SECTION III. SEPSIS AND SEPTIC SHOCK
Larissa Hutchins and Megan D. Snyder
DEFINITIONS
A. Infection is a microbial phenomenon characterized by an inflammatory response to the presence of microorganisms or the invasion of normally sterile host tissue by those organisms.
B. Bacteremia is the presence of viable bacteria in the blood.
C. Systemic inflammatory response syndrome (SIRS) is a pathophysiologic state of inflammation characterized by acute development of two or more of the following, one of which must be abnormal temperature or leukocyte count (Goldstein, Giroir, & Randolph, 2005):
1. Fever (>38.5°C) or hypothermia (<36°C)
2. Tachycardia (age related) or for children younger than 1 year bradycardia
3. Tachypnea (age related)
4. Leukocytosis or leukopenia (age related and not caused by chemotherapy), or greater than 10% bands
D. Sepsis is the systemic response to infection. It is diagnosed by the presence of SIRS associated with a suspected or proven infectious process and characterized by two or more of the aforementioned findings for SIRS. Documented bacteremia is not necessary for the diagnosis of sepsis.
E. Severe sepsis is defined as sepsis with the presence of one of the following—cardiovascular dysfunction, ARDS, or two or more other organ dysfunctions (Goldstein et al., 2005).
F. Septic shock is defined as sepsis in the presence of cardiovascular dysfunction (Goldstein et al., 2005). Septic shock is “infection with hypothermia or hyperthermia, tachycardia (may be absent with hypothermia), and altered mental status with of at least one, but usually more than one, of the following: decreased peripheral pulses compared with central pulses, capillary refill prolonged for more than 2 seconds (cold shock) or flash capillary refill (warm shock), mottled or cool extremities (cold shock), and decreased urine output” (Carcillo & Fields, 2003, p. 1370).
G. Multi organ dysfunction syndrome (MODS) is defined as the failure of more than one organ (Carcillo & Fields, 2003).
In 2016, the Sepsis-3 consensus definitions for adult sepsis and septic shock were published (Singer et al., 2016), and describe two entities: sepsis and septic shock. The new Sepsis-3 criteria for adult sepsis define sepsis as “life-threatening organ dysfunction caused by a dysregulated host response to infection” (Singer et al., 2016, p. 805). In the adult population, organ dysfunction is classified using the Sequential Organ Failure Assessment (SOFA) score (Vincent et al., 1998). Sepsis-3 defined septic shock as a “subset of sepsis in which underlying circulatory and cellular/metabolic abnormalities are profound enough to substantially increase mortality” (Singer et al., 2016, p. 805). This is operationalized as having a vasopressor requirement to maintain goals for blood pressure and lactate level, in addition to organ dysfunction according to the SOFA score criteria. Furthermore, the Sepsis-3 consensus definitions created a new bedside clinical score, the quickSOFA, consisting of tachypnea, altered mentation, and hypotension. The presence of at least two of these three clinical elements can help rapidly identify adult patients with suspected infection at higher risk of poor outcome. At this time, updated consensus definitions for the pediatric population have not yet been formalized, and pediatric practitioners continue to rely on the pediatric definitions previously described.
ETIOLOGY
A. Children have a predisposition to sepsis and septic shock as a result of environmental, immune maturity, and genetic factors.
B. All microorganisms potentially can lead to septic shock, including bacteria, viruses, fungi, rickettsia, spirochetes, protozoa, mycoplasmas, Chlamydia organisms, and parasites. The Staphylococcus species was the most common causative infecting organism for sepsis, followed by fungal infections in 1995, 2000, and 2005 (Hartman, Linde-Zwirble, Angus, & Watson, 2013).
775C. Causative microorganisms often vary with the following factors:
1. Patient’s age. Watson and colleagues (2003) have shown that age is the most significant influence in the epidemiology of severe sepsis. Pediatric severe sepsis mortality was highest in children younger than 1 year (19.2%), and children 1 to 4 years old had the second highest mortality (13.8%; Ruth et al., 2014). Immaturity of the infant and child’s immune system cause them to be more susceptible to severe infections (Randolph & McCulloh, 2013).
2. Immunocompetence. In immunocompromised patients, the usual source of sepsis is the patient’s endogenous flora. Alternatively, in immunocompetent patients, the usual source is exogenous flora, with some evidence that patients may benefit from protective isolation against ICU-acquired microorganisms (Maki, 1995). Immune system function also impacts susceptibility to fungal infections: children with cancer are twice as likely to develop fungal infections compared to children with other or no comorbidities (Hartman et al., 2013).
3. Location. In healthcare-associated infections (HAIs), the etiologic organism is usually specific to the individual unit, institution, and geographic region. Based on a recent point-prevalence survey, one in 25 hospitalized patients in the United States has an HAI (Magill et al., 2014). Community-acquired infection causes a significant proportion of infections in the pediatric intensive care unit (PICU). Recent studies suggest that there may be an increased risk of mortality with hospital-onset versus community-onset sepsis (Levy et al., 2012; Weiss et al., 2015).
4. Site of Infection. Patients with invasive monitoring devices, mechanical ventilation, and invasive catheters are more likely to acquire HAIs (Urrea, Pons, & Serra, 2003). According to Hartman et al. (2013), when a site of infection was identified nearly half of all infections were attributed to respiratory infections followed by bacteremia. In a pediatric point prevalence sepsis study (Sepsis Prevalence Outcomes and Therapies [SPROUT]), the most common site of infection of the 569 children was the lungs (40%). The bloodstream was the second most frequent site at 19%, followed by the abdomen (8%) and central nervous system (4%; Weiss et al., 2015).
