAL EDEMA FROM STROKE
Cerebral injury from either hemorrhage or infarction after acute stroke can herald the development of cerebral edema around the affected vascular area. The mechanisms of infarction and ischemia are discussed in Chapter 5. Cerebral edema, if allowed to proceed unchecked, can result in a cascade in which the zones of infarct, ischemia and edema, widen. For some people who experience a stroke, cerebral edema will be a minor issue because of the smaller size and location of the area of injury, whereas for others, it will be a significant and possibly life-threatening problem. When considering neuroanatomy, it is clear that the development of even a small amount of edema can cause devastating problems in a patient with a pontine infarct or hemorrhage, whereas an elderly patient with a normal age-related cerebral atrophy could develop a significant amount of edema without manifesting severe physiological compromise.
The development of global edema is sometimes seen after subarachnoid hemorrhage (SAH) and is associated with poor outcome and increased mortality (Claassen et al., 2002). Patients who initially present with intracerebral hemorrhage (ICH) or SAH are at higher risk for poor outcomes from cerebral edema because their intracranial pressure is already elevated as a result of the space-occupying lesion that the hemorrhage represents. Further complicating the picture is that SAH patients are at risk for cerebral edema from both acute and delayed cerebral infarct (Schmidt et al., 2007). Perihematomal edema in the setting of ICH develops rapidly and increases significantly in a week to 10 days immediately following onset of ICH (Staykov et al., 2011). Some patients with ischemic stroke will experience the complication of hemorrhage into the area of infarct. This can range from asymptomatic petechial hemorrhages seen on imaging studies to large, life-threatening intracerebral hematomas. This hemorrhagic transformation of infarct increases the risk of cerebral edema.
Cerebral edema becomes a problem when it leads to intracranial hypertension, more commonly known as increased intracranial pressure (ICP). Cerebral edema can damage and displace brain structures not initially affected by the stroke and, in about 2% to 3% of cases, leads to central herniation and death. Although younger people with strokes have less comorbidity, which could complicate the management of cerebral edema, they have less intracranial space to accommodate swelling than do older patients with age-related atrophy of cerebral tissue. Patients with ICH are also at risk for delayed cerebral edema several days after the initial event as the breakdown of the extravasated red blood cells results in irritation of surrounding tissue and swelling (Thiex & Tsirka, 2007; Xi et al., 2001). Massive cerebral edema and the resulting herniation are the most feared consequences after acute stroke especially in younger patients who do not have brain atrophy.
Because cerebral edema is often a serious complication of stroke and can be associated with significant morbidity and mortality, discussions with the patient and/or their family about goals of care should be initiated early in the management and treatment of stroke. Even with maximal medical intervention, some patients with cerebral edema will not survive, and others may sustain permanent deficits because of new or increased infarct as a secondary effect of cerebral edema. Having discussions related to end-of-life care or establishing the existence of completed advance directives or living wills prior to the development of complications can facilitate decision making. It can be difficult for families to make decisions regarding either initiating or withdrawing care if they have not had an opportunity to consider their options earlier in the patient’s hospital stay. In these cases, the palliative care specialists, if available, can be a vital support system and resource for the family and the interprofessional team in these cases.
Pathophysiology
Cerebral edema represents an abnormal increase of intracellular and/or extracellular water causing an increase in intracranial volume. The extent to which it is a local or generalized problem for a particular patient depends on the location of the original infarct or hemorrhage. Edema from a cerebellar lesion, for example, becomes a generalized problem when it obstructs the fourth ventricle and results in obstructive hydrocephalus. Swelling from cerebral edema is believed to peak between 2 and 4 days after initial injury, and there is a great deal of variation in the length of time it takes the edema to resolve. The greater the increase in ICP and the greater the number of interventions required to manage the increased ICP, the longer it usually takes for the edema to resolve.
Injury and ischemia trigger a harmful molecular cascade in which mediators such as free fatty acids, glutamate, or extracellular potassium are released or activated, leading to secondary swelling and nerve cell damage. The molecular cascade eventually encompasses the loss of membrane ionic pumps, which leads to cellular accumulation of water and eventually permanent damage to cellular functions (Jha, 2003). It is useful to keep in mind that there are three kinds of cerebral edema: cytotoxic, vasogenic, and interstitial. Cytotoxic and vasogenic edema often coexist in stroke patients (Rabinstein, 2006).
Vasogenic edema is seen most often in the white matter of the brain. It results from increased permeability of the endothelial cells in the capillary beds. As a result, a protein-containing filtrate leaks into the extracellular space. Vasogenic edema can develop around cerebral infarcts, brain tumors, and abscesses. Steroids have only been shown to be effective for edema related to brain tumors; osmotic therapy is more effective for other conditions (Rabinstein, 2006).
Cytotoxic edema occurs when there is an increase in fluids in neurons, glia, and endothelial cells as a result of adenosine triphosphate (ATP)–dependent sodium-potassium pump failure leading to the accumulation of sodium and fluid with the cells resulting in diffuse swelling. Both gray and white matter may be involved. Cytotoxic edema is often seen in relation to a hypoxic or an anoxic episode. It can also be seen with prolonged hypo-osmolar states such as water intoxication, hyponatremia, and syndrome of inappropriate secretion of antidiuretic hormone (SIADH). Steroids are not effective for this kind of edema, and therapy should be directed at restoration and maintenance of perfusion and oxygenation (Hickey, 2014; Rabinstein, 2006).
Interstitial edema is seen in prolonged hydrocephalus and is also known as transependymal flow. Cerebrospinal fluid (CSF) is not absorbed rapidly enough and is forced across ependymal tissue and into the periventricular white matter. It can be seen with both acute and subacute hydrocephalus. Patients with SAH are at high risk for interstitial edema because the location of the bleeding interferes with proper CSF drainage. Steroids are not an effective treatment for this type of edema, although acetazolamide can be used to decrease CSF production. Interstitial edema is initially best managed with temporary external drainage of the CSF. Some patients may require permanent alternative drainage through a surgical shunt placement (Rabinstein, 2006; Rincon & Mayer, 2008).
Assessment and Diagnosis
All clinicians involved in the patient’s care should know the size and location of the original stroke as well as the time since the stroke occurred. This information will guide the frequency of the neurological examination as well as the focus of the examination. A patient with a brainstem stroke, for example, would benefit from especially close monitoring of cranial nerves, whereas a patient with a right middle cerebral artery (MCA) infarct should be closely monitored for the development of or worsening of a left-sided weakness. The bedside nurse conducting frequent serial neurological assessments will likely be the first to observe subtle early neurological changes, which could indicate the presence or worsening of edema or the risk of herniation. These findings should be reported to the physician or advanced practitioner. The comparison of the current neurological examination to the patient’s baseline examination represents the most sensitive indicator of change.
A focal change in the neurological examination such as acute hemiparesis should prompt immediate concern about a localized intracranial event such as a hemorrhage or new infarct. A global change in the neurological examination such as deterioration from being completely intact to mildly confused, although it may herald cerebral edema, should also prompt evaluation of factors such as oxygenation, serum sodium, serum glucose, and body temperature, which can lead to neurological compromise. Computed axial tomography (CAT) scanning is an excellent tool for the rapid diagnosis of cerebral edema and can provide information to facilitate rapid intervention to limit patient deterioration. ICP monitoring can assist with the monitoring of cerebral edema and guide treatment (Jha, 2003). Although placement of an ICP monitor is still the “gold standard” for care, research is underway to investigate the various methods to allow noninvasive monitoring of ICP. Automated pupillometry, near-infrared spectroscopy, transcranial Doppler sonography, and optic nerve sheath diameter are some methods under investigation as ways of early identification of neurological deterioration (Rosenberg, Shiloh, Savel, & Eisen, 2011).
Treatment
Unstable stroke patients are best managed in a neurointensive care unit (NICU) staffed by a dedicated neurointensivist team. This model has been shown in studies to improve outcomes (Bershad, Feen, Hernandez, Suri, & Suarez, 2008; Diringer & Edwards, 2001). Initial basic management of all patients with stroke focuses on such measures as avoiding fever and hypoxemia, promoting cerebral venous drainage by keeping the head of the bed (HOB) elevated, and maintaining the head in a midline position. The overall goals of medical therapy are prevention of secondary complications through management of cerebral edema, maintenance of adequate blood and oxygen supplies, and optimization of cerebral metabolism (Singh & Edwards, 2013; Thiex & Tsirka, 2007). Proper management of hypertension, hyperglycemia, respiratory failure, and fever, as discussed elsewhere in this chapter, are integral to managing cerebral edema. Many of the factors associated with increased ICP have been identified and their management clarified through nursing research. Many of the interventions to manage patients at increased risk for problems related to ICP are collaborative problems and rely on astute nursing care rather than specific medical interventions (Olson, McNett, Lewis, Riemen, & Bautista, 2013; Olson, Thoyre, Bennett, Stoner, & Graffagnino, 2009).
The decrease in the level of consciousness often associated with cerebral edema frequently requires intubation and mechanical ventilation. Intubation and mechanical ventilation can make it easier and safer to manage pain, anxiety, and agitation because respiratory depression associated with pharmacological interventions is not a concern. Great care should be taken during intubation to avoid precipitous drops in blood pressure, which could worsen cerebral perfusion in the presence of elevated ICP. Propofol, in particular, can be associated with hypotension if not used judiciously during intubation (Mace, 2008). Continuous intravenous infusion of anxiolytics, amnestic agents such as propofol, and opioids can be helpful for the management of increased ICP, whereas other treatments are aimed at decreasing the edema (Finley Caulfield & Wijman, 2008). Stepwise protocols, originally designed for the management of traumatic brain injury, have been successfully applied to the management of cerebral edema after stroke. ICP monitoring has been routinely use for decades, but aggressive control of elevated ICP has not led to uniformly beneficial results. Nonetheless, ICP monitoring has been used to guide medical therapy and assist with the timing of possible surgical interventions. Osmotic therapy remains the bulwark of treatment of cerebral edema because of its rapid mode of action. Mannitol has been used for the longest time, but hypertonic saline has been gaining in popularity. The mechanisms by which mannitol works are not completely understood. It may work by a combination of decreasing brain volume through decreasing water content, decreasing CSF through the decrease in water content, improving perfusion through decreasing viscosity, and possibly acting as a neuroprotectant (Rabinstein, 2006).
Hypertonic saline in concentrations ranging from 2% to 23.5% is gaining in support as an osmotic diuretic with studies suggesting that it may be superior to mannitol (Mortazavi et al., 2012; Suarez, 2004). Hypertonic saline is believed to work by dehydrating brain tissue, reducing viscosity, increasing plasma tonicity, increasing regional brain tissue perfusion, diminishing inflammatory response to brain injury, and restoring normal membrane potentials (Suarez, 2004). Care of the patient receiving osmotic therapy requires vigilant monitoring of electrolytes and fluid balance. Fluid restriction has not been shown to directly affect cerebral edema and is to be avoided because of the potential for inducing hypotension and worsening the ischemic deficit. Controversy exists regarding the ideal level of blood carbon dioxide in the management of cerebral edema. In past years, hyperventilation was routinely used to maintain constant hypocarbia at around 25 mm Hg, but studies found that this practice was associated with cerebral vasoconstriction and increase in the size of the infarct (Broderick et al., 2007). More recently, laboratory investigations using animal models are studying permissive hypercarbia in neurological injury (Brambrink & Orfanakis, 2010; Glass, Fabian, Schweitzer, Weinberg, & Proctor, 2001). However, current best practices for the management of cerebral edema recommend maintenance of carbon dioxide levels in the normal range of 35 to 45 mm Hg for the stroke population. Induced hypothermia is also under investigation for the treatment of stroke-associated cerebral edema. Although various studies have reported encouraging preliminary results, larger trials are indicated because the therapy is not without risks or complications (De Georgia et al., 2004; Schwab et al., 2001).
