Chapter 10 Laboratory testing for HIV infection
Introduction
In the 30 years since HIV infection was first recognized it has spurred the development of a number of laboratory tests to aid in the diagnosis and management of the disease. The use of these tests has become well established in America and Europe and there are a number of up-to-date reviews of HIV laboratory testing available for these areas [1]. There is less experience with using laboratory testing in the developing world where HIV subtypes may be different, host responses to the virus may vary, and technical and financial resources may be limited. Much experience has been gained since 2005 with the expansion of testing and treatment programs in sub-Saharan Africa and Asia. In this chapter, laboratory tests for the diagnosis and management of HIV infection are described with an emphasis on those in use or applicable to resource-limited settings.
Initially, tests for the diagnosis of HIV infection, including tests of body fluids other than blood, and tests for incident versus prevalent infections, are described. Next, tests that measure the robustness of the immune system, including relatively simple tests for measuring CD4 T cells are described. Tests that measure the amount of virus present in an individual for diagnosis, prognosis, and monitoring of therapy are then presented. Finally, an overview of tests that detect drug resistance mutations in HIV are also described. A brief additional section on two tests (HLA-B5701 testing and HIV tropism testing) has been included. None of these tests are valuable without accurate quality control procedures for validating the results being generated. Pricing1 for each of the test kits are shown when possible but do not reflect technician costs, which vary greatly by country and by region. Significant price reductions for some reagents have been negotiated by the World Health Organization (WHO) and the Clinton Foundation (http://www.clintonfoundation.org) in order to make these tests more affordable.
Diagnostic Testing
During, or shortly after, the clinical presentation of acute retroviral infection, IgM antibodies to Gag (p17, p24, p55) and Env (gp41,120,160) first appear [2]. This response seems similar throughout the world, regardless of HIV subtype or host population [3]. The appearance of the IgM response is followed weeks to months later by IgG antibodies to Gag and Env epitopes and later still to viral enzymes and regulatory proteins. The time to first detectable IgG seroconversion by enzyme immunoassay in most studies in Europe and America is 2 weeks with a median of 3–4 weeks [2]. Almost all newly HIV-infected individuals will have detectable IgG by 6 months.
Currently the most established tests for detecting HIV infection rely on an enzyme-linked immunoabsorbent assay (ELISA) as an initial screening test (cost US$1–1.50 per test). This method involves coating a solid surface such as the plastic of a 96 well plate with antigen and using this as an affinity matrix for IgG present in a serum sample. First-generation ELISA used crude lysates of HIV as antigen, followed by second- and third-generation tests using increasingly refined recombinant preparations of HIV proteins to coat the plastic. Current fourth-generation tests use a combination of IgG antibody capture by the immobilized recombinant antigen and HIV antigen capture by immobilized antibody simultaneously in the same reaction to increase sensitivity [4]. The consequent reduction in the “window period,” particularly in developing world areas where incident infection is frequent, is important for reducing the number of false-negative results.
The mechanics of ELISA lend themselves to high throughput, rapid testing with automation and thus is the preferred screening test in the developed world. The US FDA and the WHO have validated a number of these assays for use worldwide (see http://www.fda.gov/cber/products/testkits/htm; http://www.who.int/diagnostics_laboratory/evaluations/hiv/en). The ELISA has also been evaluated in less developed countries with a variety of HIV subtypes and similar results for accuracy have been obtained [3, 5]. The cost–benefit analysis of frequency of HIV testing will depend on the background risk of the population and the background incidence in the population but in developed countries testing is rarely recommended more frequently than annually.
Despite high specificity, the use of ELISA in populations where the prevalence of disease is low will lead to a high proportion of positive results being false. Thus, the preferred testing strategy in the developed world is to confirm positive or indeterminate (an intermediate colorimetric reaction) results in the ELISA with a second test, Western blot. This uses a similar concept to ELISA but the immobilized antigens are first separated by size through SDS-PAGE electrophoresis and then bound to a solid medium. Antibody reactivity to different-sized proteins of HIV can be determined. The criteria for a Western blot positive test vary depending on the manufacturer of the test kit and the fluid being tested. In general, sera with antibodies reactive to gp120 and gp160 plus either gp41 or p24 must be present to confirm a positive ELISA [2]. Western blots require significant time and resources and are costly (US$11 per test), and therefore are not suited to many parts of the world where the prevalence of HIV is high.
