Current Knowledge of Acute Lung Injury and Acute Respiratory Distress Syndrome
Keywords
• Acute lung injury • Acute respiratory distress syndrome • Molecular biology • Cytokines • Molecular biology
Introduction
The first published report describing the clinical syndrome currently referred to as acute respiratory distress syndrome (ARDS) occurred in 1967.1 Since this landmark work by Ashbaugh and colleagues,1 numerous investigators have reported clinical trials and observations of adult patients who exhibited the clinical sequelae associated with this syndrome with varying outcomes. Current evidence suggests that the mortality associated with this syndrome has been decreasing while substantial morbidity persists.2–5
Acute lung injury (ALI) and ARDS involve a heterogeneous process in the lungs that results in diffuse alveolar damage. The current characteristics associated with ALI include bilateral infiltrates on chest radiograph, Pao2 to Fio2 ratio less than 300, no evidence of left ventricular failure evidenced by a pulmonary artery occlusive pressure less than 18 mm Hg or central venous pressure less than 14 mm Hg, and need for invasive mechanical ventilator support. ARDS is a subset of patients whose Pao2 to Fio2 ratio is less than or equal to 200. ALI (or ARDS) is associated with a variety of causative factors, which can be grouped into two general categories: those associated with direct lung injury via the airways and those associated with indirect lung injury via the blood stream (Table 1).6,7 More recently, investigators have focused on an extension of direct factors associated with iatrogenic lung injury induced by mechanical ventilation,8–12 currently referred to as ventilatory induced lung injury (VILI). VILI can be further stratified as lung injury attributable to volume (volutrauma) or pressure (barotrauma). This article discusses the pulmonary sequelae associated with ALI and ARDS, the support of ARDS with mechanical ventilation, available adjunctive therapies, and experimental therapies currently being tested.
Table 1 Causative factors associated with ALI and ARDS
Direct Pulmonary Insult | Indirect Pulmonary Insult |
• Pneumonia | • Sepsis |
• Aspiration (especially gastric content) | • Blood transfusions |
• Pulmonary contusion | • Nonthoracic trauma |
• Fat emboli | • Reperfusion injury from cardiopulmonary bypass |
• Near-drowning | • Acute pancreatitis |
• Pneumonitis (oxygenation, smoke inhalation, radiation, bleomycin) | • Drug overdose |
Pulmonary Sequelae Associated With ARDS
Exudative Phase
In this early presentation, the histology demonstrates marked diffuse alveolar damage, increased accumulation of neutrophils, vasodilation, endothelial cell damage, and pulmonary edema, secondary to increased vascular permeability13–16 as shown in Fig. 1.

Fig. 1 Physiologic alternations associated with onset of ARDS.
(Reprinted from Ware L, Matthay M. The acute respiratory distress syndrome. N Engl J Med 2000;342(18):1334–49; with permission.)
Analysis of bronchoalveolar lavage samples obtained from ARDS patients have identified numerous mediators present in this milieu, which include cytokines, oxygen radicals, activated complement, and leukotrienes (Table 2).17,18 These histologic changes produce the characteristic symptoms associated with the exudative phase of ARDS. Physiologically, hypoxemia results from interstitial and alveolar flooding that contributes to intrapulmonary shunting and atelectasis.19,20 The alveolar flooding also results in a decrease in pulmonary compliance.
Table 2 Proinflammatory mediators associated with the development of ARDS
Mediator Category | Mediators |
Tumor necrosis factors (TNF) | TNF-α, TNF-β |
Interleukins (IL) | IL-1β, IL-2, IL-6, IL-10, IL-12 |
Chemokines | IL-8, growth-regulated peptides, MIP-1, MCP-1 |
Colony-stimulating factors (CSF) | G-CSF, GM-CSF |
Interferon (IFN) | IFN-β |
Abbreviations: MCP-1, Monocyte Chemotactic Protein-1; MIP-1, Macrophage Inflammatory Protein-1.