EPIDEMIOLOGY
A. Incidence
1. According to recent estimates by the Centers for Disease Control and Prevention, the rate of hospitalization for sepsis has more than doubled between 2000 and 2008 (from 11.6 to 24 per 10,000) and now accounts for over 720,000 hospitalizations annually in the United States. Over 75,000 pediatric hospitalizations involved severe sepsis in the United States in 2005, with an estimated mortality of 8% to 10% (Hartman et al., 2013). Sepsis remains among the top 10 leading causes of death in children 0 to 9 years (National Vital Statistics System, National Center for Health Statistics, Centers for Disease Control and Prevention, 2016).
2. The burden of sepsis continues despite advances in medical care (Watson et al., 2003). In 2008, the United States spent an estimated $14.6 billion in healthcare expenditures for septicemia (National Vital Statistics System, National Center for Health Statistics, Centers for Disease Control and Prevention, 2014). Chronic illness is a major predisposing factor for sepsis (Watson et al., 2003). Watson and colleagues (2003) showed that infants have more underlying comorbidities related to neurologic and cardiovascular disease, whereas children usually have comorbidities of cancer and immunodeficiency disorders (Watson et al., 2003). The most common comorbidities for pediatric sepsis patients are neuromuscular, cardiovascular, and respiratory (Hartman et al., 2013).
B. Risk Factors
1. Susceptible patients are those with extremes in age (neonates and children younger than 3 years of age), noncompliance with immunization schedules, malnourishment or failure to thrive, chronic illness, malignancy, immunosuppressive therapy (e.g., malignancy, transplant recipient), primary immunodeficiency, asplenia, AIDS, and congenital heart disease.
2. Aggressive microorganisms have changing resistance patterns.
3. Several factors increase the risk of infection in children who are in the PICU. These include invasive procedures, immunosuppression, and the physiologic immunodeficiency related to the age of the child (Urrea et al., 2003). A recent study of 254 long-stay PICU patients without known baseline immunocompromise found several admission characteristics associated with increased HAI risk (increasing age, higher Pediatric Risk of Mortality Score [PRISM] III scores, diagnoses of trauma or cardiac arrest, and lymphopenia; Carcillo et al., 2016). According to Carcillo et al. (2016), up to 40% of children in the PICU for 14 days or longer will acquire an HAI and/or sepsis. It is also important to note that the 776risk of secondary infections and HAIs is increased in patients with MODS and immunoparalysis (Hall, Knatz, et al., 2011).
DEVELOPMENTAL ANATOMY AND PHYSIOLOGY
A. Immunity of the young child is not equal to that of the developmentally mature host. Several aspects of the infant and young child’s first, second, and third lines of defense are immature. The healthy infant and young child are not immunocompromised; rather, they are immunologically inexperienced. The immune system is not fully mature until adolescence (Randolph & McCulloh, 2014). See Chapter 8.
B. Relationship of shock to developmental physiology: Shock is present when metabolic demand needs are not met by or are uncoupled from oxygen and energy supply. Metabolic supply and demand are impacted by cardiac output (CO) and oxygen consumption.
1. CO regulation. See “Embryonic, Neonatal, and Pediatric Cardiovascular Physiology” section in Chapter 3. Changes in the child’s CO accompany the child’s growth and development. CO is greatest at birth (200 mL/kg/min) and then decreases throughout childhood to adolescence (100 mL/kg/min; Hazinski, 2013). This decrease is related to two events (Alyn & Baker, 1992): (a) a decrease in fetal hemoglobin and an increase in adult hemoglobin and (b) lower oxygen requirements secondary to a changing surface area. In the young child, CO is directly proportional to heart rate (Hazinski, 2013).
2. Oxygen consumption. Oxygen consumption is the volume of oxygen consumed by the tissues per unit of time. Oxygen consumption is the product of the CO and the amount of oxygen extracted from each milliliter of blood, expressed as mL/kg/min. Changes in the child’s oxygen consumption accompany the child’s growth and development. As with CO, oxygen consumption decreases throughout childhood. Normal values in the fetus are 8 mL/kg/min (Alyn & Baker, 1992); in the infant, 10 to 14 mL/kg/min; and in the child, 5 to 8 mL/kg/min (Hazinski, 2013).
PATHOPHYSIOLOGY
A. All surfaces of the body exposed in any way to the external environment serve as the first line of defense. When the microorganism breeches any of these barriers, it gains access to the body’s internal environment.
B. The inflammatory immune response, the second line of defense, is then triggered in an effort to eliminate or neutralize the microorganism and its toxins, contain the microorganism invasion, prevent access to the body’s systemic environment (i.e., bloodstream), and promote rapid healing of involved tissues.
1. SIRS. With systemic release of the microorganism and its toxins, there is an activation and release of various mediators and cytokines, resulting in SIRS. SIRS can occur both in the presence of infections and in a noninfectious state. The SIRS response can also be triggered by a state of hypoperfusion, leading to hypoxic–ischemic injury commonly seen in septic shock. Septic shock includes a component of SIRS, but is triggered by the presence of infection. A local infection may progress into a systemic infection, triggering SIRS in a patient. Left uncontrolled, SIRS in addition to microbiologic toxins may lead to multiple organ system failure and death (Figure 9.7).
2. Mechanisms within the inflammatory response and SIRS are the same, the differences being in the extent and magnitude of the response. Events or mechanisms of the inflammatory response serve protective functions, whereas SIRS can cause deleterious outcomes.
