6465CHAPTER 4
Inheritance Patterns in Human Phenotypes and Types of Genetic Disorders
Timothy M. Dwyer, Rivka L. Glaser, and Tracey M. Mason
Genetic conditions can be inherited in various ways. Typical Mendelian patterns of inheritance include autosomal recessive (AR), autosomal dominant (AD), X-linked recessive (XR), X-linked dominant (XD), and Y-linked inheritance. Although Mendelian patterns of inheritance tend to be well known, it is important to note that disorders inherited in this way are rare, compared with complex or multifactorial traits and disorders. In the first section of this chapter, we discuss typical Mendelian patterns of inheritance, in addition to non-Mendelian mechanisms of inheritance such as mitochondrial inheritance, uniparental disomy (UPD), genomic imprinting, gonadal mosaicism, and unstable or expanding triplet repeat mutations. The second section of the chapter is devoted to the different classifications of genetic disorders. Factors affecting the expression of the phenotype are discussed as well.
INHERITANCE PATTERNS OF HUMAN PHENOTYPES
AR Inheritance
In AR inheritance, the mutant gene is located on an autosome rather than on a sex chromosome. Therefore, males and females are affected in equal proportions. The affected person usually inherits one copy of the same mutant gene from each heterozygous (Aa), or carrier, parent, and is thus homozygous (aa) at that locus, having two copies of the mutant gene. Parents who have had a child with an AR disease are sometimes referred to as “obligate heterozygotes,” meaning that each must have one copy of the mutant gene, even if no test for detection exists. Occasionally, a rare recessive disorder is manifested in a person when only one parent is a carrier. This can result in one of two ways:
Because of a small deletion of the chromosome segment involving the normal gene, thus allowing expression of the mutant gene on the other chromosome of the pair
Because the person inherits two copies of the same chromosome from the parent with the mutant gene (UPD)
66Normal gene function is dominant to the altered function of the mutant recessive gene; therefore, the heterozygote usually shows no obvious phenotypic manifestations but, depending on the disorder, the heterozygote may show biochemical differences that form the basis for heterozygote detection by biochemical testing. DNA testing is now commonly used where possible, due to the prevalence of enzyme defects and deficiencies.
In clinical practice, most situations involving AR inheritance come to attention in a variety of ways, as listed in Box 4.1. Therefore, such individuals may have different immediate and long-range needs, ranging from genetic testing and carrier detection to genetic counseling to prenatal diagnosis. The nurse should refer such individuals to a professional providing these services.
If a couple has had a child with an AR disorder, the rest of the family history for the genetic disease may be completely negative, due in part to the trend to smaller family size and in part because two copies of a rare mutant gene are needed in order for one to be affected. If there are other affected individuals, they are usually members of the same generation. If the parents of the affected child are related to each other by blood (consanguinity), this suggests, but does not prove, AR inheritance. The more common the disorder is in the general population, the less relevant is the presence of consanguinity.
The mechanics of transmission of autosomal recessively inherited genes are shown in Figure 4.1. The most common situation is when both parents are heterozygotes (carriers). The theoretical risks for their offspring, regardless of sex, are to be:
Affected with the disorder (aa), 25%
Carriers like their parents (Aa), 50%
Normal, without inheriting the mutant gene (AA), 25%
BOX 4.1
Clinical Practice Situations Involving Autosomal Recessive Inheritance
Recent birth of an affected infant
Recent diagnosis, usually of an affected child
Couples who have been identified as carriers of a specific disorder (e.g., Tay–Sachs disease) and are contemplating marriage or children
One member of a couple has a sibling or cousin known to have a genetic disorder and is concerned that he or she may be a carrier
Both members of a couple belong to a population group in which a specific genetic disorder is frequent (e.g., thalassemia in Mediterranean people)
A couple is contemplating pregnancy after an earlier birth of an affected child, who may be either living or deceased
67
FIGURE 4.1. Mechanisms of autosomal recessive inheritance with one pair of chromosomes and one pair of genes.