The exudative phase of ARDS is believed to occur over 3 to 7 days from the onset of lung injury. From 33% to 50% of all deaths associated with ARDS occur during this early phase.21
Fibroproliferative Phase
Persistent ARDS leads to increased alveolar and parenchymal damage, pulmonary hypertension, and pulmonary fibrosis. After a few days of lung injury, proliferation of alveolar cells, endothelial cells, and fibroblasts occurs. If ARDS persists during this proliferative phase, alveolar scarring (fibrosis) and multiple organ failure may occur. The development of pulmonary fibrosis and multiple organ failure contributes to mortality during the late phase of ARDS. During this phase, activated lung fibroblasts secrete a procollagen that is cleaved to create a type III collagen, referred to as Pro-Collagen Peptide-III (PCP-III). Clark and colleagues demonstrated in 1995 that the presence of PCP-III in the BAL fluid of ARDS patients is significantly correlated with mortality, and PCP-III appears to reflect the development of pulmonary fibrosis.22 This finding was confirmed and further extended in subsequent investigation performed by Chesnutt and colleagues in 1997.23
Resolution Phase
For those who survive the exudative and fibroproliferative phases of ARDS, the final phase results in either death or recovery. The most recent large-scale, multicenter trial identified the mortality of ALI/ARDS at approximately 35%.24 Death in this population is commonly associated with the development of multisystem organ failure (MSOF). The sequencing of MSOF from the onset of ARDS has not been delineated, so the relative contribution to mortality for a given organ system remains unclear. This may be an area of important epidemiologic research for the future.
The successful resolution of ARDS may be related to some early changes in the histology of the alveoli.25 Neutrophils make up the majority of the cellular component of BAL fluid in patients with ALI/ARDS. As the lung injury resolves, neutrophils are replaced by alveolar macrophages. It is believed that these macrophages, although capable of inducing further lung injury, play an important role in the resolution of the injury. Fig. 2 demonstrates current understanding of the complex changes beginning with the proliferative processes and proceeding through resolution of the ALI. Important changes associated with this phase include epithelial repopulation, reabsorption of the alveolar fluid, clearing of the protein residue associated with the influx of the edema fluid observed in the exudative phase of lung injury, and finally resolution of fibrosis.6,7,26,27

Fig. 2 Mechanisms of resolution of ARDS.
(Reprinted from Ware L, Matthay M. The acute respiratory distress syndrome. N Engl J Med 2000;342(18):1334–49; with permission.)
The discovery of long-term effects on the pulmonary function of survivors associated with the onset and resolution of ALI has provided additional impetus to the systematic evaluation of quality-of-life (QOL) measures in this population. Previous investigators examined and reported the on QOL changes in survivors after hospital discharge.26–31 McHugh was the first to conduct and report such a systematic investigation.29 Similar to long-term changes in pulmonary function testing observed in ARDS survivors, there was a decrease in QOL immediately after extubation with a gradual improvement over time. The findings of abnormal QOL indices have been replicated by those of other researchers using a variety of instruments to measure QOL alterations.30–32 Hopkins identified significant neuropsychological deficits after resolution of ARDS.33 Davidson reported that ARDS patients had worse health-related QOL than the control groups.28 More recently, several investigators reported on QOL changes that extended beyond the original 2 years postdischarge in survivors of ARDS. Dowdy and colleagues reported in a meta-analysis in 2006 involving 13 studies and 557 patients substantial reductions in all domain scores (using the SF-36) except mental health and role physical domains.34 Similarly, Adhikari and colleagues reported in 2011 that depression symptoms and memory complaints persisted for up to 5 years after discharge in 64 survivors of ARDS.35 These persistent findings of altered QOL after hospital discharge in ARDS survivors have prompted the ARDS-USA Foundation to request that physicians caring for ARDS patients should make them aware of short-term and long-term alterations following hospital discharge.36 The health care burden has not been delineated but one would expect that as survivors increase, so will the burden on the health care providers.