Some subpopulations of patients require surgical intervention. For those with cerebellar lesions which are causing obstructive hydrocephalus, surgery is lifesaving. However, there is very limited research regarding the optimal management of patients with cerebellar infarct (Jensen & St. Louis, 2005). For patients with large MCA infarcts who are experiencing rapid neurological deterioration, decompressive hemicraniectomy should be considered. Several recent studies (e.g., DESTINY, HAMLET, and DECIMAL) have indicated that there is value in this procedure (Vahedi et al., 2007). However, the North American HEADFIRST trial, in which surgery was performed later in the course of the patient’s illness, failed to show improved outcomes. Thus, the optimal timing of surgery is not yet known. As providers discuss prognosis with patients and families, it is vital to give serious consideration to the likelihood that increased survival is also associated with the increased likelihood of significant disability because of the severity of the underlying stroke.
The most common form of ICP monitoring currently involves the placement of a catheter, which can also be used for CSF drainage as a way of treating elevated ICP. Although the measuring of ICP is helpful, other modalities have been developed to look at other physiological parameters, which can impact cerebral edema. There are devices available which continuously monitor brain temperature, brain tissue oxygenation, and the biochemical milieu of brain parenchyma. These have initially been used in patients who have experienced traumatic brain injury, but their use is expanding into other neurological patient populations (Singh & Edwards, 2013). It is still not clear if more intensive monitoring correlates with improved patient outcomes in stroke patients (Dhawan & DeGeorgia, 2012).
Regardless of which therapeutic interventions are required for an individual patient, no treatment should be withdrawn abruptly. Medications should be weaned very slowly with close monitoring for worsening of the patient’s condition. Mechanical ventilation should be carefully adjusted, as needed. If therapeutic hypothermia has been used, the patient should be gradually rewarmed, with close monitoring for indications of neurological deterioration (Diringer & Edwards, 2001). The clinical team should continue monitoring the ICP and other modalities in use to ensure that the patient is responding favorably to less intense treatment.
Given the likelihood of long-term disabilities associated with large-volume strokes, there are many studies underway that approach the issue from different perspectives. Some investigators are looking at early interventions designed to minimize the size of the initial stroke, whereas others are examining optimizing the timing of interventions by either improving monitoring techniques or developing new ones. Still, others are looking at ways to repair the damage wrought by the stroke by helping the body reverse the damage (Singh & Edwards, 2013). Most of these studies are in the very early stages of data collection. Ideally, given the morbidity and mortality associated with cerebral edema, all stroke patients at risk for this complication should be afforded the opportunity to participate in clinical trials.
Best Practices
As noted earlier, the unstable stroke patient is best managed in a NICU staffed by dedicated neurointensivists. Stroke patients outside of the ICU who are in the poststroke time frame, when they may still be at risk for the development of cerebral edema, are best managed by a dedicated stroke service (Jauch et al., 2013). Steroids have no role in the treatment of cerebral edema associated with stroke (Rabinstein, 2006). Hyperosmolar therapy remains a mainstay of treatment but requires appropriate monitoring modalities and gradual withdrawal once the clinical team feels that the patient no longer requires it (Rabinstein, 2006; Suarez, 2004). However, studies have not shown a clear link between the use of hyperosmolar therapy and improved long-term outcomes (Zazulia, 2009). ICP monitoring and drainage of CSF to relieve pressure remain mainstays of therapy. It remains controversial whether their use is associated with improved long-term outcomes (Jha, 2003). Mechanically ventilated patients should have their carbon dioxide levels maintained in the normal range of 35 to 45 mm Hg (Rabinstein, 2006). Hemicraniectomy is a valid treatment option for patients with life-threatening cerebral edema (Vahedi et al., 2007). Induced hypothermia is a promising therapy, which requires further study (Diringer & Edwards, 2001). Ongoing discussions about goals of care are integral to the management of the stroke patient with cerebral edema given the significant morbidity and mortality associated with its development. More studies to determine optimal management of cerebral edema in stroke patients are required (Singh & Edwards, 2013).
Evaluation
It is important to maintain a high index of suspicion for the development of cerebral edema in the first days or weeks after stroke. Should the patient develop cerebral edema, serial neurological examinations are used to evaluate the response to therapies, which are added in a step wise fashion. Serial neurological examinations coupled with normalization of data from various monitoring modalities show improvement. Once this occurs, therapies are withdrawn through a weaning process to ensure the patient no longer requires the interventions. Even with maximal medical therapy, some patients, particularly those with massive MCA infarctions, larger intraparenchymal hemorrhages, or the higher grades of SAH, will deteriorate, and the goals of therapy will switch to end-of-life care.
Clinical Pearl: A global change in the neurological examination such as deterioration from being completely intact to mildly confused, although it may herald cerebral edema, should also prompt evaluation of factors such as oxygenation, serum sodium, serum glucose, and body temperature which can lead to neurological compromise. Young patients who have a large stroke or SAH are at risk for developing cerebral edema and should ideally be transferred to a facility that has the ability for neurosurgical intervention in case the need arises.
ACUTE HYPERTENSIVE RESPONSE
An acute hypertensive response is defined by a systolic blood pressure (SBP) of 140 mm Hg or greater or a diastolic blood pressure of 90 mm Hg or greater in at least two recordings taken 5 minutes apart but within 24 hours of stroke symptom onset (Qureshi, 2008). This elevation of blood pressure (BP) occurs in about 75% of patients with ischemic stroke and in more than 80% of patients with ICH. Hypertensive response is independently associated with poor functional outcomes (Whitworth, 2003).
Because hypertension is a major risk factor for stroke, and many stroke patients have a past medical history of hypertension, it is usually difficult to distinguish inadequately treated or undetected chronic hypertension from acute hypertensive response in stroke. Distinct patterns, which suggest acute hypertensive response, include a reduction in the initial BP over a few days after admission without antihypertensive agents, or an initial requirement for more than one antihypertensive agent in the first few weeks post stroke followed by hypotensive events requiring rapid decreases in the patient’s medication regimen. The SBP will usually trend down to normal or near normal values after vessel recanalization in patients with known ischemic stroke, strongly suggesting that there are mechanisms specific to acute stroke that cause an acute hypertensive response in stroke patients (Qureshi, 2008).
Pathophysiology
Depending on the area of a stroke, death of brain cells in areas of the brain involved in the regulation of cardiovascular function can occur. Cardiovascular functions affected can include heart rate and BP regulation. In both cerebral hemispheres, inhibitory and excitatory input is provided by the prefrontal and insular cortices. This input then connects to the nuclei in the brainstem. In addition to the brainstem, the cingulated cortex, amygdala, and hypothalamus also provide modulation of inhibitory and excitatory input (Nason & Mason, 2004). Because of the widespread distribution of these modulation areas, most stroke lesions involve these areas to a certain extent, which leads to a disruption in autoregulation of BP. The widespread nature of cardiovascular function control in the brain is also supported by studies reporting that irrespective of the location of cerebral ischemia, stroke patients showed a parasympathetic cardiac deficit. In addition, patients with a right-sided stroke have increased sympathetic cardiac activity (Dütsch, Burger, Dörfler, Schwab, & Hilz, 2007). These findings place stroke patients at an increased risk for cardiac arrhythmia and other cardiovascular events. For example, patients who present with SAH or ICH have a high risk of increased ICP, particularly in the presence of brainstem compression. This increase in ICP is associated with an increase in systemic BP. However, this increase in BP does not automatically resolve with treatment of the ICP, and instead, BP slowly trends down with resolution of mass effect or cerebral edema. This further supports evidence that the primary cause of an acute hypertensive response results from damage or compression of specific cerebral regions that mediate autonomic cardiovascular control.
Assessment and Diagnosis
In the setting of acute stroke, management of acute hypertensive responses is generally guided by evidence-based policies or protocols developed by the facility. BP can be monitored adequately with an inflatable cuff in most patients with acute hypertensive response. However, for patients with ICH or SAH, those who require frequent titration of medications, or those with deteriorating neurological status, intra-arterial monitoring should be considered for more accurate and timely measurement of BP. In patients with a suspected increased ICP, ICP monitoring may be necessary to monitor and manage cerebral perfusion pressure during systemic BP lowering. Patients with a decreased level of consciousness, midline shift, or compression of basal cisterns on computed tomography (CT) scan may also be considered for ICP monitoring. Although some acute care hospitals allow use of intra-arterial monitoring of BP and titration of some vasoactive medications in noncritical care settings, ICP monitoring requires an intensive care unit setting, preferably one which specializes in the care of neuroscience patients.
If the patient received thrombolytic therapy with tissue plasminogen activator (tPA), there are specific guidelines regarding how often vital signs including BP should be checked in addition to titration goals for BP management. Hypertension greater than 185/110 mm Hg in the first 24 hours after intravenous (IV) tPA administration for ischemic stroke is associated with higher rates of cerebral hemorrhage. Frequent vital sign and neurological assessments are necessary because of the risk of rapid neurological and vital sign changes. Current recommendations for patients treated with tPA are to check vital signs including BP every 15 minutes for 2 hours, then every 30 minutes for 6 hours, and then every 60 minutes for 16 hours for a total of 24 hours (Jauch et al., 2013).
Nurses are vital for assessing and monitoring of vital signs because they are the frontline providers and can quickly detect any changes or trends in vital signs that require intervention. Nurses who provide routine care for stroke patients should be trained in detailed neurological assessment including standardized scales such as the National Institutes of Health Stroke Scale. The scales assist in objectively quantifying changes in neurological status and prime the nurse and provider into action based on these neurological changes. Because increased BP is a common occurrence in stroke patients, the nurse should consistently be evaluating whether the elevated BP is physiological due to acute stroke or due to stroke complications such as hypoxia, increased ICP due to hemorrhagic transformation of an ischemic infarct or herniation due to cerebral edema, or pain. Although patients usually have an IV antihypertensive medication as needed (PRN) order as part of a protocol order set, it may be necessary to notify the medical provider if there is consistent use of a PRN antihypertensive medication in order to control BP that remains elevated above set targeted goals. The interprofessional team caring for the patient should clarify on daily rounds the point at which a physician or advanced practice provider should be made aware of the use of PRN antihypertensive agents.
When assisting a patient out of bed for the first time after stroke, the nurse should check vital signs because some patients may have an orthostatic response (e.g., rapid drop in BP on arising) as a result of being immobilized on bed rest. The use of beta-blockers such as labetalol for SBP control also puts patients at increased risk for orthostatic hypotension. If a patient becomes acutely hypotensive due to orthostatic hypotension, which is not immediately recognized or appropriately managed, it can exacerbate neurological deficits due to ischemia and extension of the stroke or due to damage to the viable tissue surrounding the area of ischemia.
Treatment
Treatment of acute hypertensive responses vary depending on the type of stroke and clinical picture of the patient. The different subpopulations of patients are further addressed in the following texts.
Patients with acute ischemic stroke: The area of injury in ischemic stroke can be divided into different regions depending on the risk for further damage. First, there is a region of severe reduction in blood flow referred to as the core; there is also an area of moderate reduction in blood flow referred to as the penumbra (Qureshi, 2008).The penumbra has viable brain cells due to sustained blood flow via collateral blood vessels. However, because of impaired autoregulation in the area of injury, this area is vulnerable to further ischemic injury with systemic BP reduction. This is because a decrease in systemic BP and cerebral perfusion pressure result in a reduction in blood flow. The greatest risk, however, is not just a drop in BP for ischemic stroke patients, but more importantly, it is the wide fluctuations in BP within the first few hours after the stroke due to the impaired autoregulation around the area of ischemia that causes an expansion of area of infarct. Current recommendations are against routine lowering of BP in acute ischemic stroke unless it repeatedly exceeds 220 mm Hg SBP or 120 mm Hg diastolic BP in the acute period (Jauch et al., 2013).
Patients with acute ischemic stroke receiving thrombolytic therapy: The acute hypertensive response among patients with ischemic stroke receiving thrombolytic therapy is frequently transient and usually resolves with recanalization of the affected blood vessel. However, these patients will usually need reduction in their BP prior to infusion of thrombolytic therapy because significantly elevated BP has been associated with an increased risk of ICH. Current stroke guidelines recommend the reduction of BP to the thresholds used for inclusion in the The National Institute of Neurological Disorders and Stroke rt-PA (NINDS rt-PA) Stroke Study Group efficacy trial before thrombolytic therapy is administered. In that trial, treatment of hypertension to less than 185/110 mm Hg did not adversely affect functional outcomes at 90 days (“Tissue Plasminogen Activator for Acute Ischemic Stroke,” 1995).
