CHAPTER 20 Maintaining fluid, electrolyte and acid–base balance
Introduction
This chapter will provide some of the essential knowledge necessary to achieving these goals.
Fluid in the body
Fluid compartments
Body water is distributed between two major compartments: approximately two thirds as intracellular fluid (ICF) and one third extracellular fluid (ECF) (Figure 20.1). The volume of ICF is around 28 L in a 70 kg male. The ECF has a volume of approximately 12 L. It comprises the intravascular fluid (about 2.5 L within the heart and vessels) and the interstitial fluid (about 9.5 L) surrounding the cells and forming a relatively constant environment. In addition, there are very small quantities of transcellular fluid (e.g. within the eye, cerebrospinal fluid, glandular secretions) and water in bone.
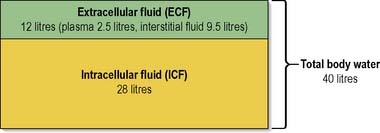
Figure 20.1 Distribution of body water in a 70 kg person.
(Reproduced from Waugh & Grant 2006 with permission.)
Electrolytes in the body
Electrolytes are essential for cellular activity and need to remain within a specific range for optimal function. In clinical practice, serum electrolytes are measured by a simple blood test, urea and electrolytes, often known as ‘U&Es’. Nurses should be aware of normal ranges and factors which may cause electrolyte imbalances in patients for whom they are caring, for example patients with cardiovascular disease or renal disease or those receiving diuretic medication. This knowledge will assist nurses and other health professionals to respond with appropriate care interventions. Box 20.1 provides a more detailed description of the common electrolytes.
Box 20.1 Information
(adapted from Waugh 2003 with permission)
Common electrolytes: functions and features
Sodium (Na+) – normal range 135–143 mmol/L
Potassium (K+) – normal range 3.3–4.7 mmol/L
Water and electrolyte homeostasis
Maintaining fluid and electrolyte balance
Maintaining normal, healthy fluid intake and the constancy of the internal environment is essential for efficient cell function. Fluid balance in the body is maintained by a number of physiological mechanisms. These mechanisms help to ensure that water gain and water loss are balanced (Table 20.1) and prevent the consequences of imbalances such as dehydration and possible death. The main stimulus for increasing intake of fluid is the thirst mechanism.
Table 20.1 Water balance – water gain and water loss (adult)
Input – Water Gain | Output – Water Loss |
---|---|
Beverages 1500 mL Food 750 mL Water produced during metabolism 250 mL | Urine 1500 mL Faeces 100 mL Sweat 200 mL Skin and respiration 700 mL (insensible loss) |
Total 2500 mL | Total 2500 mL |
Fluid and electrolyte balance is inextricably linked and in health the volume and composition of the different fluid compartments are finely regulated, with daily fluctuations in TBW of less than 0.2%. Water and electrolytes are ingested and absorbed through the gastrointestinal (GI) tract, although a small volume of water is produced through the oxidation of hydrogen in food. Excess water, electrolytes and waste products are excreted via the kidneys and in faeces. Additional water and salt loss occurs via the skin and respiratory tract, known as insensible loss. Since losses from the gastrointestinal and respiratory tract are not subject to fine regulation, the kidney is the main regulator of fluid and electrolyte balance (see Ch. 8). The flow of blood through the kidney is known as the glomerular filtration rate (GFR) and a normal GFR is 125 mL/min. In 24 hours, the total blood flow through the kidneys is approximately 180 L; this means that the plasma contained within the body is filtered and reabsorbed about 60 times a day. The kidney regulates not only fluid volume, but also electrolyte composition, pH and osmolality. Central to the renal regulation of body fluid osmolality and volume is the kidney’s role in the handling of sodium and water.