Alveolar Mediators
A vast number of cellular mediators have been implicated in the pathogenesis of ARDS.25,37–42 Tables 2 and 3 list several mediators that have been associated with the development of ARDS. These mediators are believed to have an important role in the early inflammatory process through the recruitment and activation of neutrophils and mononuclear phagocytic cells. Of particular interest are various interleukins and tumor necrosis factor (TNF). Perhaps the most important of these are TNF-α, interleukin (IL)-1β, IL-8, and IL-10. Table 3 briefly outlines the role each of these mediators play in the development and resolution of ARDS. Interventional therapies have targeted the roles these mediators play in mitigation of the inflammatory process associated with ARDS.
Mechanical Ventilatory Support
Ventilator-Induced Lung Injury
Mechanical ventilation is the main therapy supporting gas exchange and acid–base balance in patients with respiratory failure due to ARDS. Although considered a life-saving therapy, mechanical ventilation can also cause lung injury that pathophysiologically mimics ARDS, a condition referred to as ventilator-induced lung injury or VILI. The ventilator-associated complications of oxygen toxicity43 and barotrauma44 have been long appreciated in the development of VILI; however, only recently have other mechanisms of injury been identified and potentially of more clinical importance. Lung injury can occur at the two opposite ends of the breathing pattern: when regional lung tissue is overstretched during inspiration (volutrauma),9,12,45 and when open lung units are repetitively allowed to collapse during expiration and re-expand on consecutive breaths (atelectrauma).45 Both types of injury can cause inflammatory mediator release into the blood stream and trigger end-organ failure (biotrauma), which may increase mortality.11,46–48 Limiting inspired volume and peak inspiratory pressure may be critical in minimizing volutrauma, while strategies that maintain patency of recruited lungs units, such as with positive end-expiratory pressure (PEEP), may be helpful in minimizing atelectrauma.
Lung Protective Ventilation Strategies
Low Tidal Volume and Pressure Limitation
The current standard of care for ventilatory management of patients with ALI/ARDS was described in a randomized controlled trial (RCT) by the NIH ARDS Network,24 which demonstrated a 22% relative (9% absolute) mortality reduction. This study suggested that volutrauma was minimized when tidal volume (VT) was targeted to 6 mL/kg of predicted body weight (PBW), and reduced to as low as 4 mL/kg PBW to maintain the end-inspiratory plateau pressure (Pplat) 30 cm H2O or less. Although the initial study was conducted using volume control ventilation, the current recommendation allows pressure ventilation as long as Vt is monitored and limited.
When Vt is reduced to maintain target Pplat, set respiratory rate should be increased, up to a maximum of 35 breaths/min, in an attempt to maintain a pH of 7.30 to 7.45. Increasing the set rate reduces the available time for exhalation, so care must be taken to minimize the creation of auto-PEEP.49 Administering a buffering agent might be necessary to maintain pH higher than 7.15 so Vt and Pplat can be kept within target, particularly when allowing permissive hypercapnia.
With the use of smaller volumes and faster rates, it is often perceived that patients may be uncomfortable and require more sedation than patients receiving larger Vts, but this perception has not been borne out in clinical studies.50 Interestingly, a recent study suggested that the use of a neuromuscular blocking agents (NMBAs) for the initial 48 hours of mechanical ventilation was associated with reduced mortality in patients with severe ARDS.51 Although the trend in recent years is to reduce the use of NMBAs to minimize the incidence of prolonged muscle weakness, judicious short-term use early in the process may improve patient-ventilator synchrony and reduce VILI.52
PEEP and Recruitment Maneuvers
Oxygenation is supported by ventilator adjustments in both PEEP and Fio2, to target a Pao2 of 55 to 80 mm Hg or Spo2 of 88% to 95%. PEEP is an anti-derecruitment pressure, helping to keep the airways and alveoli open that were inflated during the previous inspiration. By maintaining lung volumes at end-expiration, oxygenation is improved by reducing the ventilation–perfusion mismatch. It is generally accepted that a PEEP of less than 5 cm H2O is harmful to patients with ARDS because it allows significant atelectrauma. Pressures of 8 to 15 cm H2O are appropriate for most patients to minimize atelectrauma; however, higher levels may be required in patients demonstrating potential for lung recruitment.53 How to determine the best level of PEEP to apply is controversial.