Of the phenotypically normal offspring (AA and Aa), two thirds will be carriers. These risks hold true for each pregnancy. Because chance has no memory, each pregnancy is, in essence, a throw of the genetic dice; in other words, the outcome of the past pregnancy has no effect on a future one. These theoretical risks hold true with large numbers of families. Within an individual family at risk with two carrier parents, the actual number of affected children can, by chance, range from none who are affected to all who are affected. This does not change their risks for another pregnancy from those described previously. This is a point that clients often need clarified and reinforced. Nurses should therefore be able to understand it and explain it. If two carriers have had three unaffected children in three sequential pregnancies, it does not mean that their next child will be affected: Each prior event has no bearing on the outcome of the next pregnancy in AR inheritance.
In general, most AR disorders tend to have an earlier, more severe onset than do diseases from other inheritance modes. Many are so severe that they are incompatible with a normal life span, and many affected individuals do not reach reproductive age. Due to recent advances in diagnosis and treatment of certain AR diseases, such as sickle cell anemia and cystic fibrosis, individuals who would have otherwise died in childhood are now reaching young adulthood and having their own children, creating obligatory transmission of the mutant gene to all of their offspring. If the affected person mates with someone who does not carry the same mutant gene, then all of his or her children, regardless of sex, will be carriers, but none will be affected (see Figure 4.1).
68If the affected person mates with someone who is a carrier for the same recessive gene, then there is a 50% risk of having an affected child and a 50% risk of having a child who is a heterozygous carrier, regardless of sex, for each pregnancy. This risk is most likely to materialize for a disorder such as cystic fibrosis in which the frequency of carriers in the White population is about 5%, or for sickle cell disease in which the frequency of carriers in the American Black population is 7% to 9%. If the mother is the one who has the genetic disease in question, there may be effects on a fetus that result from an altered maternal environment, as occurs in phenylketonuria (PKU). The salient characteristics of AR inheritance are summarized in Table 4.1. Examples of different genetic disorders inherited in this manner are presented in Table 4.2, with many of these disorders explained in greater detail in Chapters 8, 9, 10, and 12.
Consanguinity and AR Inheritance
Concern about consanguinity relates mostly to marriage between blood relatives. Although most individuals would be distantly related to their mate if one went back far enough in time, only relationships closer than first cousins are usually genetically important. Each individual carries from 5 to 10 harmful recessive genes that are not usually apparent. Individually, each of these is extremely rare (except for a few, like cystic fibrosis), so that the likelihood of selecting a mate with the same harmful recessive genes is remote. This chance becomes less remote if the two individuals are related to each other by blood or are from the same ethnic group or population isolate. The consequence of consanguineous mating results from the possible bringing together of two identical recessive alleles that are inherited by descent from a common ancestor, thus bringing out deleterious genes in the homozygous (aa) state. The resulting homozygous phenotypes that are deleterious are more obvious than those that are neutral or favorable. This effect may also operate for single-nucleotide variations in genes. Effects that determine one trait are more evident than those contributing to a complex trait, such as body size or intelligence.
TABLE 4.1 Major Characteristics of Autosomal Recessive Inheritance and Disorders
Gene is located on autosome.
Two copies of the mutant gene are needed for phenotypic manifestations.
Males and females are affected in equal numbers on average.
No sex difference in clinical manifestations is usual.
Family history is usually negative, especially for vertical transmission (in more than one generation).
Other affected individuals in the family in the same generation (horizontal transmission) may be seen.
Consanguinity or relatedness is more often present than in other types of inherited conditions.
Fresh gene mutations are rare.
Age of disease onset is usually early—newborn, infancy, or early childhood.
Exert the greatest negative effect on reproductive fitness.