Patients with ICH: About one third of patients presenting with ICH continue to demonstrate hematoma expansion with subsequent deterioration in the first few hours after onset of stroke symptoms, making BP control vital to reduce risk of hematoma expansion or rebleeding (Qureshi, 2008). An initial SBP greater than 150 mm Hg is associated with hematoma expansion and increased mortality among patients with ICH (Ohwaki et al., 2004).
Rapid reduction of BP in ICH patients is well tolerated likely because of reduced metabolism and preserved autoregulation in the perihematoma region unlike the disruption in autoregulation that occurs around the area of injury in ischemic stroke. The current guidelines recommend that for patients with SBP greater than 200 mm Hg or a mean arterial pressure (MAP) greater than 150 mm Hg, aggressive reduction in BP should be pursued using a continuous IV infusion of an antihypertensive agent with frequent BP monitoring. For those patients presenting with SBP greater than 180 mm Hg or MAP greater than 130 mm Hg and elevated ICP, consider reducing BP using intermittent or continuous infusion of medication while maintaining cerebral perfusion pressure greater than 60 mm Hg. If the patient presents with SBP greater than 180 mm Hg or MAP of greater than 130 mm Hg and no evidence of elevated ICP, then a modest reduction of BP (e.g., MAP of 110 mm Hg or target BP of 160/90 mm Hg) using intermittent or continuous IV medications to control BP is adequate (Morgenstern et al., 2010).
Patients with chronic hypertension: Chronic hypertension is a common diagnosis in stroke patients. Approximately 50% of patients who are admitted with a diagnosis of stroke have chronic hypertension requiring medication prior to admission (Qureshi, 2008). The abrupt discontinuation of antihypertensive medication to allow for permissive hypertension may lead to enhanced sympathetic activity, rebound hypertension, and a consequent increase in cardiovascular events in patients with coronary artery disease treated chronically on beta-blockers or those chronically on high doses of centrally acting drugs. The decision to continue or discontinue antihypertensive agents must be made on a case-by-case basis with the intent to avoid hypotension, excessive hypertension past SBP of 220 mm Hg, and myocardial ischemia, all of which can worsen neurological status resulting in prolonged hospitalization and adverse functional outcome. What may be less stressful to patients with cardiovascular disease is a reduction in the dose of the current agent or a change to a short-acting IV antihypertensive agent.
Patients with SAH: Most patients with aneurysmal SAH present with acute hypertensive response requiring IV infusion of antihypertensives prior to securing the aneurysm either by clipping or coiling procedures. About 4% to 17% of SAH patients will have a sudden episode of worsening neurological status that is suggestive of rebleeding within the first 12 hours of admission (Fujii et al., 1996; Ohkuma, Tsurutani, & Suzuki, 2001). Due to this risk of rebleeding, it is vital to control the BP to an SBP of less than 150 mm Hg (Ohwaki et al., 2004). Once the aneurysm has been secured, BP parameters can be liberalized even up to 200 mm Hg to allow for higher cerebral perfusion pressures. The higher cerebral perfusion pressure is vital especially for patient who have symptomatic cerebral vasospasm because the higher perfusion pressures can prevent secondary cerebral ischemia. The cerebral perfusion pressure is usually augmented using IV fluid boluses or high-volume infusions of IV fluids. No randomized trials of this intervention have been completed. However, clinical observations have revealed a rapid improvement in the neurological examination in many patients with this intervention and worsening when it is stopped prematurely (Connolly et al., 2012).
Best Practices
In patients with ICH, whose BP is not well managed with oral antihypertensive, continuous infusion of an antihypertensive agent should be used until the BP is controlled; once controlled, the patient can then be carefully transitioned to oral antihypertensives. In patients with ischemic stroke, continuous infusions of antihypertensive agents are rarely used for BP control to avoid quick reduction in BP and potentially resulting in worse neurological deficits. Practitioners are most likely to use a continuous infusion for BP control in ischemic stroke when the SBP is persistently elevated over 185 mm Hg in a patient who is eligible for thrombolytic therapy because there is need to control BP for thrombolytic therapy infusion to avoid ICH. The most common drug chosen for infusion is nicardipine because it has a rapid onset and short duration of action to allow for precise titration by the nurse at the bedside.
Based on the Joint National Committee (JNC) 8 recommendations, a targeted goal of a normal BP of less than 140/90 mm Hg for patients with diabetes mellitus or chronic kidney disease is reasonable after the acute stroke period. For the general population aged 59 years and younger, goal SBP is less than 140 mm Hg, whereas for those patients 60 years and older, the goal SBP is less than 150 mm Hg (James et al., 2014). Oral hypertensive agents can be initiated at 24 to 48 hours after symptom onset because most of the acute processes, such as risk to the ischemic penumbra and hematoma expansion, are uncommon after the first 24 hours. However, for some patients, permissive hypertension past an SBP of 140 mm Hg may be required even up to a week after acute stroke depending on neurological stability. The JNC 8 report does not address acute stroke management of BP; however, previous versions addressed this. The JNC 7 report recommended that the BP be maintained at intermediate levels (around 160/100 mm Hg) until neurological stability is achieved (Chobanian et al., 2003). The American Heart Association agrees that some patients may need higher BPs in the immediate period postischemic stroke with current recommendations to individualize therapy based on patient stability (Jauch et al., 2013). Special consideration includes patients with bilateral severe carotid stenosis, who may bear a high risk for stroke with aggressive BP lowering until carotid revascularization is performed. Other complications of stroke such as elevated ICP, progressive cerebral edema, ongoing cerebral ischemia due to occlusive vessel disease or symptomatic cerebral vasospasm, and postoperative cerebral changes require individualized management. When neurological stability is achieved, it is vital to pursue aggressive BP treatment to appropriate goal for secondary prevention of recurrent stroke because long-term benefits are noted in persons with and without a history of hypertension.
There is evidence that mortality is greater among patients in whom beta-blocker therapy is begun within 48 hours of symptom onset of stroke (Barer, Cruickshank, Ebrahim, & Mitchell, 1988). There is also evidence that long-term beta-blockers do not reduce risk of stroke and should not be used as first-line treatment for hypertension management. Thus, unless a patient has underlying cardiovascular disease, a beta-blocker should not be the first-line treatment for reducing BP (Wiysonge et al., 2007).
Traditionally, hemodynamic manipulation to allow for higher cerebral perfusion pressures has consisted of hemodilution, hypervolemia, and hypertensive therapy referred to as triple-H therapy. The focus now has shifted away from triple-H therapy to the maintenance of euvolemia and induced hypertension in select cases of symptomatic vasospasm (Connolly et al., 2012).
The American Heart Association/American Stroke Association (AHA/ASA) has published guidelines on how to manage stroke patients. Please see Chapters 6 to 8 on ischemic stroke, hemorrhagic stroke, and SAH for specific recommendations from these guidelines.
Evaluation
Ongoing evaluation of BP continues throughout the hospitalization. The primary care provider (PCP) is vital postdischarge for ongoing monitoring and management of BP. Medication regimens that include a once-a-day medication, or at most, twice-a-day regimen, are the most conducive to compliance by the patient. Every stroke patient should be evaluated by the PCP within a week to 2 weeks after discharge from an acute setting to ensure that no new stroke complications have occurred after discharge and to continue titration of antihypertensive therapy to the targeted goal BP.
The patient with hypertension should be encouraged to check his or her own BP routinely at home and keep a log that is reviewed with the provider to ensure that the BP goal is being maintained. Other secondary conditions such as obstructive sleep apnea should be considered in patients with chronic hypertension because there is evidence that sleep apnea is associated with an increased risk of stroke (Redline et al., 2010). Furthermore, use of continuous positive airway pressure (CPAP) for management of sleep apnea may be indicated to support BP control.
Clinical Pearl: Transition of care plays a critical role in the long-term clinical outcomes for patients and, when done well, can be significant in reducing readmission rates. Communication with the patient’s PCP regarding hospital course and plans for long-term specialty care contributes to well-thought-out transition of care. Medication regimens that include a once-a-day medication, or at most, twice-a-day regimen, are the most conducive to compliance by the patient. It is often useful to preemptively assure male patients with hypertension that should they experience sexual dysfunction due to an antihypertensive agent, this is reversible and can be managed by switching to a different antihypertensive agent. This approach is important because if symptoms are experienced before this discussion, patients are likely to be noncompliant in the future for fear of medication side effects.
POSTSTROKE SEIZURES
Stroke is the most common cause of seizures and epilepsy in the elderly (Stefan & Theodore, 2012). Poststroke seizures occur in about 10% of the patients. There is a greater risk of seizures in patient with hemorrhagic stroke compared to ischemic stroke (Burneo, Fang, & Saposnik, 2010). Poststroke seizures are associated with poor functional outcome, higher mortality at 30 days and 1 year, longer hospitalization, and greater disability at discharge (Burneo et al., 2010). A higher rate in hospital mortality has also been associated with poststroke seizures (Vernino et al., 2003). This is likely related to worsening of preexisting neurological deficits at the time of seizure onset. This worsened clinical status can last anywhere from a week to several months (Hankey, 1993).
Patients with late-onset or remote symptomatic seizure are at highest risk of developing epilepsy than those with early-onset seizures. Although a small number of stroke patients actually develop epilepsy, studies have shown that late-onset seizures post stroke is an independent risk factor for the subsequent development of epilepsy. More than half of those patients with late-onset seizures develop epilepsy (Bladin et al., 2000). Risk factors for poststroke seizures include presence of cortical lesions (Bladin et al., 2000), large lesions involving more than one lobe or an entire lobe (Kilpatrick et al., 1990), male gender (Giroud et al., 1994), and lesions from cardioembolic phenomenon because this etiology tends to involve more than one lobe as well (Kilpatrick et al., 1990).
Pathophysiology
In those patients with ischemic strokes, early-onset seizures can be the result of regional metabolic dysfunction and neurotransmitter release secondary to ischemic hypoxia. Acute ischemia causes increased extracellular concentrations of glutamate. This is an excitatory neurotransmitter that may result in secondary neuronal injury particularly in the ischemic penumbra resulting in epileptogenic potential (Camilo & Goldstein, 2004). Other metabolic derangements at the time of stroke onset can increase the risk of forming an area of epileptogenic focus. Experimental models have demonstrated that hyperglycemia and alterations in sodium or calcium levels enhance the development of seizures (Uchino, Smith, Bengzon, Lundgren, & Siesjö, 1996). The ischemic penumbra may contain electrically irritable tissues that provide a focus for seizure activity. Thus, the size of ischemic penumbra also plays a potential role in the development of seizures. Studies have shown a correlation between the total amount and duration of depolarizing events with the penumbra size in ischemic stroke (Back, Ginsberg, Dietrich, & Watson, 1996; Camilo & Goldstein, 2004).
Contrary to early-onset poststroke seizures, late-onset seizures are most likely caused by structural changes to the brain tissue such as the formation of gliosis in the area of an old ischemic injury and the development of intracerebral scar tissue (Jennett, 1978). Poststroke changes to cell membranes and the subsequent development of collaterals during stroke recovery may also lead to the development of hyperexcitability of those areas, which leads to an epileptic focus that can then lead to seizures (Stefan & Theodore, 2012). In ICH and SAH, the cause of seizures is likely related to the presence of neurotoxic agents such as red blood cells and iron (Chusid & Kopeloff, 1962; Willmore, Sypert, & Munson, 1978). Late-onset seizures have a similar frequency in ischemic stroke and ICH, further suggesting a structural reason for seizures as that one provided by gliotic scarring (Jennett, 1978; Stefan & Theodore, 2012).
