SUBJECT KNOWLEDGE
• Mechanism of feedback loops in maintaining homeostasis
• Biological basis of thermoregulation, blood pressure maintenance, pulse and respiratory homeostasis
CARE DELIVERY KNOWLEDGE
• Measurement and assessment of body temperature
• Measurement and assessment of blood pressure
• Measurement and assessment of respiration
PROFESSIONAL AND ETHICAL KNOWLEDGE
• Accountability
• Consent
• Health promotion
PERSONAL AND REFLECTIVE KNOWLEDGE
• Ideas for portfolio and skills laboratory learning
• Case study consolidation
INTRODUCTION
La fixité du milieu intérieur est la condition d’une vie libre et indépendante.
Claude Bernard (1878–9)
(‘To have a free life, independent of the external environment, requires a constant internal environment’)
Claude Bernard was a pioneering physiologist working in the early 19th century. Through his various experiments and investigations he noticed that certain physiological values stayed within a very narrow range. He proposed that the body works hard through compensatory mechanisms to maintain certain key parameters such as oxygen and carbon dioxide levels, water and electrolyte balance, blood glucose, body temperature and blood pressure within an optimal range. This idea of a fixed internal environment is termed homeostasis. By maintaining homeostasis, the body can provide the best conditions possible for cells and organs to function. Illness can be seen to arise when there is a deviation away from the normal, one that the body cannot cope with or adapt to.
A change in internal environment creates an automatic response by the body which enables mechanisms to redress deviation from normal. Our biology returns to a point of homeostasis. In the same way, in everyday life we adapt to, and cope with, all of the problems and opportunities thrown at us by an ever changing world. Nursing theorists such as Betty Neuman and Sister Callista Roy see nursing as working with people to help them cope with or adapt to problems they encounter (Marriner-Tomey & Alligood 1998). These problems may be biological in nature, involved with thinking, emotions and beliefs, or may be social in nature. More often than not, however, problems span all three domains. There is thus a holistic view of health and nursing in which the nurse and client work together to achieve a sense of control and balance in the everyday life, and also optimal biological functioning.
As an example of homeostasis, this chapter will examine the ways in which the body responds in an attempt to meet the specific demands placed upon it by changing blood pressure, body temperature and respiration. The role of the nurse in monitoring temperature, blood pressure and respiration will be explored and related professional and ethical issues considered. As the content of this chapter focuses on these three specific areas of homeostasis, it is expected that you will cross-reference with many other chapters for other complementary aspects of homeostasis (e.g. Ch. 4, ‘Resuscitation and emergency care’, Ch. 15, ‘Skin integrity’, Ch. 8, ‘Nutrition’ and Ch. 9, ‘Stress, relaxation and rest’).
OVERVIEW
Subject knowledge
The mechanisms of feedback loops are explored. These are essential to maintaining homeostasis. The chapter will concentrate on how normal temperature, blood pressure and respiration are maintained and controlled by the body.
Individual behavioural, environmental, social and emotional factors also have an effect on how the internal body balance is maintained. These factors are explored under psychosocial and environmental subject knowledge.
Care delivery knowledge
The knowledge needed by the nurse for making a comprehensive assessment of temperature, blood pressure and respirations is explored. The nursing management of some common problems that threaten homeostasis are also included in this section.
Professional and ethical knowledge
Professional issues that influence the role of the nurse in the assessment of homeostasis are identified. The discussion includes an exploration of how the issues of consent, professional accountability and health promotion apply to the nurse’s role when undertaking observations and measurements.
Personal and reflective knowledge
Suggestions are made for portfolio development and experiential exercises in which assessment of homeostasis can be practised in a safe environment. Consolidation of knowledge gained from the chapter and through your practice experience is facilitated through case study work.
On pages 172–173 there are four case studies, each one relating to one of the branches of nursing. You may find it helpful to read one of them before you start the chapter and use it as a focus for your reflections while reading.
SUBJECT KNOWLEDGE
MECHANISMS IN HOMEOSTASIS
Before outlining specific knowledge needed for clinical decision making regarding body temperature, blood pressure and respiration, it is important to understand the internal mechanisms involved in maintaining balance in all body systems and also to consider potential external influences.