There are two basic strategies for setting the PEEP level: either using a table for all patients or individualizing the level by assessing a change in gas exchange or respiratory mechanics. The Fio2/PEEP table concept was popularized by the ARDSnet studies, and many other RCTs have used this strategy in at least one arm of their study. Two Fio2/PEEP tables have been described, one using a lower PEEP level than the other for a given Fio2 (see Table 4). Both tables have been shown to be safe, but neither to be superior to the other.54 It has been recommended that the low PEEP table be used in patients demonstrating a low potential for lung recruitment, while the higher PEEP table be used for patients demonstrating a higher degree of potentially recruitable lung tissue.55
An individualized approach to setting PEEP is encompassed in the Open Lung concept, which is summarized in the saying, “open the lung and keep it open.”56 This approach involves four steps: (1) opening the lung by increasing inflation pressure to a level above the critical opening pressure of a significant number of alveoli, (2) gradually reducing PEEP from a modestly high level (approximately 20–25 cm H2O) until the critical closing pressure is reached indicating lung derecruitment, (3) reapplying the pressure used in step one to reopen collapsed lung units, and (4) applying a PEEP level slightly above that associated with the critical closing level.57 Other individualized approaches include obtaining information about respiratory mechanics from a pressure–volume loop during a slow inflation58,59 and deflation60,61 or via the pressure–time graphic scalar,62,63 but both are influenced by increased abdominal and pleural pressure, not just lung pathology.64 Measuring esophageal pressure to calculate the actual transpulmonary pressure (Ptp), or the pressure used to stretch the lung, is attractive. Ptp reflects the pressure difference between the pressure inside versus outside of the lung. In addition to setting PEEP in this manner,65 calculating Ptp at end-inspiration may inform us when high plateau pressure is safe or harmful.66–68
Three large multicenter RCTs have compared lower levels of PEEP to higher levels of PEEP: the ARDSnet ALVEOLI study,54 the Canadian/Australian LOVS trial,69 and the French EXPRESS trial.70 Each used high versus low PEEP strategy combined with a low-Vt strategy. The ARDSnet and Canadian studies used the same PEEP/Fio2 table. The Canadian trial also allowed Pplat up to 40 cm H2O and the application of intermittent increased inflation pressures (recruitment maneuvers) in the high-PEEP group. The French trial used a similar low-PEEP table, but individualized high-PEEP by increasing PEEP to attain a Pplat of 28 to 30 cm H2O. None of these studies reported a mortality difference, and the ARDSnet and French studies were stopped early for futility. A meta-analysis by Briel and colleagues analyzed the data of the 2299 patients from these three trials.71 For all patients, there was no difference in hospital mortality, although higher PEEP was associated with reduced intensive care unit (ICU) mortality, total rescue therapies (ie, inhaled nitric oxide [NO], prone positioning, high-frequency oscillatory ventilation, extracorporal membrane oxygenation), and death after rescue therapy. When stratified by the presence of ARDS or ALI at baseline, there was also a reduced hospital mortality (34.4% vs 39.1%) and more ventilator-free days at day 28 (12 vs 7 d) in the higher PEEP group for patients with ARDS at baseline. There was no difference in the incidence of barotrauma or use of vasopressors. Interestingly, there was a trend toward improved mortality in the lower PEEP group with ALI at baseline.