3. The activation and release of various mediators result from two sources: exogenous and endogenous.
a. Exogenous mediators are released by the invading microorganism. Microorganism mediators include, but are not limited to endotoxin (released from gram-negative bacteria), exotoxin (released from gram-positive bacteria), and mannan (released from fungal cell walls). Endotoxin, a lipopolysaccharide that is an integral part of the outer membrane of all gram-negative bacteria, is the most commonly studied toxin in sepsis. Endotoxin is shed as bacteria multiply or die. The body’s response to infection and presence of endotoxins is seen in inflammation, activation of coagulation, fever, tachypnea, lactic acidosis, and shock (Schoenmakers, Reitsma, & Spek, 2005).
b. Endogenous mediators are synthesized or activated by the host in response to an insult or invading microorganism (exogenous mediators).
4. Proinflammatory mediators. Cytokines are a group of proteins responsible for the inflammatory response. In large-enough concentrations they can cause damage to tissue. Animal studies have demonstrated that the infusion of cytokines (tumor necrosis factor alpha [TNF-α]) in large-enough quantities triggered the inflammatory response as seen in severe infection (Gotts & Matthay, 2016). Proinflammatory cytokines also include interferon gamma, interleukins (IL1 and IL8), histamine, and platelet activating factor.
777
FIGURE 9.7 Outcomes of mediator release in SIRS, sepsis, and septic shock.
SIRS, systemic inflammatory response syndrome.
The initial response of the host to bacteria is the monocyte–macrophage cell response. Neutrophils attempt to manage the infection at a local level to prevent systemic spread by bringing immune cells to the site (Schulte, Bernhagen, & Bucala, 2013). The monocyte cells phagocytose bacteria and release proinflammatory mediators (cytokines) such as TNF and IL-1 (de Pablo, Monserrat, Aprieto, & Alvarez-Mon, 2014).
5. Anti-inflammatory mediators. The proinflammatory response of the body is also balanced by an anti-inflammatory response mediated by such cytokines as IL-6, IL-10, transforming growth factor (TGF), and IL-4. The anti-inflammatory response attempts to decrease the inflammatory response, which, if high enough, can cause tissue damage (Schulte et al., 2013). Vagal nerve stimulation also plays a role in managing cytokine signaling and response (Gotts & Matthay, 2016).
C. In septic shock, the mechanisms of each of the mediators vary, but the overall result of the exaggerated release of exogenous and endogenous mediators includes a distributive, a cardiogenic, and a hypovolemic state. Distributive shock is caused by vascular tone changes and maldistribution of blood flow. Cardiogenic shock is caused by myocardial depression. A hypovolemic shock state is relative to vasodilation and actual dehydration. The patient experiencing septic shock will develop endothelial damage, clotting abnormalities, and metabolic alterations (Figure 9.7).
1. Distributive Shock
a. Vasodilation (vascular tone changes). Acute vasodilation caused by inflammatory mediators, nitric oxide release, and presence of endotoxins results in a relative hypovolemia. The vasodilation results in a decrease in systemic vascular resistance (SVR) and decreased preload. Injury of the endothelium also causes the release of nitric oxide by the activation of nitric oxide synthases from the endothelium to the connecting cells. The presence of nitric oxide synthase acts on the vascular smooth muscle by increasing cyclic guanylate monophosphate (C-GMP), which causes smooth muscle relaxation. This relaxation of the vasculature, or vasodilation, can contribute to a state of hypovolemia and hypoperfusion.
b. Maldistribution of blood flow. Maldistribution of blood flow leading to decreased oxygen delivery and tissue hypoxia is seen in septic shock. Endothelial cells stimulated by proinflammatory mediators and endotoxin cause activation of the coagulation cascade, and creation of microvascular plugs. Endothelin, released from the endothelium, and TNF cause vasoconstriction of arterioles, whereas vasodilation occurs in other vessels.
7782. Cardiogenic Shock. Myocardial depression may be caused by endotoxin release, the presence of cytokines in high concentrations, release of nitric oxide, and alterations in the sympathetic nervous system response. Myocardial depressant factor is thought to be released by the pancreas during hypoperfusion and ischemia.
3. Hypovolemia. Hypovolemia is caused by distributive shock and dehydration.
4. Endothelial Damage. Damage to the endothelium is caused by the effects of the inflammatory response mediators as well as endotoxin/ exotoxin exposure. When damage occurs to the endothelium, small openings on the wall of the endothelium lead to intervascular fluids leaking out of the vasculature and into the interstitial tissues. This capillary leak and shift of fluid from the intravascular space, paired with massive vasodilation, can result in hypovolemia leading to hypoperfusion. Changes to the endothelium may have a direct result on the function of organs, such as the lung, leading to interstitial edema and changes to the alveolar epithelial barrier, leading to impairment in oxygenation, ventilation, and lung compliance (Gotts & Matthay, 2016). In the gut, changes to the endothelial lining may increase the possibility of bacterial translocation and injury to the gut. The liver, kidneys, and nervous systems are all susceptible to injury and malfunction due to the effects of endotheliopathy seen in sepsis (Gotts & Matthay, 2016; Figure 9.8).
5. Alterations in Coagulation. DIC may be triggered during the inflammatory response seen with septic 779shock. DIC is an inappropriate accelerated systemic activation of the coagulation system. DIC is triggered by injury to tissue by the release of proinflammatory cytokines, causing a release of tissue factor, which triggers the initiation of the coagulation cascade. As the coagulation cascade is initiated, clotting is occurring with microvascular plugs in small vessels. As the clotting factors and platelets are being consumed, the antithrombotic mechanisms are stimulated and eventually also used up. DIC and the associated ischemia and hemorrhage cause organ dysfunction, and can lead to uncontrolled bleeding (Gotts & Matthay, 2016).
FIGURE 9.8 Processes leading to maldistribution of circulating volume.