The osmotic pressure of a solution is expressed as the osmolality (the number of particles present per kilogram of solvent). Osmolality is a standard measure used in medicine and in the very simplest of terms could be considered a measure of the concentration of a solution. Each of the constituents within the ECF and plasma, e.g. the principal ions (sodium, potassium, chloride, etc.) and other non-ionic substances such as glucose and amino acids, exerts a different osmotic pressure. The total sum of these is the measure of the osmolality of the ECF and plasma within 1 kg of the principal solvent within the body, water. The ECF and plasma are said to have an osmolality of around 300 mOsm/kg. The osmotic pressure produced by the proteins, although small, plays an important role in the exchange of fluid between body compartments (Pocock & Richards 2006).
Disturbances in fluid exchange
As approximately 20% of body fluid is found in the interstitial or tissue spaces, the maintenance of fluid volume in this compartment plays a key role in homeostasis. Normally, a dynamic equilibrium exists which maintains the extracellular fluid content of both the plasma and the tissue spaces. However, this delicate balance can easily be disturbed by the following factors and the resulting clinical picture for many of them will be oedema (Box 20.2). Further information about oedema is available on the website.
See website for further content
Regulation and adjustments of ECF volume, osmolality and sodium
The circulatory system comprises the arterial system of high pressure and low volume and the venous system which operates with a lower pressure and higher volume. Approximately 55% of the plasma volume is in the venous system, 10% in the arterial system and the remaining 35% distributed in the heart, lungs and capillaries (see Ch. 2). Thus changes in volume are usually accommodated by the venous system. The effect of gravity upon fluid in the circulatory system is marked, causing pooling of blood in the venous system with a consequent reduction in the arterial blood volume. If an individual stands for a prolonged period, particularly in a warm environment, there is a reduction in arterial flow to the cells; inadequate venous return then leads to a fall in end-diastolic volume and hence cardiac output. Inadequate perfusion of the brain can then lead to fainting.
Influence of antidiuretic hormone
The presence of a non-absorbable solute in the renal tubular lumen will also increase water loss. For example, when plasma glucose levels are raised, as in poorly controlled diabetes mellitus (see Ch. 5), and filtered glucose exceeds the ability of the nephrons to reabsorb it, urine production is increased. The glucose exerts an osmotic force, keeping water in the tubule. Osmotic diuresis can also be induced therapeutically by the i.v. administration of a non-absorbable molecule such as the sugar mannitol.