69TABLE 4.2 Selected Genetic Disorders Showing Autosomal Recessive Inheritance | ||
Disorder | Occurrence | Brief Description |
Albinism (tyrosinase negative) | 1:15,000 to 1:40,000 | Tyrosinase negative disorder resulting in melanin lacking in skin, hair, and eyes; nystagmus; photophobia; susceptibility to neoplasia, strabismus, and impaired vision |
Argininosuccinic aciduria (ASA) | 1:60,000 to 1:70,000 | Urea cycle disorder; hyperammonemia, mild mental retardation; vomiting; seizures; coma; abnormal hair shaft |
Cystic fibrosis | 1:2,000 to 1:2,500 (Caucasians) | Ion channel function disruption resulting in pancreatic insufficiency and malabsorption; abnormal exocrine glands; chronic pulmonary disease (see Chapter 9) |
Ellis–van Creveld syndrome (EvC) | Rare, except among eastern Pennsylvania Amish | Multiple mutations in the EVC gene result in short-limbed dwarfism; polydactyly; congenital heart disease; nail anomalies, natal teeth, cleft palate |
Glycogen storage disease Ia (von Gierke disease) | 1:200,000 | Glucose-6-phosphatase deficiency; bruising; hypoglycemia; enlarged liver; hyperlipidemia; lactic acidosis, hyperuricemia; neutropenia; hypertension; short stature |
Glycogen storage disease II (Pompe disease) | 3:100,000 to 4.5:100,000 | Mutation in GAA gene resulting in acid maltase deficiency. Infant, juvenile, and adult forms exist. In infant form, cardiac enlargement, cardiomyopathy, hypotonia, respiratory insufficiency, developmental delay, macroglossia, death from cardiorespiratory failure by about 2 years of age |
Hemochromatosis | 1:3,000 (Caucasians) | Several types exist, each with its own specific mutation. Excessive iron storage and tissue damage can result in cirrhosis, diabetes, pancreatitis, and other diseases; abnormal skin pigmentation seen (see Chapter 10) |
70Homocystinuria | 1:40,000 to 1:140,000 | Cystathionine β-synthase deficiency causing mental retardation; tall build with skeletal defects; optical abnormalities; neurologic problems; risk for myocardial infarction |
Metachromatic leukodystrophy | 1:40,000 | Arylsulfatase A deficiency leading to disintegration of myelin and accumulation of lipids in white matter of brain; psychomotor degeneration; hypotonia; adult, juvenile, and infantile forms |
Sickle cell disease | 1:400 to 1:600 (American Blacks) | Hemoglobinopathy with chronic hemolytic anemia; growth retardation; susceptibility to infection, painful vascular crises, leg ulcers, dactylitis (see Chapter 9) |
Tay–Sachs disease | 1:3,600 (Ashkenazi Jews) | Hexosaminidase A deficiency causing progressive mental and motor retardation with onset at about 6 months; poor muscle tone; deafness; blindness; convulsions; decerebrate rigidity; death usual by 3 to 5 years of age (see Chapters 9 and 10) |
Usher syndromes | Rare | Several types exist, with mutations in multiple genes. Manifests as a group of syndromes characterized by congenital sensorineural deafness, visual loss due to retinitis pigmentosa, vestibular ataxia, occasionally mental retardation, speech problems; several subtypes |
Xeroderma pigmentosa (complementation groups A–G) | 1:60,000 to 1:100,000 | Mutations in genes responsible for DNA repair; sun sensitivity, freckling, atrophic skin lesions, skin cancer develops; photophobia and keratitis; death usually by adulthood. Some types have central nervous system involvement |
71Many cultures and groups have actively encouraged consanguineous marriages. These have included the ancient Egyptians, Incas, royalty, and many modern societies, such as Japan, various Hindu groups in India, Muslim groups (especially in the eastern Mediterranean), and groups in which arranged marriages are an accepted custom. The frequency of consanguineous marriages depends on social custom, religious customs and laws, socioeconomic concerns, family ties and traditions, the degree of geographic isolation of a village, and the degree of isolation of a specific group within a community. It is estimated that in parts of Asia and Africa, consanguineous marriages account for about 20% to 50% of all marriages. Other groups oppose it. In South Korea, it is frowned upon to marry someone with the same family name, and same-clan marriages are barred. Every 10 years or so there is an amnesty period during which such marriages can occur. Among certain followers of the Koran, there are taboos against marriage between a boy and a girl who were breastfed by the same woman more than a certain number of times during the first two years of life. Thus, consanguinity may be perceived differently among different cultures.