Source: From Robins, E. V. (1996). Maldistribution of circulating volume. In V. B. Huddleston (Ed.), Multisystem organ failure: Pathophysiology and clinical implications (2nd ed.). St. Louis, MO: Mosby-Year Book.
6. Imbalance of Oxygen Supply and Demand
a. Maldistribution of blood flow exacerbates hypoxemia and may lead to ventilation perfusion abnormalities and intrapulmonary shunting. Imbalance of oxygen supply and demand with SIRS and septic shock are due to the decrease in the oxygen delivery secondary to myocardial dysfunction and the decrease in the tissue’s ability to extract the oxygen.
b. In normal circumstances, with oxygen present the cells produce energy through cellular respiration. In times of stress, such as fever, seizure, or pain, the consumption of oxygen will increase. Oxygen consumption is dependent on oxygen transport. If oxygen delivery does not increase enough to meet the demand, the cells will resort to the use of anaerobic metabolism. This will produce lactic acidosis, quickly using available glucose and is a short-term measure. In order to increase the supply of available oxygen, the body will attempt to compensate by increasing the CO by increasing the heart rate. The presence of low CO more than low SVR, as seen in adults, is associated with mortality in pediatric septic shock (Brierley et al., 2009).
7. Compensatory responses to shock are mechanisms to increase tissue perfusion and prevent cellular, tissue, and vital organ injury in the presence of all shock states. Most compensatory mechanisms are dependent on various “sensing” mechanisms to recognize changes in the CO or arterial blood pressure.
8. Compensatory mechanisms are sequentially stimulated in an effort to maintain perfusion.
a. Stretch receptors (in the right atrium and pulmonary artery) sense volume changes, either from decreased circulating volume or, in the case of septic shock, from increased venous capacitance. Stimulation of these receptors results in an increase in sympathetic discharge to the medullary vasomotor center.
b. Baroreceptors within the renal juxtaglomerular apparatus are stimulated by reduced renal afferent arteriolar pressure. The reduction in pressure causes activation of the renin–angiotensin–aldosterone system, which results in vasoconstriction of arterioles (and, to a lesser extent, veins), increased renal tubular reabsorption of sodium and water, increased water absorption, and increased thirst. A drop in MAP or pulse pressure close to physiologic range results in the decreased stretching of arterial baroreceptors (in aortic arch, carotid bodies, and splanchnic vessels) and loss of their inhibitory effect on the vasomotor center (baroreceptor reflex). Sympathetic vasomotor stimulation causes norepinephrine release from nerve endings and widespread vasoconstriction.
c. Norepinephrine–epinephrine vasoconstrictor reflex. Sympathetic vasomotor stimulation causes stimulation of the adrenal medulla and the release of epinephrine and norepinephrine. This causes vasoconstriction and increased CO.
d. Vascular chemoreceptors are located in tissue beds and are sensitive to changes in PO2, PCO2, and decreased pH (increased hydrogen ion concentration) related to low blood pressure. On activation, sympathetic tone increases and respiratory stimulation is triggered to help compensate for the acidosis.
CLINICAL PRESENTATION
A. History
1. Chief Complaint. The history is guided by the child’s chief complaint. The chief complaint is noted in the patient’s or the primary caretaker’s own words.
2. History of the present illness includes the date and mode of onset, course, duration, influencing factors, and exposure to infectious agents, including contact with infectious persons, animal (domestic or wild) bites, ingestion of contaminated food or water, and foreign travel. Signs and symptoms to be noted include the following:
a. General. Fatigue, change in level of activity, chills, fever, weight loss, change in feeding, night sweats, malaise
b. Mental status. Confusion, restlessness, syncope, irritability, somnolence
780c. Skin. Rash, petechiae, pallor, mottling, lesions, ulcers, rhinitis, temperature
d. Lymph nodes. Enlargement (adenopathy), tenderness
e. Respiratory status. Tachypnea, respiratory tract infection, respiratory distress, dyspnea, orthopnea, cough, hemoptysis, sputum, chest pain
f. Cardiovascular status. Tachycardia, flushed skin
g. Abdomen. Anorexia, altered bowel sounds, diarrhea, constipation, melena, vomiting, hematemesis, protuberant abdomen (not age appropriate), abdominal pain, masses, hepatosplenomegaly
h. Genitourinary tract. Hematuria, number of voids or diapers per day
3. Medical History
a. Aspects that alter the child’s resistance to infection include recent injury, diet and nutrition, immunization history, and birth history.
b. Aspects that increase the child’s risk of infection include prolonged antibiotic therapy, previous surgeries (e.g., thymectomy, splenectomy), liver or spleen disorders (functional splenectomy), metabolic and immune disorders (diabetes mellitus, renal disease, primary immunodeficiency, and allergies or hypersensitivities), malignancy, and transplantation.
c. Previous infections, including childhood infectious diseases, should be noted.
4. Family history should note familial diseases that may increase the child’s risk of infection.
5. Medication history should include those that increase the child’s risk of infection. Also consider any dietary supplements the child is taking.
6. Social–cultural history and habits include psychosocial history, environmental exposures such as radiation (either inadvertent exposure or radiation therapies [total or localized]) or chemicals (inadvertent exposure to benzene, lead, etc.), as well as recent travel.
B. Clinical Assessment
For patients with suspected sepsis, “a rapid assessment of perfusion should focus on heart rate, blood pressure, capillary refill, quality of peripheral and central pulses, and mental status” (Martin & Weiss, 2015, p. 143). “Nurses are in an advantageous position to recognize critical status changes, indicating a patient experiencing sepsis” (Jeffery, Mutsch, & Knapp, 2014).