Fluid and electrolyte disturbances
Assessment of fluid and electrolyte balance is covered later (pp. 585–589 including Tables 20.4 and 20.5 that outline some of the signs associated with fluid and electrolyte disturbances and measurable parameters and their significance).
Table 20.4 Observable/reportable signs of fluid and electrolyte disturbance and their possible significance
Clinical Signs/Symptoms | Clinical Significance | Cautions in Interpretation |
---|---|---|
Mucous membranes will appear dry and the patient will complain of thirst | Decreased saliva production will result in a dry mouth and sensation of thirst. Osmoreceptors detecting hypovolaemia will also trigger a feeling of thirst. This acts as a useful backup, as effective oral hygiene often disguises the decreased saliva production | Mouth breathing Oxygen administration Anticholinergic drugs, e.g. dicycloverine hydrochloride |
Tongue furrows | A normal tongue has one long longitudinal furrow, but in dehydration additional furrows will be present and the tongue will appear smaller due to fluid loss (Lapides et al 1965) | – |
Sunken eyes | The result of decreased intraocular pressure | – |
Increased jugular venous pressure (JVP). With a patient positioned at 45 degrees, venous distension should not exceed 2 cm above the sternal angle | Distended veins indicate fluid overload; flat veins indicate decreased plasma volume | Assessing the right internal jugular vein gives a more reliable reading than on the left as it is the most anatomically direct route to the right atrium |
Reduced capillary refill time | Capillary refill taking 2–3 s indicates a mild fluid deficit Refill times in excess of 3 s signify severe fluid deficits | Peripheral shutdown due to cold will slow capillary refill irrespective of fluid status |
Reduced skin turgor | Reduction in interstitial and intracellular fluid will reduce skin elasticity | In an older person it is difficult to detect changes in skin turgor due to the gradual loss of skin elasticity with age |
Cool peripheral temperature and pale skin colour | As a result of the renin–angiotensin cycle, hypovolaemia will result in peripheral vasoconstriction and therefore reduced temperature and colour | Patients with poor circulation, e.g. peripheral vascular disease or Raynaud’s disease, will normally have cool peripheries Certain antihypertensives including vasodilators and ACE inhibitors will disguise the body’s normal compensatory mechanism |
Dark urine | Hypovolaemia will trigger release of ADH, leading to more concentrated urine | Patients with liver disease may have bilirubin present in their urine giving it a very dark colour. Diuretic therapy will override the body’s production of ADH |