Assessment and Diagnosis
Seizure presentation can be clinical, in which case seizure activity is observed by providers or family. Presentation of seizures can also be subclinical, in which case the patient may have nonspecific physical assessment finding that prompts initiation of electroencephalographic (EEG) monitoring which then confirms seizure activity electrographically. Most often, EEG changes after stroke are nonspecific. However, when changes are present post stroke, they tend to be focal, especially in ischemic stroke, where there is usually focal slowing of EEG activity in the area of injury, loss of normal background activity, and reduction of overall amplitude (Cheung, Tsoi, Au-Yeung, & Tang, 2003). In ICH, slowing is not only focal but can also be diffuse or multifocal if vasospasm is present, which is often the case with SAH (Laino, 2007). Other possible findings include periodic lateralizing epileptiform discharges (PLEDs) and sharp activity and frontal intermittent delta activity (FIRDA) (Horner, Ni, Duft, Niederkorn, & Lechner, 1995; Niedzielska et al., 2001). The presence of PLEDs is usually indicative of recent infarction and has been associated with watershed infarcts (De Reuck, Goethals, Claeys, Van Maele, & De Clerck, 2006; Stefan & Theodore, 2012).
The registered nurse (RN) is vital in identifying subtle neurological changes to the patient’s assessment, which may clue the interprofessional team that the patient may be having a waxing and waning neurological examination typical of seizure activity. The RN staff is also instrumental in keeping the patient safe should actual clinical seizures occur because many facilities have a protocol or plan of care related to seizure patients. This plan of care may involve padding bed rails and having the patient ambulate with assistance. The poststroke patient usually is admitted to a dedicated neuroscience unit with a neurologist directing his or her care. However, for those facilities without a dedicated neuroscience unit, collaboration with a neurologist may be warranted for assistance in identifying the appropriate therapy for the patient. Collaboration with the pharmacist is also vital to make sure of the selection of an appropriate pharmacotherapy agent with less side effects and interactions with other medications.
Treatment
There are no specific guidelines addressing the management of poststroke seizures because most of the studies addressing this condition have been observational or prospective studies. Most recommendations for the treatment of poststroke seizures are currently based on expert opinion or on consensus based on the available observational studies. The prophylactic initiation of anticonvulsants for patients with stroke who have not had a seizure is not recommended (European Stroke Organisation [ESO] Executive Committee & ESO Writing Committee, 2008; Stefan & Theodore, 2012). If a patient should have a poststroke seizure, there continues to be varying recommendations regarding how to manage the patient after that first seizure in the acute setting. Some experts recommend that in the case of a first time acute seizure onset, other causes of seizures should be ruled out; treatment should only be instituted if the patient has a second seizure or if the first seizure was prolonged or the patient was in status epilepticus with that first occurrence (Abbott, Bladin, & Donnan, 2001). However, given evidence of worse functional outcomes for patients with seizures, other experts recommend that once other causes of seizures have been ruled out, treatment with antiepileptic drugs (AEDs) should be started. This is because even if the occurrence was a first time seizure for the patient, the patient remains at increased risk of both status epilepticus and remote seizures as compared to other stroke patient (Ferro & Pinto, 2004).
Patients who develop status epilepticus should be appropriately treated with AEDs to be continued at discharge from the hospital. Although patients who develop epilepsy post stroke most often have a favorable outcome in terms of seizure freedom with monotherapy, a referral to a neurologist should be initiated for continued management postdischarge because treatment with AEDs does not reduce the risk of recurrent seizures after stopping AEDs (Gilad, Lampl, Eschel, & Sadeh, 2001; Stephen & Brodie, 2000).
Gabapentin and lamotrigine have the most efficacy and effectiveness as initial monotherapy for treatment of poststroke seizures in the elderly with partial seizures (Glauser et al., 2006). A study conducted by the Veterans Affairs (VA) confirmed this finding as well as with patient tolerance. Gabapentin and lamotrigine worked well as compared to carbamazepine (Rowan et al., 2005). Arif et al. (2010) retrospectively looked at effectiveness of AEDs in older adults with epilepsy, and they found that lamotrigine had the highest 12-month retention rate at 79%; levetiracetam, 73%; carbamazepine, 48%; gabapentin, 59%; oxcarbazepine, 24%; phenytoin, 59%; and topiramate 56%. Thus, first-line pharmacotherapies for poststroke seizures are lamotrigine, levetiracetam, and gabapentin (Arif et al., 2010).
Best Practices
EEG, although very helpful in the diagnosis of seizures and epilepsy, does not rule out the presence of epilepsy if the results of testing are negative. It is always important for the interprofessional team to integrate clinical and assessment findings when making the decision about treatment or diagnosis of seizures. Before starting AEDs, the team should weigh the risk of anticonvulsant therapy with the individual patient especially as many will be elderly and particularly susceptible to drug reactions and interactions (Stephen & Brodie, 2000). Interaction with warfarin should especially be considered because many stroke patients are receiving warfarin for treatment of atrial fibrillation or other cardiovascular conditions. Drugs with a better tolerability profile and a favorable drug interaction profile may be more ideal especially for the elderly. Once-daily dosing can help to improve compliance as well. Newer agents may have improved tolerability profiles, but they may be most costly and thus could limit their use. It is also important to remember that medications such as carbamazepine and phenytoin which are classified as enzyme inducers have the potential to increase cardiovascular and cerebrovascular disease risk because they are potent inducers of the cytochrome P450 enzymes, which are involved in cholesterol synthesis (Mintzer & Mattson, 2009).
Evaluation
Ongoing monitoring for seizures is important for patients who have poststroke seizures, most especially as they recover and undergo community reintegration post stroke. If the patient had an acute seizure post stroke and treatment was initiated, then he or she may need to be reevaluated to determine if it is appropriate to wean off AEDs once he or she has advanced in his or her recovery. The patient who has seizures post stroke will also need monitoring by a neurologist to ensure a seizure-free period before he or she is permitted to drive again because many states have laws regarding how seizure patients use their privilege to drive. If patients are on other cytochrome P450 inducers, they may need ongoing monitoring for lipids and liver functions tests to ensure stability and tolerance of medical therapy.
Clinical Pearl: Patients, who have a gaze preference or deviation on initial physical assessment after stroke, should be evaluated for seizures if the gaze preference changes to the opposite side. This is usually informally referred to as wrong-way eyes. This is because when a patient has a stroke, the eyes look toward the side of the lesion. When a patient has a seizure, the eyes look away from the side of the lesion.
In the Unites States, there are laws related to people with seizures being able to drive while under treatment. Providers should be aware of the laws in their states so that they can help protect the public and advocate for their patients as well.
FEVER AND STROKE
Body temperature above 37.5°C is associated with a worse outcome and prognosis following acute stroke. Fever control is an important aspect of stroke patient care. Multiple retrospective studies published in the late 1990s and early 2000s confirmed experimental data linking fever, especially early in the course of acute stroke, to poorer outcomes (Hajat, Hajat, & Sharma, 2000; Phipps, Desai, Wira, & Bravata, 2011). Studies have demonstrated that close to 50% of patients will develop fever after acute stroke often from systemic infection. Patients who have experienced aneurysmal SAH are at higher risk for fever when there is intraventricular extension of the blood or a poorer overall clinical grade (Fernandez et al., 2007). Fever in stroke patients may result from infection, central fever from the stroke itself, side effects of medications, or a combination of factors. Early fever after stroke may be an indicator of the cause of the stroke such as infective endocarditis or of systemic infection such as urinary tract infection or early pneumonia (Jauch et al., 2013). Sinusitis from nasogastric tubes or naso- or oroendotracheal tubes should not be overlooked as a cause of fever (Aggarwarl, Azim, Baronia, & Kumar, 2012).
Patients at higher risk for infection and subsequent fever after stroke are older, have higher National Institutes of Health Stroke Scale (NIHSS) scores, and have a large-volume infarct. Pneumonia occurs in up to 22% of stroke patients and is the most common cause of death (Coplin, 2012; Wartenberg et al., 2011). Fever accelerates injury and normothermia preserves tissue; each 1-degree elevation in temperature on the Celsius scale raises the basal metabolic rate by 6% to 7%. A common observation in patients with neurological injury is that fever worsens the observed neurological examination, whereas control of fever improves it. One study reported a 3-month mortality rate of 1% in a normothermic group of stroke patients and a rate of 16% in the febrile group (Andrews et al., 2002). Fever in the neuroscience population has been shown to extend ICU stay and raise hospital costs (Diringer, Reaven, Funk, & Uman, 2004; Reaven, Lovett, & Funk, 2009).
Pathophysiology
Fever has been defined as an adaptive complex, coordinated autonomic, neuroendocrine, and behavioral response, which is often part of the acute-phase reaction to an immune challenge from both infectious and noninfectious causes (Saper & Breder, 1994). The mechanisms associated with fever’s detrimental effect are thought to be the increase in metabolic demand, free radical production, and an increase in excitatory neurotransmitters. Reversing fever has been shown to cause multiple favorable cellular responses. Normothermia or hypothermia is associated with decreased release of the excitatory neurotransmitters glutamate and glycine, decrease release of calcium, decreased formation of free radicals, and decrease in AMPA-related delayed excitotoxic injury. This translates clinically into decreased cerebral metabolic rate and oxygen demand, decreased carbon dioxide production, and decreased ICP. The first concern in a hospitalized stroke patient with fever should be the development of acute infection, but noninfectious causes should simultaneously be considered.
Assessment and Diagnosis
The bedside RN monitors serial temperatures and looks for subtle global changes in the neurological examination. Patients with more severe strokes would likely benefit from continuous rather than from intermittent temperature monitoring to enable more intensive management of impending fever. Continuous monitoring includes the ability to alert the nurse when a parameter is exceeded, allowing for more timely interventions. For stroke patients not admitted to the ICU, intermittent temperature monitoring should occur at least every 2 hours in the initial few weeks after stroke and whenever a change in patient status raises concern for fever as a contributing factor. The clinical team collectively and regularly assesses for adventitious breath sounds; monitors closely for signs of aspiration; inspects indwelling device sites for signs of inflammation, infection, or the development of skin breakdown; and assesses urine for signs of infection such as cloudiness or malodor. The interprofessional team monitors for evidence of leukocytosis and thrombocytosis with complete blood counts. Such clinical indications of infection should lead to a fever workup including pan cultures and possibly a chest radiograph. Serial chest radiographs to monitor for development, progression, and resolution of infiltrates or atelectasis can be ordered. Noninfectious causes of fever such as acute pulmonary embolism (PE), acute myocardial infarction (MI), acute pancreatitis, phlebitis, acute gout flare, or drug hypersensitivity reaction should be considered and ruled out, as appropriate. Based on medications which the patient may have recently received, neuroleptic malignant syndrome and malignant hyperthermia should also be considered.
Treatment
All methods of maintaining normothermia or inducing hypothermia are based on the principle that heat in the body is transferred from a higher to a lower temperature. The four methods of heat transfer are radiation, evaporation, conduction, and convection (Guyton & Hall, 2006). Radiation is the most passive method of heat transfer. Keep the environment cooler than the patient while avoiding shivering. Evaporation involves water on the skin evaporating and causing cooling as this occurs. Again, avoiding shivering is critical. Conduction involves the exchange of heat between two surfaces and is the principle underlying traditional cooling blankets. Convection involves the transfer of heat from a surface to a fluid and is the principle underlying intravascular cooling methods.
Surface cooling has always been an imperfect method of treating or avoiding fever. It is very difficult to achieve and maintain goal temperature. Shivering is a significant and difficult problem to manage; there is always concern about skin breakdown. Rewarming cannot be performed in a controlled manner, and it is very nursing labor intensive. Newer methods of surface cooling have been developed, which address these concerns and which can provide controlled cooling and rewarming (Mayer et al., 2004; Scaravilli, Tinchero, & Citerio, 2011). The difficulties inherent in these methods of cooling led to research into convection and the development of intravascular cooling devices. Intravascular cooling allows for rapid control of temperature with less shivering, maintenance of temperature in the desired range, controlled gradual rewarming, and is less nursing intensive.