1. Inspection includes LOC, level of activity, general appearance, and respiratory rate, rhythm, and effort. Skin and mucous membranes should be examined for color and consistency, the presence of lesions, and the presence of generalized or localized edema, which is usually periorbital or sacral. Pedal edema may occur after the child begins to walk. Characteristics of fontanelles and jugular venous distention are noted to assess hydration status. Precordial activity is noted and is due to hypertrophy of the right ventricle, causing the lower end of the sternum and ribs to project forward. Although the point of maximal intensity (PMI) may be visible in some children, especially those who are thin, a prominent or heaving precordium may be an indication of cardiac dysfunction.
2. Palpation
a. Skin and mucous membranes are palpated for moisture, texture, refill, and temperature (note any demarcation in temperature from distal to proximal extremities and extremities versus the trunk). With cardiac dysfunction, blood is circulated at an insufficient rate, and compensatory vasoconstriction occurs in the extremities to shunt blood toward vital organs. Normal capillary refill is less than or equal to 2 seconds. The presence and intensity of peripheral pulses are noted. Also note proximal versus distal pulse quality because it is reflective of perfusion status. The clinical presentation of septic shock in children is more variable than in adults. Pediatric patients more commonly present in “cold shock”—a state of elevated SVR and low CO with delayed capillary refill and cold extremities (Martin & Weiss, 2015).
b. The level at which the normal liver border may be palpable is related to age. In the infant, the liver is normally felt up to 3 cm below the right costal margin; in the 1-year-old child, up to 2 cm below the right costal margin; and in the 4- to 5-year-old child, up to 1 cm below the right costal margin. The presence of hepatomegaly may indicate cardiac dysfunction, tumor, or hepatitis. An enlarged liver may also indicate fluid overload because the liver may act as a sponge and become engorged when central venous pressure (CVP) increases.
7813. Auscultation. The presence and quality of breath sounds are noted. Respiratory rate and heart rate should be evaluated within the context of the child’s age, clinical condition, and other external factors, such as fever. Apical heart rate and rhythm are auscultated. In the infant and young child, CO is directly proportional to heart rate because stroke volume is small. Blood pressure is a late sign of decompensation, but is still obtained. Blood pressure should be evaluated in the context of the child’s age, clinical condition, and other parameters reflective of perfusion.
C. Invasive and Noninvasive Diagnostic Studies
1. Serum hematologic studies include:
a. CBC (see Chapter 8)
b. White blood cell (WBC) count: Total WBC count is generally considered normal at 5,000 to 10,000/mm3 but is age specific. Leukocytosis occurs in all forms of shock as a result of the demargination of neutrophils (Kumar & Parrillo, 2001). Leukopenia occurs in late septic shock or in infants and young children who are less able to replace neutrophils repeatedly in the face of an overwhelming infection. The WBC differential measures the five subcategories of circulating WBCs and is reported as a percentage. Neutropenia may be seen. An increased percentage (more than 10%) of bands (immature neutrophils) is seen when there is an increased demand for or decreased supply of neutrophils in the presence of an overwhelming infection (a shift to the left). A lymphocyte count of less than 1,000 for longer than 7 days may also be seen (Carcillo & Fields, 2003). Absolute granulocyte or neutrophil counts (AGC or ANC) may be low. An ANC lower than 1,000/mm3 carries a moderate risk for infection, and an ANC lower than 500/mm3 carries a high risk for infection.
c. Hemoglobin is variably affected. With the extravasation of intravascular water, erythrocytosis usually occurs.
d. Platelet count increases acutely but may be followed by thrombocytopenia with progressive septic shock.
e. Other serum tests of inflammation that may be helpful include erythrocyte sedimentation rate (ESR) and C-reactive protein, which are elevated secondary to an infectious process.
f. Procalcitonin (PCT) is an amino acid peptide exclusively produced by the neuroendocrine cells in response to endotoxin or mediators released in response to bacterial infections; thus, PCT normally circulates at very low levels (Pierce, Bingham, & Giuliano, 2014; Schuetz, Albrich, & Mueller, 2011). PCT is a reliable serum marker for detecting the presence or absence of invasive bacterial infection and response to antibiotic therapy (Pierce et al., 2014). PCT levels greater than 2 ng/mL may be predictive of bacterial infection in febrile children.
2. ABG analysis. Respiratory alkalosis occurs early in the course of septic shock. Initial respiratory alkalosis occurs as a compensatory mechanism to reduce carbon dioxide in the presence of increasing lactic acidosis from decreased perfusion. As septic shock progresses and respiratory reserves fail, respiratory acidosis develops. The body is unable to compensate for the increasing acid buildup. An anion gap metabolic acidosis is present because of the elevated levels of lactic acid.
3. Routine chemistries. Sodium, potassium, chloride, and bicarbonate are required to assess an anion gap. BUN and creatinine levels may initially appear normal. Lactate should be followed serially because it is a marker of tissue oxygen debt and supply-dependent oxygen consumption. It is a late marker of tissue hypoperfusion but one of the few available means of estimating tissue oxygenation. Arterial lactate levels greater than 2 mEq/L are associated with increased mortality in the adult population. Pediatric patients with severe sepsis or septic shock should have their blood glucose and calcium levels monitored because they are at risk of hyper/hypoglycemia and hypocalcemia (Brierley et al., 2009). Low glycogen stores of infants and young children in shock states significantly increase their risk of developing hypoglycemia (Brierley et al., 2009). Furthermore, observational studies have identified hyperglycemia as a risk factor for mortality in children with septic shock (Branco et al., 2005). Current guidelines recommend insulin therapy to avoid hyperglycemia (blood sugar ≥180 mg/dL) while also avoiding hypoglycemia (Brierley et al., 2009).