AD Inheritance
As in AR inheritance, the mutant gene is on an autosome, so males and females are equally affected. Only one copy of the dominant gene is necessary for the detrimental effects to be evident; the affected individual is heterozygous, and there is no carrier status. It is believed that in most AD disorders, homozygous individuals who have inherited two genes for an AD disorder are so severely affected that they die in utero or in infancy. An example of an exception is familial hypercholesterolemia (see Chapter 10) in which the homozygote survives but shows the very early onset of severe effects. In contrast to AR inheritance, structural protein defects, rather than those involving enzymes, are common. AD disorders are usually less life-threatening than AR ones, although they may have more evident physical malformations.
A later age of onset of symptoms and signs is frequent and may not become evident until adulthood. In practice, persons usually seek counseling or experience events that come to clinical attention for reasons shown in Box 4.2.
BOX 4.2
Usual Clinical Practice Situations Involving Autosomal Dominant (AD) Inheritance
The person or his or her mate is affected with a particular AD disorder.
Someone in their family (often a parent, aunt or uncle, or sibling) has an AD disorder.
They have had a previous child with an AD disorder.
72The recognition of an AD disorder in a child may indicate the presence of that disorder in one of the parents as well. However, there are exceptions. When the parents appear normal, several possibilities exist:
The gene can be present but nonpenetrant (discussed further in the section “Penetrance”).
The gene expression may be minimal and may not have been detected by the practitioner.
The disorder can be caused by a new mutation.
The child is not the natural offspring of both parents.
The following case example demonstrates the extreme importance of careful examination of both parents in the detection of an AD disorder.
CASE EXAMPLE
A child was brought for counseling with full-blown Waardenburg syndrome (deafness, heterochromic irises, partial albinism, and broad facial appearance); however, no evidence of the disorder was at first seen in either parent. This case occurred before subgrouping of this syndrome was known. If the disorder was caused by a new mutation, then the risk for those parents to have this syndrome appear in another child would be negligible. If, however, one of the parents had the syndrome, then the risk for recurrence in another child would be 50%. It turned out that the only manifestation that the mildly affected mother had was a white forelock of hair, which she usually dyed. Thus, simply looking at the couple would not have revealed the situation. This is an example of variable expression (discussed in further detail in Chapter 5) in which the parent was only mildly affected but the child had severe manifestations. Such cases represent a challenge to the practitioner. In this case, the counselor, knowing the full constellation of the syndrome, specifically asked the mother if anyone in the family had premature white hair. If the mother had not been directly asked, she may not have volunteered this information because
the relevance of it was not recognized by the client
of guilty feelings when only one parent transmits a disorder
of fear of stigmatization or being blamed for transmission of the disorder
of other reasons
The mechanisms of transmission of AD traits are shown in Figure 4.2. In matings in which one partner is affected and one is normal, the risk for their child to inherit the gene, and therefore the disorder too (except in disorders with less than 100% penetrance), is 50%, regardless of sex. The chance for a normal child is also 50%. This holds true for each individual pregnancy regardless of the outcomes of prior pregnancies. Unless nonpenetrance has occurred, those truly unaffected individuals run no greater risk than the general population of having an affected child or grandchild of their own. Risk calculations that include the possibility of nonpenetrance can be made by the geneticist. If a woman were an affected heterozygote for a rare AD disorder with 60% penetrance and she was planning a family with a normal man, the risk for each child to both inherit the mutant gene and manifest the disorder is as follows: the risk to inherit the mutant gene from each parent (50% from the mother and the population mutation rate from the father, which in this case is disregarded because of rarity) multiplied by the penetrance (60%) or (0.5 × 0.6 = 0.3). Therefore, the risk for the child to inherit the gene is 50% and to both inherit the gene and manifest the disorder is 30%.
73
FIGURE 4.2. Mechanisms of autosomal dominant inheritance with one pair of chromosomes and one pair of genes.
If two individuals affected with the same AD disorder have children, as is frequently seen in some conditions such as achondroplasia (a type of dwarfism), then for each pregnancy, the chance is 25% for having a child who is an affected homozygote, 50% for having an affected heterozygote like the parents, and 25% for having a normal child without a mutant gene (see Figure 4.2). The homozygote is usually so severely affected that the condition is lethal in utero.