Shivering, while a normal response attempts to induce hypothermia, is counterproductive in patients with neurological injury. It raises metabolic rate, increases oxygen consumption, and can lead to increased ICP (Mayer et al., 2004; Presciutti, Bader, & Hepburn, 2012). Attempts to control shivering often require increased sedation as well as the use of systemic paralytic agents. Deployment of these therapies increases the risk of pneumonia, increases time on mechanical ventilation, increases time in the ICU and interferes with neurological assessment, and places the patient at increased risk for critical illness myopathy or neuropathy (CIM or CIN). These are complications of critical illness seen in patients who have experienced severe multisystem organ dysfunction, often with sepsis (Kress & Hall, 2014). They are often seen in those who required prolonged use of IV paralytic agents. CIM and CIN present as muscle weakness and failure to wean from mechanical ventilation. Research is ongoing regarding the underlying cause; the diagnoses should be considered in patients experiencing difficulty in weaning with new bilateral weakness or paralysis (Ydemann, Eddelien, & Lauritsen, 2012). A simple bedside shivering assessment tool can be used to trend shivering in response to adjustments in therapies (Presciutti et al., 2012). Recommendations garnered from the available literature advocate for establishing and treating the underlying cause of the fever and the use of appropriate antipyretic measures (Gross, Sung, Weingart, & Smith, 2012; Jauch et al., 2013; O’Grady et al., 2008). However, consensus does not yet exist on optimal measures. It is not clear from studies if there is a definite improvement in long-term outcome if measures are taken to avoid fever through prophylactic use of either antipyretic medications or antibiotics.
If the patient is believed to be at high risk for hemorrhagic conversion of the stroke, some clinicians prefer the use of acetaminophen over ibuprofen or aspirin. Studies indicate that current antipyretic medications are only minimally effective, suggesting that escalation to other measures to manage fever should occur early in the course of management (Jauch et al., 2013). IV antipyretics have not been studied sufficiently to recommend routine use over available enteral forms of these medications (Coplin, 2012). Because there is data suggesting that acute stroke patients who are hypothermic on presentation have better outcomes, inducing normothermia or hypothermia with either surface or intravascular, cooling may be attempted. Great care must be taken with these methods to avoid shivering, which will only increase metabolic demand and hinder fever reduction. Ice packs and traditional cooling blankets have been shown to be ineffective and erratic in consistently reducing fever. Induced cooling is often very uncomfortable for the patient. Because it may require significant sedation and possibly intubation and mechanical ventilation to avoid shivering, induced hypothermia should only be undertaken in the ICU where the appropriate level of monitoring can occur. There is ongoing research, particularly in the area of inducing hypothermia, studying optimal temperature management post stroke (Coplin, 2012). When induced hypothermia is used as a therapy, the nurse and clinical team needs to be even more vigilant in monitoring for developing infections because fever will be suppressed. Aggressiveness in the treatment of fever should be tailored to the perceived risk of deterioration and worsened outcomes in specific patients.
Prevention of fever is the best method of avoiding the complications associated with fever in the stroke patient. Indwelling devices should be maintained, changed, or removed in accordance with institutional policies. Because pneumonia is the most likely infection in acute stroke, measures to prevent and combat pneumonia should be instituted. Several interventions can be implemented. The HOB should be maintained at 30 degrees or higher to prevent aspiration. Patients who are able to cough and deep breathe or participate in the use of incentive spirometry should be taught and encouraged to do so. Use of specialty beds which can provide rotation or chest physical therapy can be useful. Vest percussion to help loosen secretions can be helpful. If the patient is high risk for aspiration, nursing staff should scrupulously adhere to recommendations from speech therapy (ST). These recommendations include that meals adhere to the recommended consistency, liquids are appropriately thickened, and the patient either feeds himself or herself or is fed in the manner recommended by ST, which usually involves eating slowly and alternating small bites of food with small sips of liquids. Family members who wish to assist with feeding should be taught appropriate techniques and then monitored intermittently by the nurse to ensure they are consistently using the techniques.
Best Practices
Many national and international health care organizations have issued guidelines endorsing aggressive fever management in the neuroscience patient population. Pneumonia and urinary tract infections are the most common infections in stroke patients (Wartenberg et al., 2011). The use of the ventilator-associated pneumonia (VAP) bundle for prevention of pneumonia and compliance with standards on early removal of urinary catheters are supported in the literature (Institute for Healthcare Improvement, 2012). There are no widely accepted guidelines regarding frequency of obtaining cultures and chest radiographs for the evaluation of a new fever in a hospitalized patient. There is no agreement regarding the long-term benefits of inducing hypothermia in the acute stroke patient, although the success of the therapy with other patient populations is encouraging. Studies of the benefits of hypothermia are currently underway (Jauch et al., 2013).
Evaluation
Evaluation for fever is an ongoing process throughout the hospital stay of the stroke patient, although more frequent measurements of temperature and interventions to maintain normothermia are indicated in the first several days after acute stroke when it is most critical to protect ischemic tissue and hopefully limit the size of the infarct. The clinical team works together to implement therapies in a timely fashion and change therapy as indicated while monitoring closely for complications of therapy.
Clinical Pearl: Patients with drug-induced fever often look better clinically than patients with fever from an infectious cause. A diagnosis of drug-induced fever should be a diagnosis of exclusion, making sure that all other infectious etiologies have been well investigated first.
RESPIRATORY FAILURE AND STROKE
The incidence of respiratory failure associated with stroke varies, depending on the mechanisms underlying the stroke. The majority of patients who experience ischemic stroke will not require early intubation (Jauch et al., 2013). Patients at highest risk are those with a large-volume cerebral infarcts or infarctions involving the brainstem. Only 5% to 10% overall may require intubation, but up to 25% of those with ischemic MCA stroke may need intubation. The mortality rate for acute stroke patients requiring intubation is higher than seen in those who do not require intubation (Milhaud, Popp, Thouvenot, Heroum, & Bonafé, 2004; Santoli et al., 2001). Patients with ICH strokes have significantly higher rates of intubation and mechanical ventilation and significantly higher early mortality rates as well (Broderick et al., 2007; Towfighi, Greenberg, & Rosand, 2005). Patients who sustain an aneurysmal SAH may require intubation acutely, depending on the severity of the SAH, whereas others will only require it while undergoing the securing of the ruptured aneurysm (Seder & Mayer, 2009).
Unfortunately, emergent intubation and mechanical ventilation may be required before the likely stroke outcome is known or advanced directives have been addressed. Early and frequent discussions with family and patient, to the extent that the patient is able to participate, are critical in determining whether intubation will be part of maximal medical intervention or whether extubation and withdrawal of support will be more appropriate. Older patients who are comatose at the time of admission and intubated have the poorest outcomes even with aggressive therapy (Foerch, Kessler, Steckel, Steinmetz, & Sitzer, 2004). The reason for delayed intubation is often related to pneumonia from aspiration. Again, discussions with family and patient will determine whether the best course of action for that patient will encompass maximum therapy or if palliative care is more appropriate. The AHA/ASA has published guidelines on palliative and end-of-life care in stroke to guide practice (Holloway et al., 2014).
Development of acute respiratory distress syndrome (ARDS) is rare (2% to 4%) in acute ischemic stroke but is associated with higher mortality (Rincon et al., 2014). A recent study of patients with hemorrhagic stroke found a significantly higher incidence (25%) than was seen in ICH (Elmer et al., 2013), whereas another study found an incidence of approximately 27% in patients with SAH (Kahn et al., 2006).
In some patients, it may be possible to manage airway problems in the short term with CPAP or bilevel positive airway pressure (BiPAP) and avoid intubation. Some studies have shown that stroke patients, especially those with cardiac or pulmonary disorders, are at high risk for developing hypoxemia in the days immediately following the stroke (Roffe et al., 2003; Sulter, Elting, Stewart, den Arend, & De Keyser, 2000). However, limited data exist about the use of supplemental oxygen in patients who have not demonstrated hypoxia through documented oxygen desaturations. Rather, the interprofessional clinical team, and in particular, the bedside nurse, should closely monitor the acute stroke patient for signs of partial airway obstruction, aspiration, incipient pneumonia, or the development of irregular breathing patterns, all of which can lead to hypoxia seen at the bedside as oxygen desaturation. Incipient pneumonia is based in a clinical judgment that a particular patient’s difficulties with managing oral secretions, compromised ability to cough effectively, and impaired swallowing are beginning to manifest as abnormal breath sounds, subtle changes in respiratory effort, and low-grade fever which may herald a need to increase such measures as chest physiotherapy to avoid pneumonia. All members of the interprofessional team should be cognizant of applicable medical history such as the diagnoses of chronic obstructive pulmonary disease (COPD), congestive heart failure (CHF), or obstructive sleep apnea (OSA) (Jauch et al., 2013). If a patient arrives with the diagnosis of sleep apnea, he or she should be encouraged to use his or her home machine for management while hospitalized. If the patient has not been using a device, this should be discussed with the patient and family, and the importance of proper management of sleep apnea is stressed. If sleep apnea is noted in a patient not previously diagnosed, a sleep study and fitting of a proper device can be ordered. Given that sleep apnea is an independent risk factor for stroke, managing it properly is important for secondary prevention after stroke (Durgan & Bryan, 2012; Yaggi et al., 2005).
In patients in which intubation has resulted in placement of a tracheotomy tube, two considerations need to be addressed. The first is weaning from mechanical ventilation, and the second is weaning from the tracheostomy back to a natural airway. A study which investigated tracheostomy rates in patients with ischemic stroke found that less than 2% required a tracheostomy, but 33% of patients who underwent decompressive craniectomy required a tracheostomy (Walcott et al., 2014). The optimum timing for a tracheostomy in patients with acute ischemic or hemorrhagic stroke has not been well studied, but a large multicenter trial is underway (Bösel et al., 2013). Early tracheostomy is anecdotally favored for patient comfort and is associated with increased ease of weaning from mechanical ventilation.
Pathophysiology
Respiratory failure can result in both hypercapnia and hypoxemia, which can worsen stroke outcome by expanding ischemic injury and raising ICP. The three primary pathways leading to acute respiratory failure in acute ischemic stroke are disturbance of respiratory rhythm generation, interruption in descending respiratory pathways, or bulbar weakness leading to aspiration. If a patient has comorbidity such as OSA or COPD, is a current tobacco smoker, or is using illicit substances, he or she may develop respiratory failure earlier or more easily. Normal respirations are maintained by a balance of metabolic, autonomic, voluntary, and limbic inputs. The metabolic or autonomic pathways maintain acid–base balance and oxygenation. Lesions in the pons and medulla can disrupt these pathways leading to an inability to maintain adequate respirations particularly when the patient is sleeping. Impaired autonomic ventilatory control can be manifested in a variety of abnormal and inadequate breathing patterns. Apneustic and ataxic patterns of breathing require intubation in patients who are receiving aggressive medical therapy and may herald impending death in patients who are receiving palliative care.
The development of hiccups requires close monitoring because they may signal a medullary lesion and can be a precursor to the development of a pathologic breathing pattern. Primary central neurogenic hyperventilation is rare but problematic because of the resulting derangement in acid–base balance. Interruption of voluntary respiratory pathways can lead to respiratory apraxias such as the inability to cough voluntarily, initiate swallowing, or breath holding. Cheyne-Stokes respirations represent an alteration in voluntary control of breathing and can be seen in up to 50% of patients after unilateral supratentorial infarct but may also be seen in infratentorial infarct as well. Table 9-1 shows the abnormal breathing patterns.
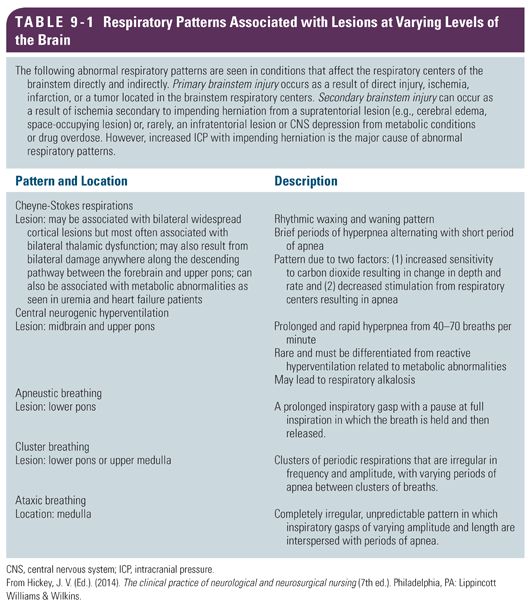
Such patients may require intubation to prevent episodic hypoxemia from compromising the ischemic penumbra and increasing the size of the infarct. Limbic or emotional respiration represents a descending pathway that preserves respiratory modulation to emotional stimuli including laughing, coughing, and anxiety. Patients with bulbar lesions are at increased risk for aspiration pneumonia because of a combination of impaired swallowing, abnormal respiratory patterns, reduced vital capacity, and reduced or absent triggering of the cough reflex. Pneumonia from a variety of pathogens is seen in approximately 20% of patients after acute stroke. Patients with ICH strokes are at high risk for respiratory failure given that they are more likely to have vomited at the time of stroke (Broderick et al., 2007). Patients with high-grade SAH may develop respiratory failure requiring intubation and mechanical ventilation for a variety of reasons, including poor neurological function, aspiration, or acute lung injury related to SAH (e.g., neurogenic pulmonary edema) (Davison, Terek, & Chawla, 2012; Seder & Mayer, 2009). Hypoxemia may worsen the effects of cerebral ischemia and should be avoided.