4. Blood cultures may identify causative microorganisms. Aerobic and anaerobic cultures are usually obtained. Obtaining blood cultures is a simultaneous priority with administration of broad-spectrum 782antibiotic coverage. Attempt to obtain blood cultures before the administration of antibiotics, but never “hold” antibiotic administration to obtain blood cultures.
a. The timing of culture results will vary. Results from cultures for common microorganisms, such as streptococci, staphylococci, and Enterobacter, are available in 24 to 48 hours.
b. Results from blood cultures may be negative. Some microorganisms, such as Mycobacterium tuberculosis, shed intermittently, causing negative results from cultures. In these instances, serial cultures may be required. Some patients, especially those who have received antibiotics, may also yield negative results from blood cultures. Only 50% of the patients with clinical signs of sepsis will have an organism isolated (Hartman et al., 2013). When an organism or clear source of infection cannot be identified, it is referred to as culture-negative sepsis.
5. Other specimens and cultures
a. Sputum specimens from the lower respiratory tract versus oropharyngeal secretions may be helpful.
b. Fecal cultures are used to find organisms that are not a part of the normal bowel flora or to determine normal flora that become pathogenic (e.g., Clostridium difficile, Escherichia coli).
c. Urine specimens with bacterial counts of less than 10,000 colony-forming units (CFU)/mL of urine are considered free of infection. Bacterial levels between 10,000 and 100,000 CFU/mL in urine cultures are inconclusive and require a second specimen. Bacterial counts greater than 100,000 CFU/mL of urine represent a definitive urinary tract infection.
d. Cerebrospinal fluid. Lumbar puncture may be needed to evaluate spinal fluid. Gram stains are particularly useful with cerebrospinal fluid infections for the purpose of selecting antibiotics.
6. Routine chest radiography is useful for ruling out pneumonia as the source of infection. Radiographic studies of other areas of the body are indicated by the child’s history and physical examination.
7. Invasive hemodynamic monitoring should be considered with patients who do not respond quickly to initial fluid boluses or who do not demonstrate adequate physiologic reserve (Davis et al., 2017).
a. All patients with suspected shock should have an indwelling arterial catheter to monitor blood pressure serially. Blood pressure assessment via manual sphygmomanometer or automatic noninvasive oscillometric techniques may be inaccurate in patients in shock secondary to marked peripheral vasoconstriction.
b. CVP is often used to manage the child with septic shock to follow trends in intravascular volume status and responses to therapy. CVP is measured through a central venous catheter with the tip of the line located in the SCV–RA junction.
c. A pulmonary artery catheter may be helpful in managing the child in florid septic shock; however, pulmonary artery catheter use is becoming less common and more controversial. It provides continuous monitoring of cardiac filling pressures (CVP and pulmonary artery wedge pressure [PAWP]), an estimate of left ventricular end-diastolic pressure [LVEDP] and volume [LVEDV]), cardiac flow (CO per cardiac index [CO/CI]), cardiac contractility (stroke volume, stroke volume index, stroke work index), and afterload (SVR, systemic vascular resistance index [SVRI]). It also allows the withdrawal of blood from the pulmonary artery catheter to determine oxygen consumption: pulmonary artery oxygen content and mixed venous oxygen saturation (SvO2).
8. Echocardiogram is a noninvasive tool that can be used to evaluate myocardial contractility and assess hemodynamics in patients with fluid refractory shock (Davis et al., 2017).
D. Phases and Clinical Manifestations of Septic Shock (Table 9.24). Stages may be identified in the clinical progression of septic shock in some children. During the hyperdynamic compensated phase, blood pressure is maintained. In the later hyperdynamic uncompensated phase, blood pressure begins to fall. The 783hypodynamic state essentially appears congruent to cardiogenic shock.
PATIENT CARE MANAGEMENT
A. Guidelines for the management of children in septic shock were published in 2002, 2007, and in 2014 (Davis et al., 2017). Use of these guidelines has improved the outcomes for children in septic shock. The updated guidelines provide recommendations that each institution develop tools to identify and manage patients experiencing septic shock. Best practice quality-improvement efforts, including recommendations of treatment within the first hour of recognition, establishing IV access, initiation of IV fluids for resuscitation, administration of antibiotics, and initiation of vasoactive medications to support adequate perfusion, are needed. Many institutions have created management bundles and audit the care provided in the management of patients with septic shock based on these recommendations (Davis et al., 2017).
B. Essential treatment of septic shock must include both definitive treatment, including identification, localization, and eradication of the source of infection with antibiotic administration and source control procedures if necessary, and advanced life support treatment, including oxygenation, ventilation, and circulation with fluid and inotropic administration. Controversial treatments are those with conflicting results or undetermined efficacy such as steroids for fluid refractory catecholamine-resistant shock and plasma exchange. Futuristic treatments are those currently under investigation or scheduled for investigation in the near future such as passive immunization and monoclonal antibody administration. Controversial and futuristic treatments often fall into one of two approaches, which include either neutralizing microbiologic toxins or modulating host inflammatory immune responses.
TABLE 9.24 Phases and Clinical Manifestations of Septic Shock
Fluid-resistant shock | Shock persists despite 60 mL/kg resuscitation |
Catecholamine-resistant shock | Shock persists despite dopamine 10 mcg/kg/min and direct acting catecholamines (epinephrine, norepinephrine) |
Warm shock | Vasodilation, low SVR, high CO Warm extremities, flash capillary refill <1 sec, bounding pulse Decreased diastolic blood pressure, wide pulse pressure (>40 mmHg) |
Cold shock | High SVR, low CO Cold extremities, prolonged capillary refill (>3 sec), faint pulses Normal or increased diastolic blood pressure Narrow pulse pressure (<30 mmHg) |
CO, cardiac output; SVR, systemic vascular resistance.