In many AD disorders, the primary defect is still unknown, so that diagnosis of the individual who is known to be at risk for having the disorder before symptoms become clinically evident or prenatal diagnosis for their offspring may not be possible, although gene mapping and DNA technology are making this situation less common. In disorders in which the onset is characteristically late and diagnosis is not available, individuals with a family history of such a disorder have difficulty in making reproductive and life plans because they may not know whether they have inherited the mutant gene. Some choose alternate reproductive options such as artificial insemination, in vitro fertilization, embryo transfer and implantation, or adoption rather than run a possible 50–50 risk, but others 74become aware of the hereditary nature of the disease only after they have had children. Some choose to “take a chance.” Nurses should encourage individuals to talk with their partners about the options, and, if possible, both should also talk with a counselor to clarify their feelings and options. Such supportive counseling may need to be ongoing.
A summary of the major characteristics of AD inheritance is given in Table 4.3. Examples of disorders inherited in an AD manner are shown in Table 4.4.
New Mutation
If no other cases exist in a family and neither parent can be found to have any subclinical signs of the disorder, it may be caused by a new mutation. Such a case is often called de novo or sporadic. The affected person with the new mutation can transmit the disorder to his or her offspring in the same manner as an affected individual with an affected parent. When truly unaffected parents have had a child with a genetic disease caused by a new mutation, the risk of having another child with the same disorder is no greater than for that of the general population (except in rare cases of gonadal mosaicism, explained in the section “Gonadal (Germline) Mosaicism”). New mutations are most frequently seen immediately in the dominantly inherited syndromes, because only one mutant gene is necessary to produce a phenotypic effect. When a recessive single gene disorder appears in a person and both parents are not heterozygous, this should prompt cytogenetic analysis of the affected individual because a microdeletion of chromosomal material that includes the normal gene may be present that allows expression of a single recessive mutant gene without the countering effect of the normal gene that is missing. The more incapacitating the disorder is, the more likely it is for a large percentage to be due to new mutations because the affected person is less likely to reproduce. Disorders in which a high proportion of cases are caused by new mutations include Apert syndrome (an AD disorder with craniostenosis, shallow ocular orbits, and syndactyly) and achondroplasia (a type of disproportionate dwarfism).
TABLE 4.3 Major Characteristics of Autosomal Dominant Inheritance and Disorders
Gene is on autosome.
One copy of the mutant gene is needed for phenotypic effects.
Males and females are affected in equal numbers on average.
No sex difference in clinical manifestations.
Vertical family history through several generations may be seen.
There is wide variability in expression.
Penetrance may be incomplete (gene can appear to skip a generation).
Increased paternal age effect may be seen.
Fresh gene mutation is frequent.
Later age of onset is frequent.
Male-to-male transmission is possible.
Normal offspring of an affected person will have normal children and grandchildren.
Exerts least negative effect on reproductive fitness.
Structural protein defect is often involved.
In general, disorder tends to be less severe than the recessive disorders.