Assessment and Diagnosis
The initial assessment of patients with acute stroke will determine if they are at increased risk for respiratory failure. As noted earlier, some patients will be obviously distressed and require intubation at the scene or in the emergency department. The RN and other members of the interprofessional clinical team will need to collect an accurate clinical history, most often from records or from the patient’s family, to determine if there are comorbidities and risk factors such as COPD, OSA, or use of tobacco or illicit substances. The NIHSS can be used to determine existence and severity of dysarthria. Dysarthria, which is often accompanied by dysphagia, indicates that the patient likely has difficulty managing secretions and is at high risk for aspiration and possibly pneumonia and respiratory failure. In many institutions, the bedside RN can administer a bedside swallow test according to a protocol to determine initial risk for aspiration. If the stroke patient is found to be at risk for aspiration, nothing by mouth (NPO) status is maintained until assessment is completed by an ST for recommendations to guide the timing of reintroduction and progression of oral intake.
The bedside RN and other members of the interprofessional clinical team will monitor the work of breathing, assess for the development and/or progression of adventitious breath sounds, and evaluate the patient’s ability to manage secretions. Respiratory therapists with experience in working with neurological patients are integral to managing ventilator settings, monitoring weaning parameters for either extubation or liberation from the ventilator, and providing input for weaning and removal of the tracheostomy tube as the patient improves. Throughout the weaning process, the interprofessional care team uses frequent and serial assessments of the work of breathing (e.g., respiratory rate and depth, use of accessory muscles), adequacy of cough effort, and ability to clear secretions to ascertain how well the patient is progressing with the weaning process. Ideally, the process will proceed smoothly and rapidly, but there are often setbacks and plateaus; the interprofessional team should be certain that the patient and family understand this aspect of the process.
Treatment
The nurse encourages the patient to participate in basic pulmonary toilet such as coughing and deep breathing and use of an incentive spirometry. If chest physiotherapy, with either a specialty bed or a chest vest, is prescribed by the interprofessional care team, the nurse ensures the therapy is carried out and monitors patient response. Some studies support the use of CPAP starting the first night after stroke in patients with OSA as a way to improve long-term outcome post stroke (Lévy & Pépin, 2011). If the patient has required intubation and mechanical ventilation, the nurse monitors response to therapy and works with the interprofessional care team to wean the patient from mechanical ventilation and to move toward extubation. The interprofessional care team works collaboratively to manage any anxiety related to the weaning process. Stroke patients often meet respiratory criteria for extubation, but the care team may hesitate because of concerns about ongoing ability of airway protection. Use of the Glasgow Coma Scale (GCS) score may be helpful in predicting successful extubation in patients with MCA stroke. However, some stroke patients who required intubation will require tracheostomy placement. Other stroke patients who were intubated initially will become candidates for transition into palliative care, and their extubation will be terminal and managed in conjunction with the palliative care team. The bedside nurse along with the entire interprofessional team works to establish and maintain a therapeutic relationship with the patient and family to facilitate decision making regarding intensity of care.
The ST and respiratory therapist (RT) work with the interprofessional team to implement the use of speaking valves or capping trials and determine the optimal timing of tracheostomy progression to decannulation (Christopher, 2005; Morris, McIntosh, & Whitmer, 2014). However, a lack of standardization exists regarding tracheostomy weaning, and nurses can be integral in the effort to develop and establish standardized protocols (Morris et al., 2014). The bedside nurse is key in assessing patient’s readiness and tolerance to weaning, but a systematic approach to tracheostomy management provided by a dedicated team is likely to result in fewer complications during weaning.
It is not readily apparent from a review of the literature how often patients with acute stroke will not be able to be weaned either from mechanical ventilation or from dependency on an artificial airway. Patients whose stroke has resulted in a locked-in syndrome will often require tracheostomy and prolonged mechanical ventilation (Smith & Delargy, 2005). Unfortunately, tracheostomy increases the difficulty for out-of-hospital placement for stroke patients. Studies, which have investigated long-term outcome for all patients requiring long-term tracheostomy, have found high mortality rates in the first year (Engoren, Arslanian-Engoren, & Fenn-Buderer, 2004).
Artificial airway placement complicates the already impaired ability of a stroke patient to communicate with loved ones and his or her care team, and it increases the stress in an already stressful situation. Nonverbal communication methods such as facial expressions, gestures, pointing, eye movement or blinking, and possibly sign language are encouraged for patients with artificial airways, although the stroke may have already significantly compromised communication abilities. An ST can determine whether some sort of communication device such as letter or picture boards, flashcards, or more sophisticated devices may be appropriate for a particular patient. The bedside nurse ensures the implementation and appropriate use of communication tools by all members of the care team.
Best Practices
There are no prospective trials, which have established an optimal mode of ventilation for stroke patients. For those patients who have required intubation and mechanical ventilation, the mode of ventilation is often related to the reason for intubation and the sedation requirements of the patient. An RT with experience in the care of patients with neurological disease including stroke is invaluable in the appropriate management of stroke patients. Groups of interventions, or “bundles,” have been introduced in the last decade for the prevention of common ICU complications. The VAP bundle, which includes HOB elevation, daily sedation vacations, assessment of extubation readiness, peptic ulcer disease prophylaxis, deep vein thrombosis prophylaxis, and proper oral care, has been shown to significantly reduce the incidence of VAP (Tolentino-DelosReyes, Ruppert, & Shiao, 2007; Wip & Napolitano, 2009). However, use of the VAP bundle is not definitively supported by large clinical trials so that controversy exists about the use of the bundle (O’Grady, Murray, & Ames, 2012). Sometimes, patients with stroke will require a tracheostomy but, as noted earlier, there is no clear data regarding timing of tracheostomy. Additionally, there is no clear data about the number of stroke patients who will be discharged from an acute care hospital to either a long-term care facility or home with a tracheostomy in place.
Evaluation
Evaluation of respiratory function in the stroke patient is a continuous process requiring frequent reassessments by all members of the care team for any evidence of change. Stroke patients who have required intubation and mechanical ventilation will require daily assessments of their ability to be weaned and liberated from mechanical ventilation. Those who have gone on to require tracheostomy placement will require daily reassessment of their ability to progress to decannulation. Those patients who did not initially require an artificial airway will require close monitoring in the days immediately following the stroke to identify any deterioration in their ability to continue to protect their airway or for the development of pneumonia. Patients in whom the decision has been made to transition to comfort measures only will require monitoring for signs of distress, which may require intervention for comfort such as glycopyrrolate to alleviate for copious secretion management. End-of-life care is discussed in more detail later in this chapter.
Clinical Pearl: If at all possible, patients able to tolerate a progression to decannulation prior to discharge should have a care plan that allows progression to decannulation at the care facility where they are placed. Long-term care facilities usually have a limited number of beds for patients with tracheotomies, so having a structured plan in place for tracheostomy tube removal can facilitate placement.
HYPERGLYCEMIA
Hyperglycemia has been noted to be a common occurrence in acute stroke patients, especially during acute ischemic stroke, with more than 40% of patients presenting with hyperglycemia. Patient presenting with ICH and SAH also experience hyperglycemia with three out of four patients presenting with SAH having hyperglycemia on admission (Kruyt et al., 2009). There is evidence that hyperglycemia is associated with poorer neurological outcome at 3 months despite adjustments for age, stroke severity on admission, other vascular risk factors, and diabetes mellitus (DM) (Badjatia et al., 2005; Bruno et al., 2002; Kruyt et al., 2009). In ischemic stroke, there is evidence that hyperglycemia is only associated with nonlacunar strokes (Bruno et al., 1999). Among ischemic stroke patients treated with IV recombinant tissue plasminogen activator (rtPA), hyperglycemia has been associated with symptomatic ICH and subsequent poorer clinical outcomes (Bruno et al., 2002). The association between DM, hyperglycemia, poor glycemic control, and stroke outcomes remain controversial mainly due to the lack of controlled studies that specifically define optimal glucose control during acute brain ischemia.
Pathophysiology
Many studies have evaluated hyperglycemia in acute stroke, but there has been no consensus regarding a specific mechanism responsible for hyperglycemia not associated with DM encountered in stroke patients. In part, it is difficult to separate those patients with DM, whether known or unknown, from those patients experiencing hyperglycemia related to acute ischemic stroke. DM is a risk factor for ischemic stroke. It is much easier to identify hyperglycemia related to acute cerebral injury in SAH because DM is not a risk factor for SAH (Feigin et al., 2005). Another possible explanation for elevated glucose levels at admission is that many stroke patients present in a nonfasting state and therefore may have elevated glucose levels. Another mechanism implicated in hyperglycemia associated with acute stroke is a stress response mediated partly by the release of cortisol and norepinephrine (O’Neill, Davies, Fullerton, & Bennett, 1991). This stress response has also been implicated in SAH. It is believed that the activation of the sympathetic autonomic nervous system results in increased levels of cortisol and catecholamines, which persist even up to 10 days post-SAH (Naredi et al., 2000; Vergouwen et al., 2010). The increased levels of these hormones promote multiple process including glycogenolysis, gluconeogenesis, proteolysis, and lipolysis, all resulting in excessive glucose production and hyperglycemia (Barth et al., 2007; Seematter, Binnert, Martin, & Tappy, 2004).
Assessment/Diagnosis
Hyperglycemia is most often defined as blood glucose levels greater than 140 mg/dL via finger stick glucose check or serum glucose check. Many patients are placed on NPO until a formal speech evaluation is completed, which usually is within 24 hours of admission. Thus, in the time prior to this formal evaluation, the patient is only on non–dextrose-containing IV fluids unless there are contraindications such as dialysis-dependent renal failure. Routine blood sugar checks every 6 hours while NPO are recommended. In addition, it is recommended that every stroke patient be screened for DM by checking a glycosylated hemoglobin level. This will assist the interprofessional clinical team in determining whether hyperglycemia at admission is the result of undertreated or untreated DM or is associated with an acute reaction to the stress of the stroke.
The nursing staff is vital in recognizing patients who have elevated glucose levels because they are the interprofessional team members responsible for monitoring blood glucose at the bedside in addition to oral intake of food and fluids. Many patients with baseline diagnosis of DM will have their oral hypoglycemic medications held while on NPO status and during hospitalization due to unpredictable nutritional intake and risk of lactic acidosis if medications such as Glucophage are administered to patients who received contrast medium needed for some diagnostic studies. The pharmacist is also a vital interprofessional team member and is helpful in determining appropriate medication selection for those patients with newly diagnosed DM or for the patient presenting with hyperglycemia difficult to control in the acute setting either due to preexisting diabetes or due to ongoing critical illness.