Before discussing control loops, it is useful to consider an everyday occurrence. Sian is running a bath for her toddler, Tom. She runs in hot and cold water and tests the temperature with her hand. If it is too cold she turns off the cold tap and runs in more hot, if it gets too hot she runs in more cold. Eventually she ends up with a bath full of water at the right temperature. What Sian has just done is an illustration of how the body controls all of our physiology through feedback loops. The elements of a feedback loop are:
1 Detector (Sian’s hand).
2 Sensory pathways (nerves in Sian’s arm and spinal cord).
3 An integrator to bring all the information together and make a response (Sian’s perception of whether or not the bath is too hot or too cold).
4 Pathways carrying the decision out (motor nerves in Sian’s spinal cord and arm).
5 An effector to put into effect the decisions of the integrator (Sian’s hands that turn on and off the taps).
This situation described is an example of negative feedback. In negative feedback, the response that is triggered by the detector negates the deviation from the desired point. Negative feedback loops are the most common mechanism for controlling body functions. Positive feedbacks tend to amplify the trigger rather than reduce it, thus moving the body further away from homeostasis. They are rarely found in healthy individuals (Seeley et al 2008). However, many diseases are characterized by positive feedback, including rheumatoid arthritis, asthma and heart failure. Positive feedback also has a role to play in mental health settings; panic attacks and phobias have elements of positive feedback, where, for example, awareness of sweating and a pounding heart increase anxiety levels even further.
The hypothalamus is a key control and integration centre in the brain and is part of the limbic system, which helps to maintain homeostasis. The hypothalamus regulates eating and drinking, sleeping and waking, body temperature, hormone balances, heart rate, sex drive and emotions (Seeley et al 2008). It also directs the master gland of the brain – the adenohypophysis (pituitary gland) – which is responsible for regulating hormonal balance within the body. The brain is therefore a major organ of adaptation and can respond to changes in the external and internal environment quickly and flexibly to maintain health (Shmaefsky 2007). How homeostasis is specifically maintained is the major focus of this chapter, but although homeostasis is concerned with the stability and maintenance of internal physiological systems, it is also important to consider such balance as being within a context of some external influence.
EXTERNAL INFLUENCES ON HOMEOSTASIS
Psychosocial, cultural, economic and environmental factors can affect homeostasis and need to be considered. Influences on behaviour are individual to each person, but are usually associated with the family and school in childhood, peers in early adolescence, and social groupings, religious beliefs, the media and multiple other sources in adult life. The beliefs of society are influential and how individuals become ill or remain healthy is affected by cultural beliefs on what is good and bad ‘healthy’ behaviour. In the past, many sociologists have commented on the ‘lay’ perceptions of health as opposed to how professionals describe, label and classify ‘ill-health’ (Kleinman, 1980, Mishler, 1981 and Helman, 1994).
Understanding differences in perception can be important in deciding how to approach individuals and groups when involved in health promotion activities. Cultural beliefs can also lead to misunderstandings and may even be a threat to health. An example would be using a hot water bottle during the shivering phase of a fever for a young child aged between 6 months and 3 years. Besides the danger of burning the skin, the child’s temperature may rise rapidly, leading to an increased risk of febrile convulsion.
Health can also be detrimentally affected by environmental factors, which include political and economic factors over which individuals have limited control. Correspondingly, government legislation and policy can be influential in controlling situations where detriment to health is likely (see Ch. 3, ‘Safety and risk’).
In order to cope and adapt to illness and life problems, people need resources. This is another way the government can influence a person’s level of wellness, through controlling what services and resources are available to people to help them overcome or live with their health problems. So, for example, the Scottish Executive introduced free personal and nursing care in 2002, and the Welsh Assembly Government introduced free prescriptions for all in 2007. Both of these policies are likely to have dramatic effects upon people’s abilities to maintain their own health and well-being.
Government economic policy has also had a direct impact on individuals in society. It could be argued that imposing a tax on fuel and limiting extra payments to the elderly and disabled until certain low temperatures have been reached over consecutive days has little impact overall on reducing morbidity and mortality due to hypothermia during the winter months. Research in this area is fraught with difficulties as hypothermia is often not the main recorded cause of death (Watson, 1996 and Age Concern, 2008); however, the Department of Health (2001a) suggest that there are about 80 000 cold-related deaths each year in the UK. Although health promotion may be addressed at the levels of behaviour and lifestyle, it may still be the cost factor that predominates in an individual’s ability to keep warm in winter.
A more recent concern is that of the health effects of climate change in the UK (Department of Health 2001a). It is anticipated that there will be a gradual fall in cold-related deaths and an increase in heat-related deaths due to global warming over the next few decades.