75TABLE 4.4 Selected Genetic Disorders Showing Autosomal Dominant Inheritance | ||
Disorder | Occurrence | Brief Description |
Achondroplasia | 1:10,000 to 1:12,000 | Mutation in the FGFR3 gene involved in development of bone and brain tissue. Short-limbed dwarfism; large head; narrowing of spinal canal |
Adult polycystic kidney disease | 1:250 to 1:1,250 | Mutations in PKD1 or PKD2 genes resulting in enlarged kidneys, hematuria, proteinuria, renal cysts, abdominal mass; eventual renal failure; may be associated (adult) with hypertension, hepatic cysts, diverticula; aneurism resulting in cerebral hemorrhage may occur; cystic kidneys seen on x-ray films (see also Chapter 10) |
Aniridia | 1:100,000 to 1:200,000 | Mutation in the PAX6 gene involved in early eye development. Absence of the iris of the eye to varying degrees; vision impaired; glaucoma may develop; may be associated with other abnormalities in different syndromes |
Facioscapulohumeral muscular dystrophy 1A | 1:100,000 to 5:100,000 | Hypomethylation of the FSHD1 gene resulting in facial weakness; atrophy in facial, upper limb, shoulder girdle, and pelvic girdle muscles; speech may become indistinct; much variability in progression and age of onset |
Familial hypercholesterolemia (type IIa) | 1:200 to 1:500 | Mutation of the LDLR gene causing low-density lipoprotein (LDL) receptor mutation. Symptoms are elevated LDL, xanthomas, arcus lipoides corneae, and coronary artery disease (see Chapter 10) |
Hereditary spherocytosis | 1:2,000 to 1:5,000 | Mutation in ANK1 gene causing a red blood cell membrane defect leading to abnormal spherical shape, impaired survival, and hemolytic anemia due to cell rupture |
Huntington disease | 1:18,000 to 1:25,000 (United States), | Progressive neurologic disease caused by CAG trinucleotide repeat expansion of the HTT gene; involuntary muscle movements with jerkiness, gait changes, lack of coordination, mental deterioration with memory loss, speech problems, personality changes, confusion, and decreased mental capacity; usually begins in mid-adulthood (see Chapter 10) |
76Nail–patella syndrome | 1:50,000 | Mutation of the LMX1B gene causing nail abnormalities, hypoplasia or absent patella, and iliac horns; elbow dysplasia; renal lesions and disease; iris and other eye abnormalities; glaucoma; gastrointestinal problems |
Neurofibromatosis 1 | 1:3,000 to 1:3,300 | Mutation in the NF1 gene involved in skin pigmentation. Café-au-lait spots, neurofibromas, and malignant progression are common; complications include hypertension; variable expression |
Osteogenesis imperfecta type I | 1:30,000 | Mutation in COL1A1 gene responsible for collagen assembly. Blue-gray sclera; fragile bones with multiple fractures; mitral valve prolapse; short stature in some cases; progressive hearing loss in some cases; wormian bones of the cranium (see Chapter 9) |
Polydactyly | 1:100 to 1:300 (Blacks), 1:630 to 1:3,300 (Caucasians) | Extra (supernumerary) digit on hands or feet |
Tuberous sclerosis-1 (TSC1) | About 1:10,000 | Mutation in the TSC1 gene controlling cell growth and size. White ash-leaf-shaped macules and shagreen patches of the skin; facial angiofibromas; erythemic nodular rash in butterfly pattern on face and other skin lesions; seizures; intellectual delay; learning and behavior disorders; may develop retinal pathology and rhabdomyoma of the heart |
van der Woude syndrome | 1:80,000 to 1:100,000 | Mutation of the IRF6 gene involved in transcription factor development. Cleft lip and/or palate with lower lip pits, missing premolars |
von Willebrand disease | 1:1,000 to 30:1,000 | Deficiency or defect in plasma platelet protein called von Willebrand factor, leading to prolonged bleeding time; bruising; bleeding from mucous membranes (nosebleeds) |
77X-Linked Inheritance
In both dominant and recessive X-linked disorders, the mutant gene is located on the X chromosome. Males have only one X chromosome. There is no counterpart for its genes. In males, therefore, any gene located on the X chromosome is expressed when present in one copy regardless of whether it is dominant or recessive in females. Males cannot be carriers; they will show the effects of the gene in question and are said to be hemizygous. A female receives one X chromosome from each of her parents for a normal sex constitution of XX. A male receives his single X chromosome from his mother and his Y chromosome from his father for a normal sex constitution of XY. Whether it is the X chromosome that a woman gets from her father or the X she gets from her mother that is passed to her sons and daughters is random. Figure 4.3 illustrates X and Y chromosome transmission.
FIGURE 4.3. Transmission of the X and Y chromosomes.