Source: Permission obtained from Scott Weiss, MD.
C. Identify those patients at risk for the development of sepsis or septic shock. Rapid identification of the patient experiencing sepsis and septic shock is essential in the rapid treatment and effective management of the patient. The adult literature defines shock by hypotension and increased lactate in a normotensive patient (Brierly et al., 2009). Early recognition of sepsis or septic shock can be challenging in pediatrics (because either may be subtle and insidious), especially in younger patients, such as neonates and infants and patients undergoing treatments or interventions that alter inflammatory immune response, including glucocorticoid administration. Hypotension may be a delayed or late response in young children. Although teenagers and adults may first experience warm shock with increased CO and decreased SVR, a young child may experience vasoconstriction with low CO and poor peripheral perfusion. This vasoconstriction seen with a cold shock state causes the hypotension to be seen later (Martin & Weiss, 2015). The current recommendation is for early recognition of shock in pediatrics using clinical examination (Brierly et al., 2009). 784Shock may be identified by hypothermia or hyperthermia, tachypnea, altered mental status and oliguria, vasodilation (indicative of warm shock), or vasoconstriction with delayed capillary refill (indicative of cold shock; Brierly et al., 2009; Davis et al., 2017). Bradycardia or tachycardia is also seen. Monitor ongoing changes in the patient or the patient’s response(s), such as behavior, level of consciousness, temperature patterns (e.g., fever), and WBC count.
D. Initial Resuscitation (see Figure 9.9)
1. Assess ABCs. Rapid cardiopulmonary assessment, proposed by the American Heart Association PALS program includes a primary survey of life-threatening conditions followed by a secondary survey. Once the ABCs are ensured, the child should receive, as required, antibiotic administration, volume resuscitation, and vasoactive agents. Vascular access will quickly need to be obtained for fluid and IV therapy. Access may be difficult to obtain in a child or infant experiencing septic shock. PALS guidelines provide direction on the placement of peripheral and central access, including the use of umbilical access and intraosseous access (Brierly et al., 2009).
2. Volume Resuscitation. In a child with septic shock who is hypovolemic, an aggressive approach is taken as fluid resuscitation can reverse the shock state and increase odds of survival. A 20 mL/kg IV fluid bolus with subsequent boluses of 20 mL/kg over 5 minutes is started soon after presentation. A septic patient may require a large volume of crystalloid NS or lactated Ringers) reaching 60 to 200 mL/kg in the first hour of resuscitation (Brierly et al., 2009). Fluid boluses should be administered and titrated to assessment of the patient clinical state with frequent reassessment for the development of volume overload. Age-specific parameters for blood pressure and heart rate should be considered. The assessment should include heart rate, blood pressure, peripheral pulses, capillary refill, urine output, level of consciousness, and skin temperature for signs of improvement (Brierly et al., 2009). Colloids, such as 5% albumin, may also be used. Fresh frozen plasma may be used to correct PT or partial thromboplastin time (PTT). Packed red blood cells may also be chosen to increase hemoglobin and to act as a volume expander (Brierly et al., 2009; Davis et al., 2017). During fluid resuscitation, patients should also be assessed for signs of fluid overload such as increased rales, cardiac gallop, or hepatomegaly (Brierley et al., 2009). A study by Rivers and colleagues (2001) found that early aggressive volume resuscitation in patients with severe sepsis and septic shock improved the likelihood of survival. Patients who do not respond to fluid boluses may need invasive hemodynamic monitoring. Serial measurements of cardiac filling pressures (CVP, pulmonary capillary wedge pressure [PCWP]) and ventricular performance (CO/CI, SVR) are useful in monitoring CVP and PAWP’s trends during treatment. Approximately 50% of patients with septic shock will have reduced mortality and morbidity and lower organ dysfunction rates associated with adequate intravascular fluid resuscitation (Medeiros, Ferranti, Delgado, & Brunow de Carvalho, 2015). However, risk of mortality doubles per hour delay in restoring the blood pressure level to normal and improving perfusion (Han et al., 2003; Vincent et al., 1998). Patients may require increased fluid administration for multiple days due to capillary leak and leakage of the fluid from the vessels into the tissues (Brierly et al., 2009).
3. Antibiotic Administration. Broad spectrum antibiotics are administered with the initial dose ideally given with in the first hour of recognition (Dellinger et al., 2013). In one pediatric study, patients experienced less sepsis-related organ failure and decreased mortality when antibiotics were administered within 3 hours (Weiss et al., 2014). Blood culture specimens should be obtained before the administration of antibiotics, but antibiotics should never be withheld to obtain a culture. With the emergence of many resistant organisms, antibiotics may be given in accordance with regional resistant/sensitivity patterns. Antibiotic choice will also be influenced by the age, medical history, status of immune compromise of the child as well as the suspected source of infection.