Treatment
Currently, there is no clinical evidence that targeting blood glucose to a particular level during acute ischemic stroke will improve outcomes. The main risk from aggressive hyperglycemia correction in acute stroke appears to be possible hypoglycemia. It is reasonable to follow the current American Diabetes Association (2010) recommendation to maintain the blood glucose in a range of 140 to 180 mg/dL in all hospitalized patients. Dysphagia and dysarthria are complicating problems in the glucose management of acute stroke patients. Many stroke patients, after evaluation by ST, are initially placed on diets with modified textures and fluid viscosities. The nursing staff is vital in ensuring adequate patient intake of both food and fluids and reporting any difficulties to the rest of the interprofessional care team. The nutritionist/dietitian works with the patient and family to first identify food preferences, which will increase adherence to diets involving modified textures and viscosities and, secondly, to discuss ways to improve adherence to a healthy diet after discharge. The ST continues to engage the patient in therapy to assist with strategies to avoid aspiration resulting in complications such as pneumonia or pneumonitis. Scheduled home insulin regimens will likely require significant modification from home doses, and if the patient is a newly diagnosed diabetic, the assistance of a DM management specialist will be helpful especially in providing education regarding management, preventing of complications, and adherence to a diabetic diet.
Best Practices
Nutritional intake varies among stroke patients, and adjusting hyperglycemia treatment to suit oral intake is difficult in the few days to weeks following stroke, complicating efforts to maintain the targeted glucose level of 140 to 180 mg/dL. The current recommendation is to use regular insulin to treat hyperglycemia but to err on the side of mild hyperglycemia rather than hypoglycemia because mortality is increased for patients with hypoglycemia compared to those with hyperglycemia. This is why the goal for glucose is not less than 140 mg/dL but rather above the 140 mg/dL mark (Jauch et al., 2013). The care team should communicate this information to the patient and family so they understand this temporary deviation from what may or will become long-term management goals for the patient.
Once the patient’s hyperglycemia is resolved and DM has been ruled out, routine glucose monitoring should be discontinued to avoid unnecessary testing, support cost savings, and avoid potential medical errors associated from administration of regular insulin to a patient who does not need insulin administration.
Evaluation
Patients who present with hyperglycemia should have their glucose levels followed, but unless diagnosed with DM, they should not have any oral hypoglycemic agents started in the acute setting. Patients with pre-DM should receive ongoing nutritional counseling to assist with lifestyle changes to prevent or slow progression of hyperglycemia. Patients whose hemoglobin A1C suggests poor blood glucose control should be referred to an endocrinologist for more aggressive control to prevent further complications from diabetes.
Clinical Pearl: If DM medications are started or changed during hospitalization, compliance can be increased if affordability is taken into consideration because some of the newer insulin preparations are expensive if the patient is uninsured or may carry higher copayments. It is especially important to take time to educate the patient in layman’s terms regarding the disease process and nutrition, especially stressing that DM is a chronic condition that requires long-term follow-up and management.
DEEP VEIN THROMBOSIS
The risk of deep vein thrombosis (DVT) in hospitalized patients is a well-recognized complication. It is even more vital to monitor for this serious complication in stroke patients than in other patient populations due to the increased likelihood of prolonged immobility. Some stroke patients are at greater risk due to a previous history of DVT, dehydration, malignant disease, or clotting disorders (Kappelle, 2011). DVT occurs in up to 50% of patients with ischemic stroke who did not receive pharmacological prophylactic therapy. These DVTs most often develop between days 2 and 7 after stroke onset (Brandstater, Roth, & Siebens, 1992) with about 80% of the DVTs occurring in the first 10 days post stroke (CLOTS Trials Collaboration, 2009). DVT is associated with increased mortality and morbidity in stroke patients. Patients with ICH tend to have an increased risk of DVT compared to ischemic stroke patients. This is likely due to the recommended delay in initiation of pharmacological prophylaxis in hemorrhagic stroke as well as more severe focal deficits.
Clinically, a DVT may be asymptomatic. The major concern associated with asymptomatic proximal DVT is the potential for PE. This is a major clinical concern because it is believed that PEs account for 13% to 25% of early deaths after stroke (J. Kelly, Rudd, Lewis, & Hunt, 2001). Fatal PEs are unusual in the first week post stroke or in the acute setting after stroke unless a patient has a prolonged acute setting stay. PEs are most frequent between the second and fourth weeks post stroke when patients have been discharged home or to a rehabilitation facility (Viitanen, Winblad, & Asplund, 1987). Nonfatal PEs usually result in significant morbidity due to impaired cardiorespiratory reserve adversely affecting rehabilitation and functional outcome.
Pathophysiology
Factors contributing to the development of venous thrombosis include venous stasis, activation of coagulation factors, and vein damage. These factors are commonly referred to as the Virchow triad. Venous stasis can occur in the presence of anything that slows or obstructs the flow of venous blood, resulting in an increase in viscosity of the blood and the subsequent formation of microthrombi. When microemboli are not washed away by normal blood flow, an accumulation of microemboli can form in an area resulting in a thrombus that propagates. Endothelial (intimal) damage, whether due to intrinsic factors or due to external trauma to a blood vessel, can also result in thrombus formation because once there is endothelial damage, a hypercoagulable state occurs due to a biochemical imbalance between circulating coagulation factors. After the initial damage, platelets adhere to the subendothelial surface due to the presence of von Willebrand factor or fibrinogen in the vessel wall. Neutrophils and platelets are activated, and they release inflammatory mediators. Resulting complexes that form on the surface of platelets increase the rate of thrombin generation and fibrin formation. As a result, a mature thrombus composed of platelets, leukocytes, and fibrin develops in the wall of the vein, resulting in a DVT (Kuijper et al., 1997).
Assessment and Diagnosis
The nursing staff, physical therapist (PT), and the occupational therapist (OT) are critical team members in the prevention of DVTs. The nurse ensures that PT and OT have timely access to the patient so that they can complete an initial evaluation for ambulation safety and mobility. Recommendations from PT and OT provide direction for the safe and early mobilization and ambulation of the patient. The nurse can then assist the patient with mobility because PT and OT may not work with the patient on a daily basis in the acute care setting. The nurse is also the interprofessional team member best situated to regularly inspect the patient’s leg for signs of DVT development and communicate such findings to the rest of the care team.
Doppler ultrasound is an effective and economical technique for detecting symptomatic proximal DVTs. However, there is evidence that the sensitivity of Doppler ultrasound for the diagnosis of asymptomatic proximal DVT is only 62%, and for asymptomatic below-knee DVTs, only 48% (Wells, Lensing, Davidson, Prins, & Hirsh, 1995). However, many studies reporting these findings were completed in high-risk postoperative patients. The stroke patient population may have other characteristics that complicate DVT diagnosis unlike the sample in the reported studies. Although thrombi missed with ultrasound screening tend to be smaller and nonocclusive, it has been suggested that the technique may be unsatisfactory as a routine screening tool because many DVTs will not be detected. Thus, other tests such as serum levels of D-dimers are used in conjunction with ultrasound technology for patients in whom DVT is strongly suspected but not confirmed by ultrasound.
An alternative to ultrasound is magnetic resonance imaging (MRI), which is noninvasive and allows simultaneous imaging of the venous system in both lower limbs. In addition, pelvic vein and inferior vena cava thrombosis are accurately identified, which presented an important advantage over other techniques (Fraser, Moody, Morgan, Martel, & Davidson, 2002). However, it is an expensive modality, and it is has limited availability.
D-Dimers are composed of cross-linked fibrin and are formed as a waste product in the degradation of the fibrin matrix of fresh venous thromboemboli (J. Kelly et al., 2001). Acute nonlacunar ischemic strokes increase D-dimer levels. An elevation in D-dimer will usually decrease to baseline over the first 30 days post stroke. This elevation has stimulated debate about use of D-dimers in stroke patients, resulting in multiple studies specifically investigating the use of a D-dimer assay as a screening test for subclinical DVT in stroke patients. There is evidence that in patients who are on average 25 days post stroke, a threshold of 1,092 ng/mL has a sensitivity of 100% and specificity of 66% for diagnosing DVT as detected by Doppler ultrasound (Harvey, Roth, Yarnold, Durham, & Green, 1996).
Although these findings support the use of D-dimer as a screening test to allow the provider to identify patients who should undergo targeted imaging with ultrasound or MRI, the results cannot be applied to patients in the first few days after stroke. The other important issue to remember is that several D-dimer assays are now available for commercial use, and they are not all interchangeable. The results from studies using one manufacturer’s test cannot necessarily be applied to another manufacturer’s test. Thus, when providers translate evidence related to D-dimer assay usage into practice, it is important to keep this in mind.
Treatment
Ischemic stroke patients should be started on pharmacological DVT prophylaxis upon admission, unless they received thrombolytic therapy with tPA in which case providers will need to wait for a full 24 hours prior to initiating pharmacological DVT prophylaxis (Jauch et al., 2013). For patients with ICH, pharmacological DVT prophylaxis can be started 3 to 4 days post onset of hemorrhage, as long as there is no evidence of ongoing rebreeding (Wu et al., 2011). Patients with aneurysmal SAH can be started on pharmacological DVT prophylaxis 3 to 4 days post aneurysm coiling or clipping. If it is a nonaneurysmal SAH, those patients are not started on pharmacological DVT prophylaxis due to risk of rebleeding. They are, however, given mechanical DVT prophylaxis, and early mobility is encouraged.
DVT prophylaxis in patients with stroke: low-molecular-weight heparin (LMWH): Despite a small but definite risk of major hemorrhage, LMWH is the preferred medication for the prevention of DVT in patients with ischemic stroke. There is evidence that enoxaparin, the most commonly used LMWH, when compared to unfractionated heparin, is more effective at reducing the risk of DVT and PE in stroke patients (Sherman et al., 2007).
Unfractionated heparin (UFH): Patients who, due to renal dysfunction or other medical reasons, cannot receive LMWH should receive UFH for DVT prophylaxis. Usually, this is defined as a 5,000 unit dose administered every 8 hours for the general medicine patient. However, for the patient with increased risk of bleeding, as in the case of patients with large ischemic stroke or those with SAH or ICH, the recommendation is an adjusted dose of 5,000 units every 12 hours. UFH should be used only if LMWH is contraindicated in that patient.
Graduated compression stockings: The routine use of stockings alone as prophylaxis for DVT is not adequate and should be avoided. There is evidence that full-length elastic stockings do not significantly reduce the risk of DVT, as compared with no stockings (CLOTS Trials Collaboration, 2009). Both knee-high stockings and thigh-high stockings are associated with an increase in the risk of skin breakdown. This is especially problematic in stroke patients who often have communication and cognitive deficits from their stroke which prevent them from reliably being able to report discomfort or skin breakdown.
Sequential compression devices: For patients who cannot receive pharmacological prophylaxis for DVT due to risk of bleeding, as is the case with ICH patients in the first few days post hemorrhage, use of mechanical methods of DVT prophylaxis is recommended. The method with the least skin irritation remains the sequential compression device. There is evidence that intermittent compression prevents DVT and prevents venous stasis (CLOTS Trials Collaboration, 2013). The major disadvantage of relying on a mechanical method for prophylaxis over pharmacological prophylaxis is that of proper application of the device, making sure the device is turned on, and compliance.
Treatment of DVT in stroke patients: Treatment of DVT requires initiation of anticoagulation. The choice of anticoagulant is largely based on clinician choice. The duration of treatment depends on multiple factors, including whether the DVT was provoked or not, whether this is a first time DVT versus recurrence, and whether genetic predisposition to hypercoagulability exists.
Acute treatment: Prior to the approval of the thrombin inhibitor, rivaroxaban, for DVT treatment by the FDA, treatment of DVT could only be accomplished with warfarin, which takes several days to achieve its desired therapeutic effect. For this reason, oral anticoagulant therapy alone without bridge therapy with either continuous IV heparin or therapeutic dosing with subcutaneous enoxaparin or another LMWH was unacceptable. Now, if patients are being treated with rivaroxaban, there is no need for bridge therapy because therapeutic anticoagulation is achieved with the initial dose. The advantage of rivaroxaban is that it does not require routine clinic visits or blood work for monitoring because dosing is standardized. The disadvantage is that it currently has no generic version and is expensive. Warfarin, on the other hand, is very affordable, although its use is frequently cumbersome for the patient. Dosing of warfarin is highly individualized because it interacts with many foods and medications and thus requires regular international normalized ratio (INR) monitoring of the blood to maintain a narrow therapeutic range and avoid either under or over anticoagulation.