78X-Linked Recessive
The most common pattern of XR transmission is that in which the female partner is a heterozygous carrier for the mutant gene (see Figure 4.4). If her partner is normal, then for each pregnancy, the couple runs a 25% chance for the offspring to be one of the following:
A female carrier like the mother
A normal female without the mutant gene
A normal male without the mutant gene
A male who is affected with the disease in question
Thus, the risk for a male offspring to be affected is 50%. As in the other types of single gene inheritance, the outcome of one pregnancy does not influence the others; these odds remain the same. The carrier female usually shows no obvious clinical manifestations of the mutant gene unless X inactivation is skewed (discussed later in this chapter). In such an instance, she may be a manifesting heterozygote. For example, if the mutant gene was for Duchenne muscular dystrophy, a carrier female might demonstrate muscle weakness, enlarged calves, and moderately elevated serum creatine kinase levels. If the mutant gene were for hemophilia A, she might demonstrate prolonged bleeding times. Females with X chromosome abnormalities, even submicroscopic deletions, may also manifest XR disorders if the normal gene on the counterpart chromosome was deleted. Such individuals should have cytogenetic analysis. In practice, individuals at risk for XR disorders usually seek genetic counseling for the reasons shown in Box 4.3.
FIGURE 4.4. Mechanisms of X-linked recessive inheritance with one pair of chromosomes and one pair of genes.
79BOX 4.3
Usual Clinical Practice Situations Involving X-Linked Recessive (XR) Inheritance
Before marriage or before planning children when they have a male family member such as an uncle or brother who has a known XR disorder
After the birth of an affected child
Mother is known heterozygote for XR disorder
Because better treatment has increased the life span for many XR disorders such as hemophilia, affected males are now reproducing. If the female is normal in such a mating, all of their female children will be carriers and all the males will be normal; stated otherwise, the theoretical risk for each pregnancy is that there is a 50% chance that the offspring will be carrier females and a 50% chance that they will be normal males. If the male is affected and the female is a carrier for the same disorder, as may occur in the very common XR disorders such as glucose-6-phosphate dehydrogenase (G6PD) deficiency and color blindness, then with each pregnancy, there will be a theoretical risk of 25% for the birth of each of the following offspring: an affected female, a carrier female, a normal male, or an affected male (see Figure 4.4). A much rarer mating is that of an affected female and normal male in which, with each pregnancy, there is a 50% chance that the child will be a female carrier and a 50% chance that the child will be an affected male.
In the past, little could be accomplished in the way of prenatal detection for XR disorders except to determine the sex of the fetus. For the more common types of matings, this often resulted in the loss of normal, as well as affected, male offspring due to termination of those pregnancies in which the fetus was a male. It is now possible to provide more accurate prenatal diagnosis for many of the XR disorders by using molecular technology, so the nurse should be sure to refer such couples to a genetic counselor for the latest information and not rely on older printed material. A summary of the characteristics of XR disorders is given in Table 4.5, and examples of these disorders are listed in Table 4.6.
X-Linked Dominant
This type is less frequently seen than the other modes of inheritance discussed. Because the mutant gene is dominant, only one copy is necessary for its effects to be manifested phenotypically. Both males and females can be affected, and both can transmit the gene. Because of the gene’s location on the X chromosome, there are several differences between this type of inheritance and AD inheritance:
80TABLE 4.5 Major Characteristics of X-Linked Recessive Inheritance and Disorders |
|
An affected male (except in cases of new mutation) has an affected mother because males inherit their X chromosome from their mother, not their father.
Male-to-male transmission is not seen because males transmit their X chromosome only to their daughters, not to their sons. Thus, an affected male would transmit the disorder to all of his daughters and none of his sons.
There may be an excess of female offspring in the family tree or pedigree, as some XD genes are lethal in the male.
Some affected females may be less severely affected than males because of X inactivation.