Recruitment maneuvers (RMs), as previously described, are part of the Open Lung concept, but they are also used as a stand-alone strategy to improve oxygenation. The ideal RM method has not been identified. A recent systematic review of recruitment maneuvers included 40 studies totaling almost 1200 patients.72 The sustained inflation method (ie, continuous positive airway pressure [CPAP] of 35–50 cm H2O for 20–40 seconds) was used most often (45%), followed by pressure control using high inspiratory pressure and PEEP settings (23%), incremental PEEP (20%), and a high VT/sigh (10%). Oxygenation was significantly increased after a RM, but the duration of this effect was short lived. Adverse effects during the RM included hypotension (12%) and oxygen desaturation (8%), both of which were generally transient, returning to baseline shortly after the RM, and rarely caused the RM to be prematurely discontinued. Serious adverse events, such as barotrauma (1%) and arrhythmias (1%) were infrequent. The authors concluded that given the uncertain benefit of transient improvement in oxygenation, routine use of RMs could not be recommended or discouraged and they should be considered on an individualized basis in patients with a life-threatening hypoxemia.
It has been suggested that studies of recruitment maneuvers showing oxygenation improvement but short duration of benefit may not have set PEEP to an adequate level after the RM, and that those that showed no benefit used relatively low recruiting pressures or applied them late in the course of disease.73 Iannuzzi demonstrated that the pressure control method (peak pressure of 45 cm H2O with PEEP of 16 cm H2O for 2 minutes) compared to the sustained inflation method (CPAP 45 cm H2O for 40 seconds) improved oxygenation to a larger degree and was better tolerated hemodynamically.74 A recent study of 50 ARDS patients evaluated the timing of lung recruitment and hemodynamic effects of a RM using a constant pressure of 40 cm H2O for 40 seconds.75 More than 98% of the volume ultimately recruited was attained within 10 seconds of applying the pressure. Mean and systolic blood pressures were stable for the first 10 seconds but became significantly reduced (P<.01) from baseline at 20 and 30 seconds. The authors recommended that when using the constant pressure RM method, the duration should be limited to 10 seconds to maximize recruitment and minimize hemodynamic compromise.
Current evidence suggests that that RMs should not be routinely used on all ARDS patients unless severe hypoxemia persists. RM might be used as a rescue maneuver to overcome severe hypoxemia, to open the lung when setting PEEP, or after evidence of acute lung derecruitment such as a ventilator circuit disconnect.76
Airway Pressure Release Ventilation
Airway pressure release ventilation (APRV) was originally described in 1987.77,78 This mode of ventilation uses a breathing system that allows “unrestricted breathing” at two levels of CPAP. APRV is often described as “upside down IMV” in that intermittent mandatory ventilation allows spontaneous breathing at a low CPAP level that is intermittently raised to provide mechanical tidal inflation (ie, a mandatory breath). APRV encourages spontaneous breathing at a high CPAP level that is intermittently released to a lower CPAP level, allowing an increased exhaled volume to augment spontaneous minute ventilation.
There are two schools of thought on how to set the lower CPAP level and duration of release. One practice sets the low CPAP to 0 cm H2O and a release time short enough to create air-trapping (auto-PEEP).79 The other practice is to set the low CPAP to a more traditional PEEP level (eg, 10–15 cm H2O) and the release time sufficient to allow complete exhalation before transition to the high CPAP level (ie, no auto-PEEP).80 In both methods, a majority of the breathing cycle is at the high CPAP level (generally an I:E ratio of >4:1), resulting in a higher mean airway pressure (Pmean) compared to conventional pressure and volume ventilation modes. All potential spontaneous breathing occurs at the high CPAP level, as the release period is so short that it allows only exhaled volume and there is no time for spontaneous breathing.
Spontaneous breathing during APRV improves the ventilation–perfusion distribution in dependent lung regions, enhances venous return and improves cardiac output in the face of increased Pmean, and requires less sedation.81 Although APRV-like settings can be applied to a passive patient, it is essentially pressure-control inverse-ratio ventilation if the patient is not spontaneously breathing.