4. Vasoactive Agents (Table 9.25). If volume resuscitation fails to restore perfusion or severe hypotension is present, vasoactive agents are indicated in order to maintain organ perfusion (Davis et al., 2017). Fluids should continue to be administered with vasoactive agents until clinical parameters are met. Vasoactive agents may be initiated and changed over time as the patient’s clinical condition evolves (Davis et al., 2017). Persistent shock following 40 to 60 mL/kg of fluid administered within the first hour is considered fluid-resistant shock and vasoactive agents are considered (Martin & Weiss, 2015). A mixed α- and β-adrenergic agent, such as epinephrine or dopamine, is considered first-line inotropic support for cold shock in children (Brierly et al., 2009, Martin & Weiss, 2015). If blood pressure continues to be decreased, inotropic agents are added. In the presence of hypotension, the drug of choice is epinephrine due to the preponderance of “cold shock” in children. If a child is in “warm shock,” norepinephrine may be more appropriate to increase SVR (Martin & Weiss, 2015). For patients with a normal blood pressure and myocardial dysfunction, dobutamine may be considered a first-line agent to increase contractility when there is a low CO state with high or adequate SVR (Brierly et al., 2009; Martin & Weiss, 2015). Norepinephrine is considered a first-line agent for a patient experiencing warm shock, considered with a high CO state and low SVR. Epinephrine may be initiated in the treatment of cold shock in 785the lower doses, primarily for its beta-2 adrenergic effects. Higher doses will provide more α-adrenergic effects. Central access is preferred for vasoactive agents, although current recommendations provide support for initial peripheral infusion during resuscitation. Vasopressors can be titrated to clinical parameters, such as CVP or urine output, with the intention to avoid the negative effects of excessive vasoconstriction (Brierly et al., 2009).
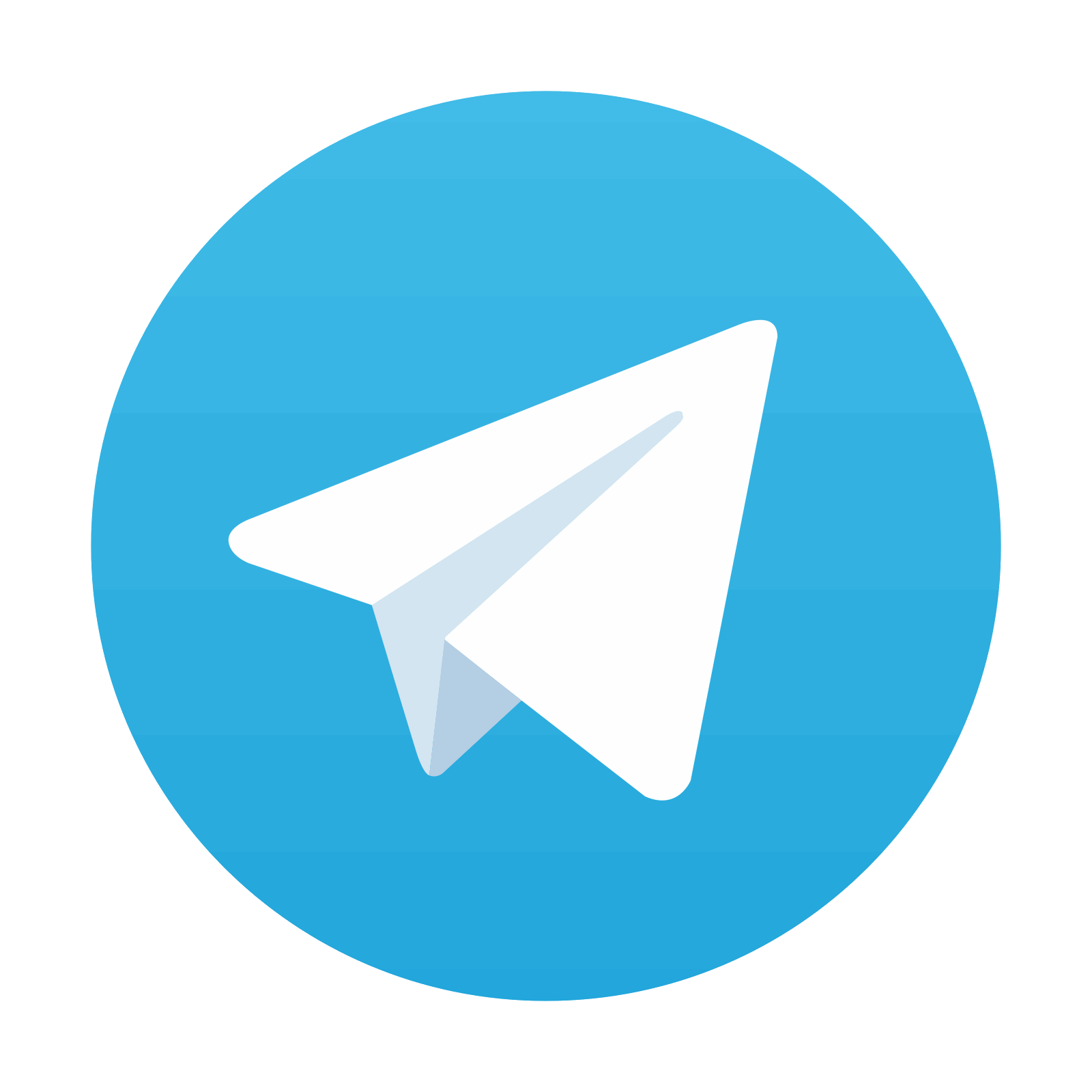
Stay updated, free articles. Join our Telegram channel

Full access? Get Clinical Tree
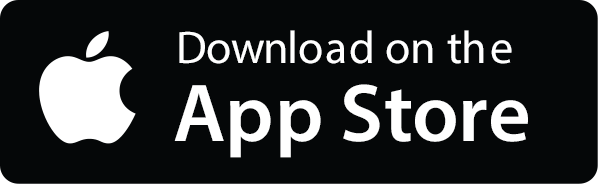
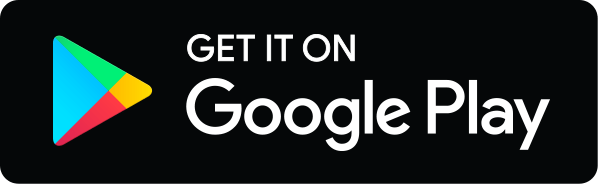