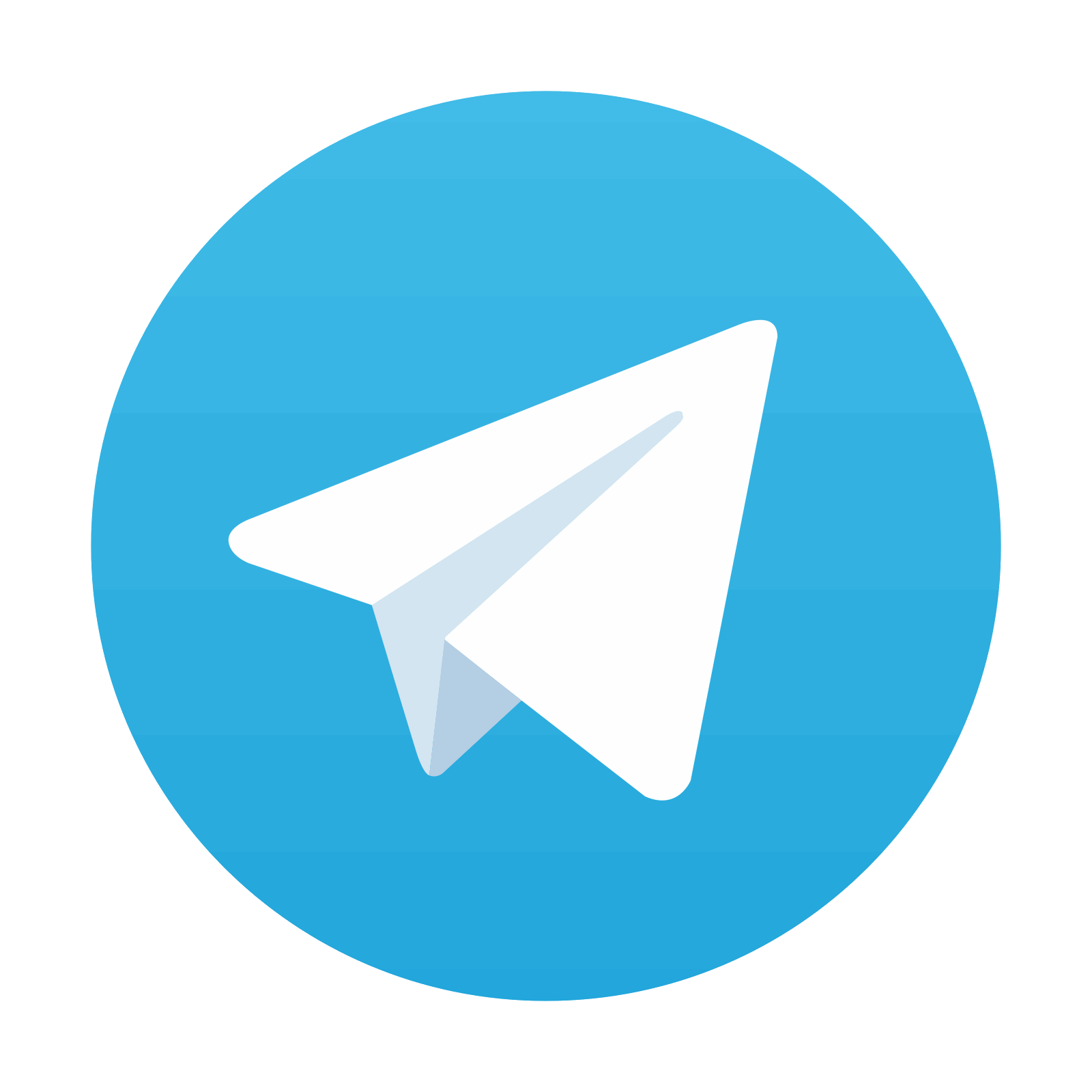
Stay updated, free articles. Join our Telegram channel

Full access? Get Clinical Tree
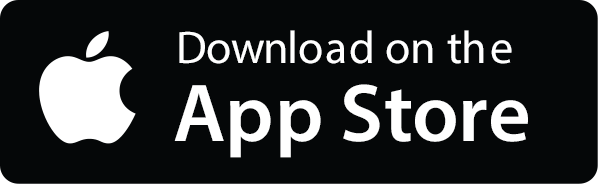
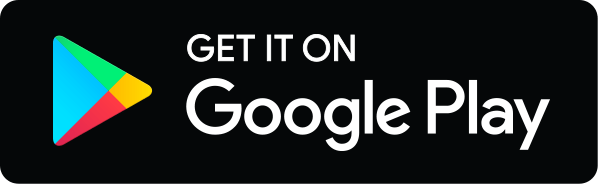