Nonrandomized studies in ALI/ARDS patients report APRV to be associated with a lower peak inspiratory pressure,82,83 less sedation and vasopressor use, improved oxygenation,84,85 and possibly improved mortality.85 Four RCTs of APRV have been reported. Multiple trauma patients at risk for ARDS were randomized to either APRV (n = 15) or pressure-control ventilation (PCV) (n = 15).80 This study is often referenced in support of APRV, as it was associated with shorter duration of mechanical ventilation and ICU stay, as well as less use of vasopressors and inotropes. A significant limitation to this study, however, was that the control group was paralyzed for the first 72 hours, which may have given a bias toward the APRV group who were allowed to spontaneously breathe. A study of ARDS patients randomized to APRV (n = 30) or synchronized intermittent mandatory ventilation (SIMV) (n = 28) found lower inspiratory pressures were required in the APRV group but no difference was observed in gas exchange, hemodynamics, or ventilator-free days at day 28.86 Computer-assisted tomography was performed on 37 ALI patients at baseline and 7 days after randomization to APRV or SIMV. It was found that the change in the amount of nonaerated lung tissue was similar (P = .65), suggesting that neither form of ventilation was superior.87 In addition, in a study of trauma patients randomized to APRV (n = 31) or SIMV with pressure support ventilation (n = 32) found no difference in ventilator days, ICU days, incidence of pneumothoraces or ventilator-associated pneumonia, percent of patients requiring a tracheostomy, percent of patients failing the assigned modality, or mortality rate.88
High-Frequency Oscillatory Ventilation
High-frequency oscillatory ventilation (HFOV) delivers very small Vts (0.1–3 mL/kg PBW) at rapid rates (180–900 cycles/min or 3–15 Hz).89 Conceptually it applies high level CPAP with a wiggle. Oxygenation is supported by adjusting Fio2 and Pmean, while ventilation in affected by increasing the drive pressure or reducing the rate of oscillation. Although counterintuitive, reducing the rate improves minute ventilation by allowing more time for the piston to move back and forth, generating a larger stroke or Vt.90