Peripheral oedema | Oedema occurs with the movement of fluid into interstitial spaces as a result of fluid excess and/or reduced levels of plasma proteins A consequent decrease in intravascular fluid may lead to a reduction in blood pressure | – |
Pulmonary oedema (fluid within the lung alveoli), observable through frothy, pink-stained sputum and/or dyspnoea (shortness of breath) | Pulmonary oedema will result in decreased gaseous exchange, with a reduction in oxygen saturation and arterial oxygen levels | – |
Table 20.5 Measurable parameters and their significance in fluid and electrolyte imbalance
Clinical Parameters/ Measurements | Clinical Significance | Cautions in Interpretation |
---|---|---|
Pulse | If there is a reduction in circulating volume and therefore stroke volume, the heart rate will increase to compensate and maintain cardiac output (see Ch. 2) Initial assessment of rhythm, based on the regularity of the pulse, may be useful and indicate a need for an ECG recording | Cardiac drugs, e.g. beta-blockers, such as atenolol, celiprolol, will inhibit the body’s compensatory mechanism and therefore block an increase in heart rate |
Blood pressure | Measurement of blood pressure will give an indication of circulating volume. Pulse pressure, the difference between systolic and diastolic pressure, will give an indication of vasoconstriction, i.e. compensation by the body | As a result of numerous compensatory mechanisms, blood pressure is maintained by the body for as long as possible. A ‘normal’ blood pressure in the presence of compensation must be acted upon immediately |
Central venous pressure (CVP) | Will be reduced as a result of hypovolaemia and/or vasodilatation An increase in CVP does not necessarily indicate fluid overload as CVP is influenced by numerous other factors including cardiac competence, systemic vascular resistance (SVR), intrathoracic and intra-abdominal pressure | For further information, see Chapter 18 |
Urine volume | In health, the body produces 1 mL urine/kg of body weight per h Acceptable urine output in the critically ill patient is equal to 0.5 mL urine/kg per h | A knowledge of the patient’s weight and calculation of desired urine output based upon that is essential Administration of diuretics will override normal physiological processes. Their use must be noted when assessing volume of urine produced |
Specific gravity (SG) of urine | Demonstrates the body’s ability to concentrate urine as an indicator of kidney function and/or response to ADH production | Administration of diuretics will override normal physiological processes. Their use must be noted when assessing urinary SG |
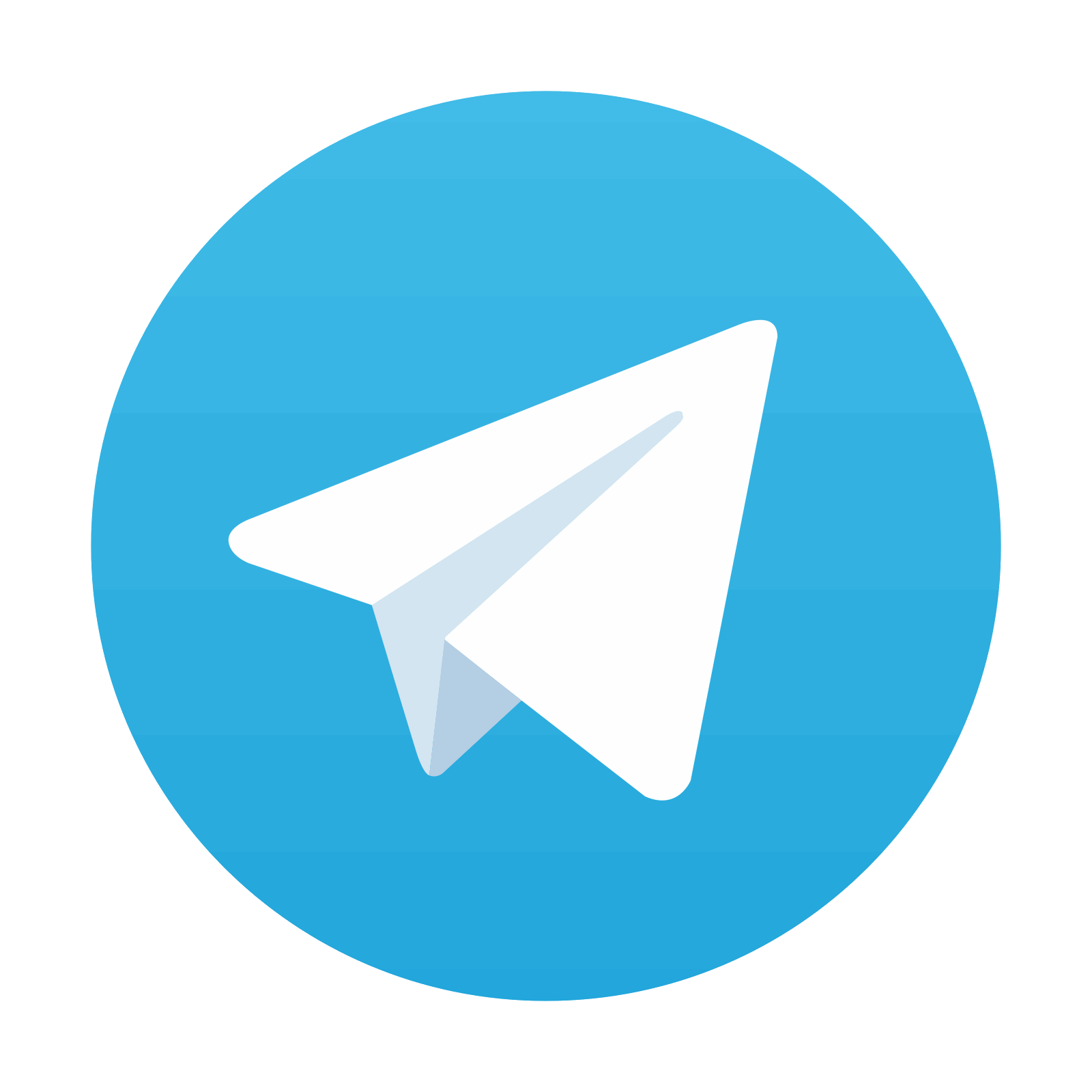
Stay updated, free articles. Join our Telegram channel

Full access? Get Clinical Tree
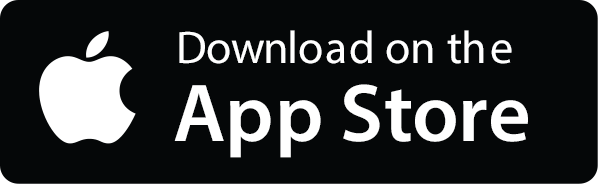
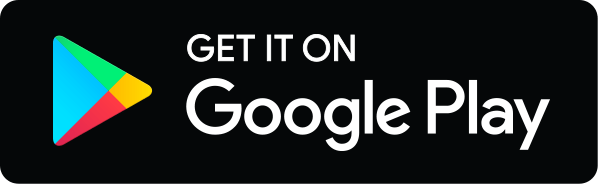