A test that has worked well in resource-poor areas is the rapid HIV test. There are several of these approved for use by both the FDA and the WHO. The basic method requires spotting of whole blood or serum/plasma on a test strip with HIV antigen prebound (http://www.cdc.gov/hiv/rapid_testing). Lateral chromatography of the antibodies within the test strip and reaction with the immobilized HIV proteins is revealed colorimetrically in a “pregnancy test” fashion within minutes. These tests have higher than 99% sensitivity and specificity when combined with a confirmatory test such as Western blot in the developed world or a second rapid test in the developing world [6]. The lack of sample preparation, ease of storage, simple visual readout, speed, and cost (US$0.47–1.30) make these tests attractive for use all over the world and they have been evaluated in a number of African countries where their sensitivity and specificity profiles have been comparable with results in Europe and America [5, 7, 8]. A number of algorithms (Fig. 10.1) have been developed for confirmation of rapid testing results without resorting to ELISA or Western blotting and these have been validated in different resource-poor settings [9].
Other screening strategies for HIV infection have tested for the presence of antibodies in fluids other than blood. Levels of IgG are much lower in urine than in plasma but a highly sensitive screening test for urine, similar to ELISA, has a reported sensitivity of 99% and a specificity of 94% and is FDA approved [10]. A positive result should be confirmed with a standard serological ELISA. Saliva contains much higher concentrations of IgA and IgG than blood and there is an FDA-approved collection method involving soaking a pad in the mouth and then testing the adsorbed antibodies in a standard ELISA/Western blot system, which is extremely sensitive and specific [11]. An ELISA test for IgG in cervico-vaginal secretions is available. The principal benefit of these approaches lies in their convenience for the patient and the lessened risk of needlestick injury to the tester, but their reliance on ELISA for screening or confirmation make them less adaptable to the developing world.
Vertical transmission of HIV presents a particular diagnostic challenge as placentally transfered maternal antibodies can persist in the neonate for as long as 18 months postpartum [12]. Nucleic acid testing (DNA or RNA) provides the most sensitive method for diagnosing HIV in an infant. At 4–6 weeks, most infants can be diagnosed using these methods.
For epidemiological purposes, it is important to measure the incidence of HIV infection in populations. Recently, chronically infected individuals can be differentiated by biological changes that occur as the immune system responds over time to the infection [13]. The evolution of the immune response is shown in Figure 10.2. There is a window period of approximately 3 weeks, where HIV RNA can be detected but an antibody response to the virus is not detectable. During the initial development of the humoral response, the strength of binding and concentration of antibody specific for HIV increases with time. There is also a switching of the class of antibody to the virus. An epidemiological tool for determining incidence rates from cross-sectional population sampling exploit these biologic differences between incident and chronically infected individuals. Currently the only commercially available assay is the BED-capture EIA (BED-CEIA), though many groups have developed their own assays [14]. Polymerase chain reaction (PCR) of pooled blood samples for detecting viral RNA positive samples before antibody has developed has been pioneered in North Carolina, USA, and may be the most sensitive and specific method for detecting incident infection in at-risk populations, but requires surveys in excess of 50,000 individuals [15]. It has not been tested in resource-poor areas but may be rapidly adaptable to Eastern Europe or China where PCR technology is already available. For all cross-sectional incidence approaches, sample sizes in the thousands are needed with an accurate knowledge of factors associated with misclassification.
Laboratory diagnosis of HIV-2 is frequently necessary depending on geographical area, travel, and exposure history. Infection with HIV-2 is prevalent in West Africa and in many cases, HIV-1-infected people are also infected with HIV-2, although it is rare in the rest of the world. The virus is less transmissible, replicates more slowly, and causes a slower rate of T cell decline and there are few standardized tests useful for management. The diagnostic testing methodology for HIV-2 is identical to that for HIV-1, except that the serological tests are specific for antibodies against HIV-2 proteins. Currently there is one commercially available assay for HIV-2 Western blot testing (HIVBlot 2.2-Genelabs) but no viral load test. However, HIV-2 viral load data can be generated by real-time RT-PCR using primers specific for HIV-2 [16]. There are rapid test kits and diagnostic ELISA tests available specifically for HIV-2 and one test available for diagnosis of both HIV-1 and HIV-2 simultaneously [17,18], although 20–30% of HIV-1 ELISA tests do not detect antibodies to HIV-2 [1].