HFOV has been shown to aid in lung recruitment, improve oxygenation, and reduce histologic lung damage and inflammation in animals.91–94 Adult clinical case series suggest that HFOV is safe and at least as effective as conventional ventilation, but has generally been applied as a rescue therapy for refractory hypoxemia.95–103 Two RCTs compared HFOV to conventional ventilation in ARDS patients. In one study, 148 patients were randomized to HFOV (n = 75) or conventional ventilation (n = 73). They found early improvement in oxygenation with HFOV, but the difference was not sustained beyond 24 hours.104 Although there were no differences in any other measured parameter, there was a trend (P = .102) toward improved mortality in the HFOV group (37% vs 52%). A limitation of this study was that the control group did not receive what is now considered a lung protective ventilation strategy. In an RCT of ARDS patients randomized to HFOV (n = 37) and conventional ventilation (n = 24), no difference was found in survival without supplemental oxygen or requiring ventilator support, mortality, therapy failure, or crossover to the other ventilatory strategy.105 A post hoc analysis found a better treatment effect in patients with higher oxygenation requirement at baseline. Two RCTs of HFOV in conjunction with prone positioning in ARDS patients found that proning, regardless of ventilation method, improved oxygenation106 and that HFOV maintained the oxygenation gained from the prone position better than conventional ventilation when returned to the supine position.107
HFOV theoretically should provide the optimal protective ventilation pattern with very small Vts to minimize volutrauma, and a high Pmean for recruitment to minimize atelectrauma. To provide the most lung protective settings, it is suggested to use a high oscillation pressure amplitude (starting at 90 cm H2O) coupled with the fastest frequency tolerated (ideally >6 Hz) targeting a pH of 7.25 to 7.35.100,108,109
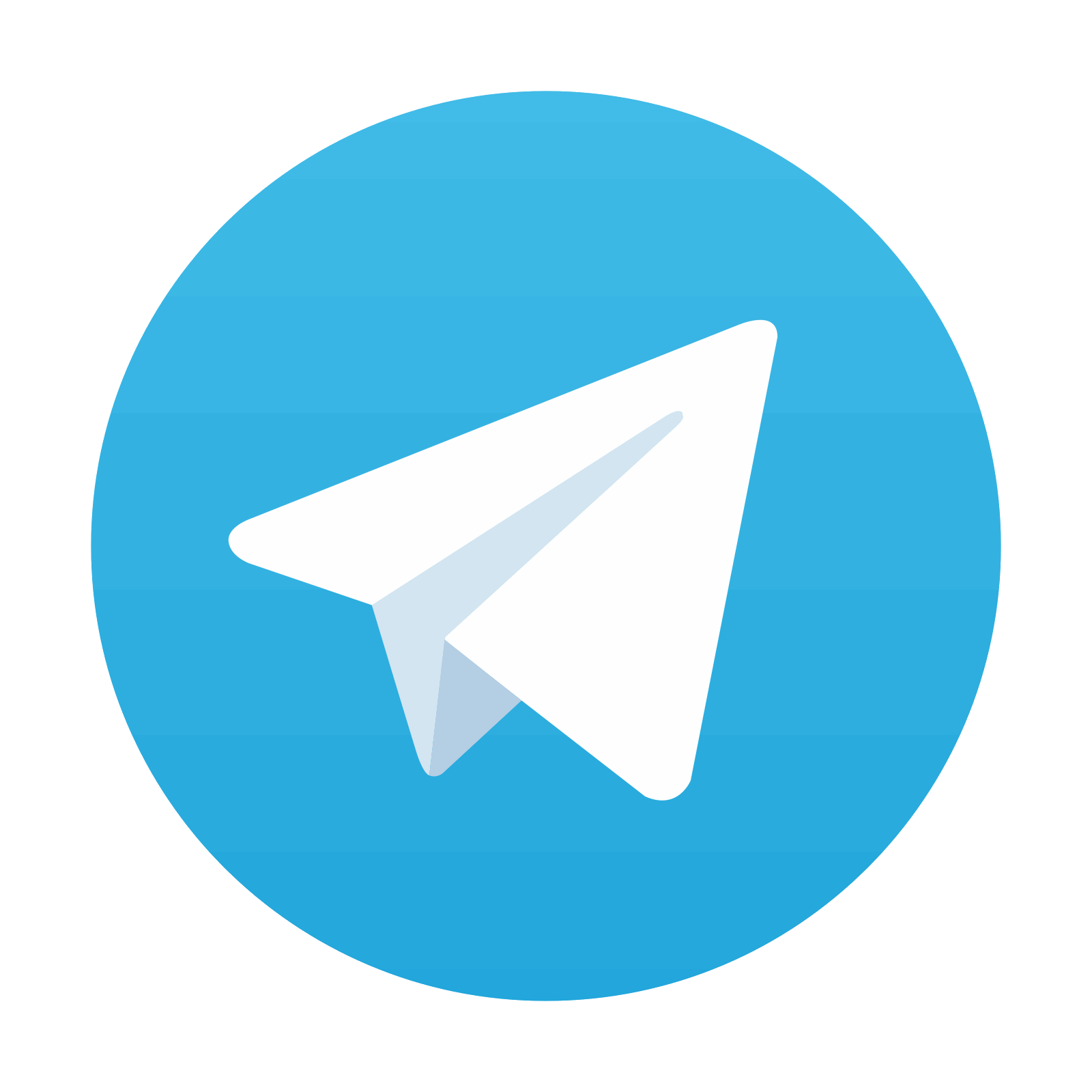
Stay updated, free articles. Join our Telegram channel

Full access? Get Clinical Tree
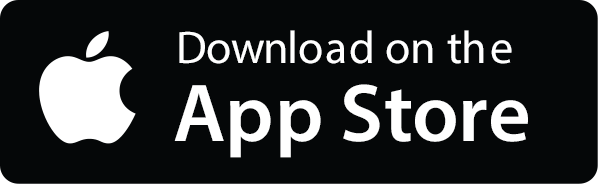
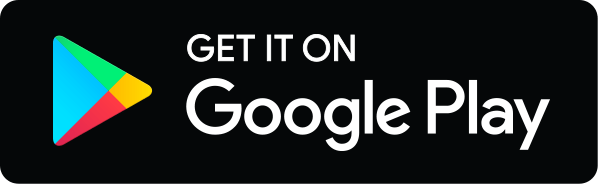