For bridge therapy while initiating warfarin, most clinicians initiate treatment with LMWH rather than UFH. The ease of administration and efficacy of LMWH makes this the preferred anticoagulant because there is no need for titration or checking activated partial thromboplastin times (APTTs) as with UFH infusion. UFH infusion requires continued hospitalization until the patient achieves therapeutic anticoagulation with warfarin, whereas patients using LMWH can be discharged once they or a family member has received appropriate instruction and demonstrated the ability to safely and successfully administer the required subcutaneous injections. Appropriate follow-up for INR monitoring and medication adjustment should be arranged by the care team prior to patient discharge. Although LMWH is associated with a lower risk of hemorrhage in medical patients, data comparing LMWH and UFH in stroke patients are insufficient to draw conclusions about their relative safety in this context especially in relation to hemorrhagic conversion of infarct. However, most diagnoses of clinical venous thromboembolism are made several days after stroke onset, by which time, the risk of hemorrhagic stroke transformation is lower.
The recommendation is to administer weight-adjusted LMWH divided into two doses for 5 to 7 days as initial treatment while bridging to Coumadin therapy. Because LMWH is predominantly excreted by the renal route, it should not be used in patients with significant renal dysfunction for risk of drug accumulation resulting in hemorrhagic complications. For patients with history of heparin-induced thrombocytopenia (HIT), an acceptable agent is the synthetic pentasaccharide fondaparinux, which is at least as effective and safe as LMWH in the treatment of DVT.
For patients at high risk of bleeding or renal dysfunction, a continuous infusion of UFH may be more appropriate given that UFH, in the low doses delivered via continuous infusion, is eliminated from the bloodstream via a saturable mechanism in which it binds to the reticuloendothelial system and endothelium (Boneu, Caranobe, & Sie, 1990). The other advantage is that it has a shorter half-life compared to LMWH or fondaparinux. Some stroke patients who require anticoagulation for DVT may still potentially require placement of a tracheostomy or percutaneous gastric feeding tube or may still have an ICP monitoring device in place which will require removal; the ability to quickly and easily suspend anticoagulation is advantageous for them. The main disadvantage of using heparin infusion in stroke patients who are not expected to require surgical interventions or invasive diagnostic testing is that it prolongs hospitalization, resulting in increased patient costs. Using LMWH and fondaparinux injections while bridging to Coumadin therapy reduces the length of stay in the hospital because these patients can be managed in either rehabilitation facilities or the outpatient setting.
Long-Term Treatment of Deep Vein Thrombosis
First proximal DVT occurs in the context of a transient risk factor (e.g., surgery or trauma): In this situation, the risk of recurrence is very low and a limited duration of therapy of 3 months is adequate (Levine et al., 1995).
First DVT occurs in the context of active malignant disease: Malignancy, especially if newly diagnosed, is an ongoing risk factor. Patients with malignancy have a higher incidence of recurrent thrombosis and bleeding complications while receiving oral anticoagulation therapy (Prandoni et al., 2002). This is likely due to the prothrombotic state associated with cancer and to the difficulty of managing oral anticoagulant therapy with concomitant chemotherapy drugs, unpredictable oral intake, and liver dysfunction. Studies have shown that long-term anticoagulation therapy with LMWH is more effective than warfarin for preventing recurrent venous thrombosis without a statistically significant increase in bleeding risk in cancer patients (Meyer et al., 2002). It is recommended that all patients who have active malignant disease be treated with LMWH for at least 6 months if they have adequate renal function. Not only will it lead to lower risks of recurrent thrombosis but also it facilitates the management of patients who need to undergo multiple procedures (e.g., biopsy, line insertion) and who have periodic thrombocytopenia due to chemotherapy. Because the risk of DVT recurrence is higher among patients with cancer than among those without cancer, anticoagulation is recommended as long as the cancer is felt to be active. It is recommended to wait 6 months after cure or complete remission before discontinuing therapy (Prandoni et al., 2002).
First DVT occurs in the context of a thrombophilic defect: These defects include factor V Leiden; prothrombin gene mutation; deficiencies in protein C, protein S, and antithrombin; increased factor VIII levels; hyperhomocysteinemia; and elevated antiphospholipid antibody levels. Patients with persistently elevated antiphospholipid antibody levels have a higher relative risk of recurrence after stopping anticoagulation therapy for a first DVT than those without this thrombophilia. It has been reported that patients with an elevated factor VIII level have a 2-year risk of recurrence of 37% after stopping anticoagulant agents, compared with 5% among those with normal levels (De Stefano et al., 1999). However, many of the studies that looked at this as a risk factor included calf vein thromboses, which have a lower risk of recurrence. Currently, with the exception of patients with elevated antiphospholipid antibody levels or those with homozygous genetic defects, it is not routinely recommended to offer prolonged anticoagulation therapy after a first idiopathic DVT (Kearon, 2004). However, it is recommended to place the patient on aspirin for secondary stroke prevention if the patient has had a stroke and not placed on prolonged anticoagulation for other reasons such as atrial fibrillation.
Recurrent DVT: After a second recurrence of DVT, the risk of further thromboembolic events following the discontinuation of anticoagulation therapy is believed to be excessive if only 6 months of oral anticoagulation therapy is administered. Therefore, it is generally recommended that anticoagulation therapy be continued. However, no study has investigated the risk of recurrent DVT if both events occurred during a transient risk period. In this situation, a shorter duration of anticoagulation therapy may be adequate (3 to 6 months), but other factors may influence this decision. When considering prolonging anticoagulation therapy, the risks of bleeding must be individualized and weighed against the potential benefits of preventing recurrence of thrombosis.
Best Practices
Currently, there are no recommendations for routine surveillance for DVT in stroke patients. Currently, the focus is on prevention of DVT unless a patient becomes symptomatic, then further testing is warranted at that time to confirm diagnosis of DVT. Best practices in prevention of DVT are a combination of nonpharmacological and pharmacological therapies. These include early mobilization, hydration, intermittent pneumatic compression, UFH, or LMWH.
Avoiding dehydration post stroke is vital. Dehydration after ischemic stroke is independently associated with DVT (J. Kelly et al., 2004). In the context of DVT prophylaxis, fluid intake has not been evaluated in a clinical trial, but current guidelines recommend keeping patients well hydrated in the early stage of ischemic stroke. This is especially important for those patients with dysphagia or with communication deficits which affect their ability to ask for oral fluids or taking in enough oral fluids. Extremity swelling, especially in the hemiplegic extremity or in the extremity with a central catheter, should warrant further investigation for DVT in the hospitalized stroke patient.
Evaluation
The preferred method for reevaluation of resolution of DVT is currently Doppler ultrasound because it is widely available and affordable. Patients need reimaging in 3 to 6 months to evaluate resolution of DVT if the plan is to discontinue anticoagulation. However, if the patient is asymptomatic and requires long-term anticoagulation, there is no need for reimaging. For those patients needing long-term anticoagulation, bleeding risk can be assessed during yearly visits, which will enable a risk–benefit evaluation to determine if anticoagulation therapy should continue.
For patients with thrombophilic defects, it is important to refer them to a hematology specialist to ensure that a comprehensive hypercoagulability workup is completed because this determines long-term anticoagulation decision. Hypercoagulability studies completed in the acute setting may need to be repeated in the outpatient setting to ensure that inflammatory responses in the acute setting were not responsible for any positive results. Hypercoagulability testing tends to be expensive so the interprofessional care team uses careful judgment in deciding which patients should have this blood work initiated in the acute setting. If any of the test results raise concern for hypercoagulability, a referral to a hematologist is beneficial so that a decision regarding long-term anticoagulation can be made as well as determining the use of testing other family members to better assess their risk of stroke or other thrombotic events.
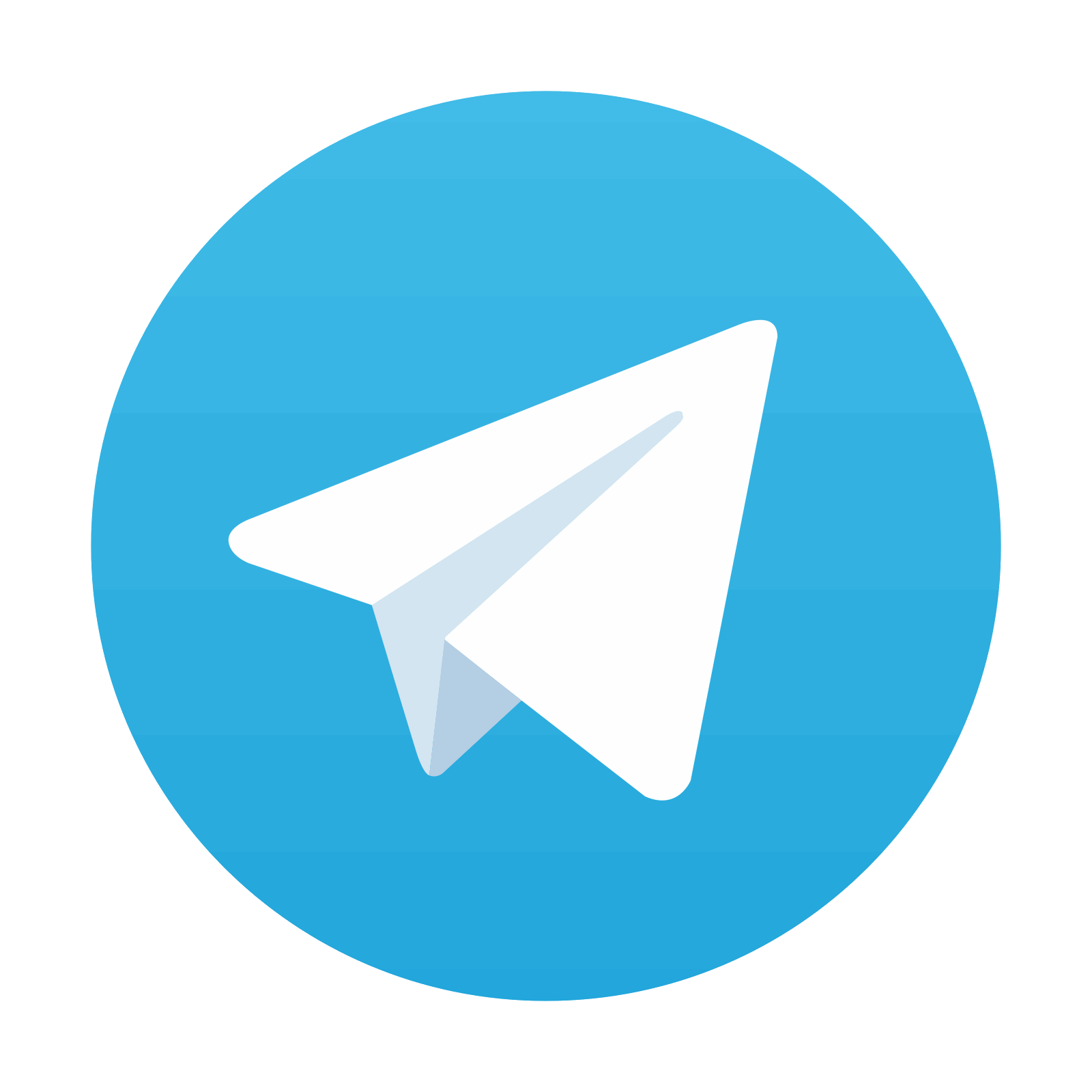
Stay updated, free articles. Join our Telegram channel

Full access? Get Clinical Tree
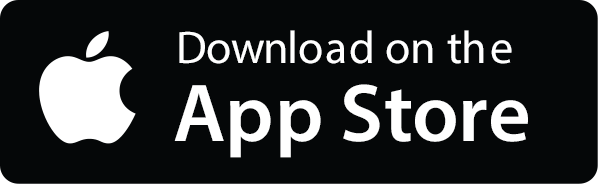
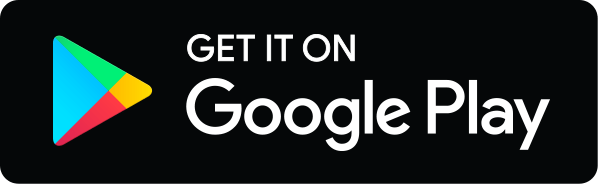