Monitoring Patient Health
Tests for estimating T lymphocytes
Monitoring of the health of the immune system is accomplished with estimates of the number of CD4 T cells circulating in a person’s bloodstream. This is the most reliable prognostic test available [19] and is the primary means of deciding need for anti-viral treatment. Tests of CD8 T cells are valuable for measuring anti-HIV cytolytic activity as a research tool but their number has not been found to correlate with clinical outcome.
The value of a CD4 determination at any particular time point is approximate, with wide confidence intervals. Thus, the utility of testing is mainly in observing trends. The recommended interval of testing is 3–6 months (see DHHS, WHO guidelines) although some national treatment programs, notably Malawi, have scaled up without widespread CD4 testing. A result markedly different from prior results should be repeated. In addition, many factors other than HIV-1 influence the CD4 count, including diurnal variation, intercurrent illness, corticosteroid use, idiopathic lymphocytopenia, and splenectomy [1]. Of significance in some parts of the world, most notably Brazil and Haiti, is the interaction between HIV-1 and HTLV-1 co-infection. In these patients the true level of immunosuppression is more accurately represented by a CD4 T cell value half as high as that actually measured [20]. The CD4 T cell fraction of total lymphocytes, expressed as a CD4 percentage, is sometimes used to account for variation in the total number of T cells but this has not been found to correlate as accurately with risk for opportunistic infection as the absolute count [21]. The total lymphocyte count has been used with some success in resource-poor areas as a surrogate for T-cell counting, although the sensitivity of this strategy was found to be low in a cohort in Uganda [22].
The standard method in America and Europe for measuring CD4 T cells is by flow cytometry. This requires a fresh blood sample and antibody reagents specific for CD4 and CD8 that are labeled with fluorescent molecules. The number of labeled cells present is counted by flow of individual cells past a fluorescence detector. This is expensive, both in equipment and in reagents (US$6–20 per test), and requires frequent calibration of the machine. A variation on standard flow cytometry for CD4 counting is leukogating. The primary advantage of leukogating is the ability to do the analysis in a single reaction with a lowered cost of reagents (<US$4 per test) but still requires an expensive flow cytometer for detection. For medium throughput applications, a microflow method that is more portable and uses smaller volumes has been developed (Guava Technologies). Lower throughput methods such as FACSCount (Becton-Dickinson) and PointCare (Beckman Coulter) give automated, absolute, or absolute plus percentage CD4 counts, respectively, making the PointCare machine more useful for pediatric monitoring. Alternative, low throughput (<10 samples per day) methods that have been developed specifically for resource-limited laboratories are Cyto-Spheres (Beckman Coulter) (US$4–8 per test) and Dynabeads (Dynal Biotech) (US$3–5 per test). The former uses latex beads coated with anti-CD4 monoclonal antibody to “rosette” CD4 lymphocytes that can then be counted in a hematocytometer by light microscopy. The latter uses magnetic beads that are coated with anti-CD4 antibody to purify CD4 lymphocytes from whole blood using a magnet. A newer test, the PIMA POC CD4 Counter (Clondial Gmbh), consists of a disposable test cartridge containing dried reagents. All of these “low-tech” methods correlate well with flow cytometry both in Europe and in the developing world [23].
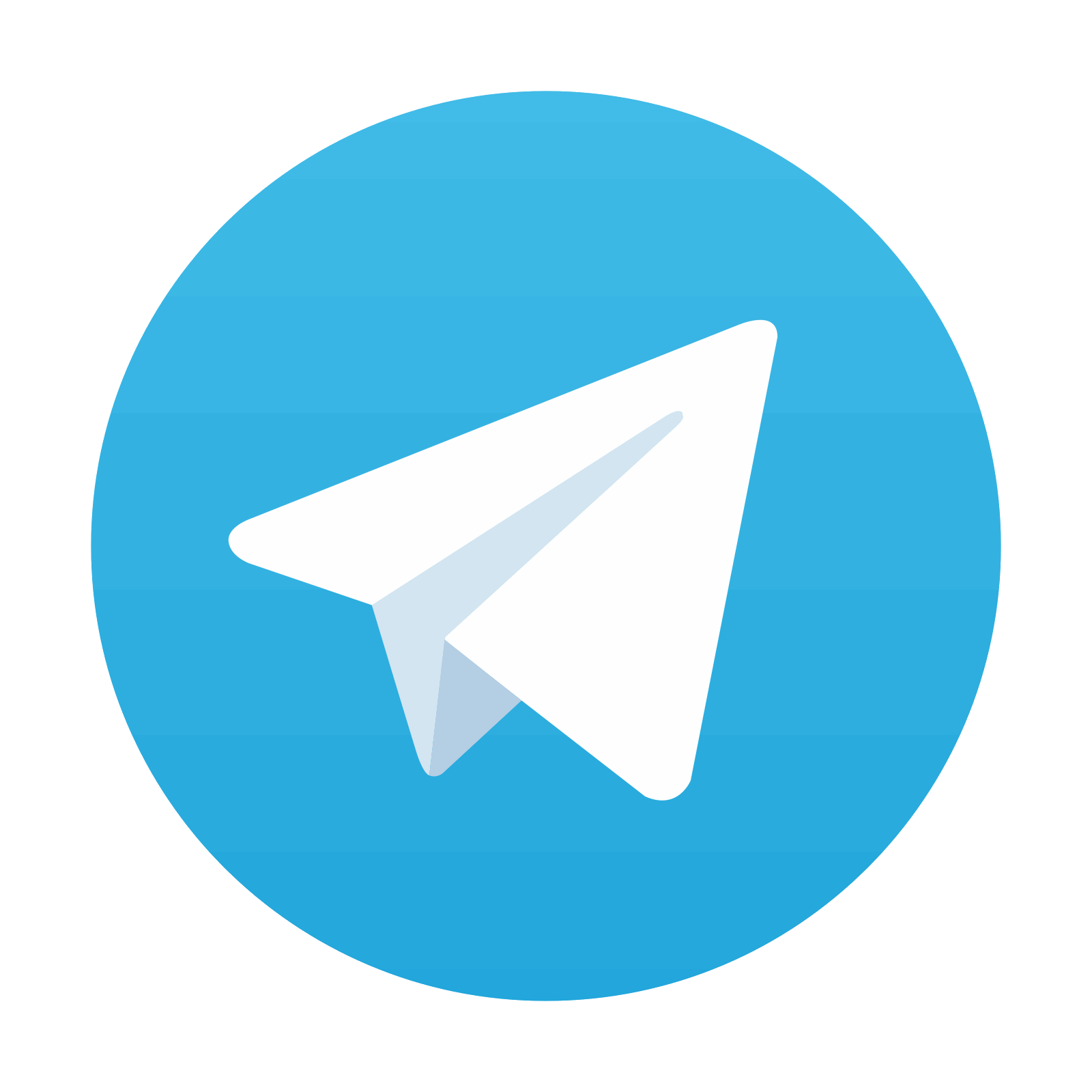
Stay updated, free articles. Join our Telegram channel

Full access? Get Clinical Tree
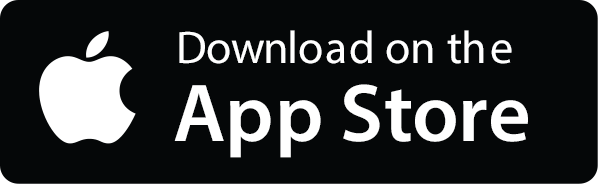
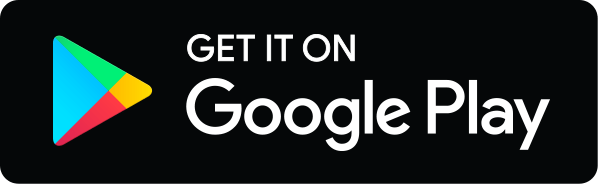