During a nine day heat-wave from 4–13 August 2003, there were 2091 more deaths than expected in England; likewise there were 1311 more emergency hospital admissions than expected, with the over 75 age group suffering particularly badly (Office of National Statistics 2006). This kind of statistic has clear implications for nursing and the management of resources in the future.
Whether a person’s health status is considered as an individual matter, a disturbed internal biology or a mental health problem is continuously debated. In some respects it is simpler to take this view as the individual’s health status is focused clearly as their own personal concern and can be identified as their problem when health is poor. However, by considering people as interacting with the world they live in, we can see that local, national and even international factors often have a role to play in determining a person’s health status. Nurses must be cognizant of these debates, for they can respond in a number of ways. On an individual basis, nurses may need to help a person cope with, or adapt to the challenges these factors present in their lives, while on a wider scale the role of the nurse in advocating for patient communities is relevant.
THE BALANCE OF BODY TEMPERATURE
The main organ for maintaining normal body temperature (thermoregulation) is the skin. The skin has many vital functions. However, in this particular section, thermoregulation and its homeostatic control will be the main focus (see Chapter 15 for other functions of the skin).
Humans are homeothermic and strive to maintain a core temperature within a narrow range of 36–38 °C despite the day-to-day fluctuations encountered as a consequence of environmental temperatures and our own metabolic activities. Homeothermy is dependent upon continual thermoregulation with the scales finely balanced between heat production and heat loss. We need to maintain a body temperature of around 37°C because this is the optimal temperature for our metabolism and enzyme systems to function at. Deviation above or below the normal range of body temperature will have increasingly severe consequences for our health and will eventually lead to death (Montague et al 2005).
Heat production occurs in two ways. Firstly, muscle contraction generates large amounts of heat as a waste product. This excess heat is removed from active muscles and distributed around the body by the circulatory system, using blood as a heat transfer medium. If we begin to feel cold we naturally increase our level of activity, almost unconsciously, and might even start to shiver to increase heat production. If we are not exercising – resting on the sofa watching television, for example, or asleep – heat is generated through metabolism. The liver is highly active metabolically and generates a large amount of heat that can be distributed around the body via the bloodstream.
The general level of metabolic activity in the body is chiefly governed by the hormone thyroxine, and levels of this hormone increase when we are cold to increase heat production (Martini 2006). This mechanism is particularly important in young babies who may not have developed the ability to shiver.
Four major physical processes are involved in the loss of heat from the skin to the environment:
• evaporation
• conduction
• radiation
• convection.
Each of these processes is important to understand when managing the care of patients and clients.
Evaporation (22% of heat loss)
Large amounts of heat energy are needed to convert liquid water into water vapour, a process known as evaporation. If you wet your hands the liquid water strips heat out of the tissues of your hand and uses this heat energy to turn from liquid to vapour. We experience two things: firstly, our hands chill and secondly, they dry. Sweat is composed mainly of water and as it evaporates the energy used in the process of evaporation cools the body. Water is constantly being lost from the body; insensible water loss (i.e. too small or gradual to be perceived) is approximately 500 mL/day as a result of evaporative loss from the skin and the respiratory passages. This loss can be increased as a result of exercise or sweating. This is why on a hot day the cooling of the body through cool sponges may be soothing. In nursing the use of cooling liquids to reduce temperature is recognized with some caution. In children, whose surface areas are large, there is a risk of too rapid cooling leading to discomfort and in most patients there is a risk of shivering, which is counterproductive as it is a mechanism which generates heat.
Conduction (3% of heat loss)
In conduction, heat loss or gain is brought about by contact between the surface of the skin and some other object that is either warmer or cooler, for example jumping into a hot bath after being outdoors in the winter for 2 hours in the freezing cold, or having a lovely chilled drink on a hot day. Heat loss to cold objects can be decreased by insulation and clothing, for example wearing thick socks and boots when walking in snow.
Radiation (60% of heat loss)
Radiation is a process whereby heat is transferred from one heat source to another via infrared radiation without direct contact. For example, when you are cold it is possible to gain heat by standing in the sunshine. The human body also loses heat via radiation. The amount of heat lost through radiation is directly proportional to the amount of skin exposed. Babies and small children have a relatively large skin surface area when compared to their size so they tend to lose heat rapidly via radiation. Conversely, they can also be re-warmed by radiation too, and baby resuscitation equipment often includes overhead infrared warming lamps.
Convection (15% of heat loss)
As air moves over the skin heat is lost through convection. When there is little movement of air, the still air forms an insulating barrier and reduces the amount of heat lost; when air movement increases heat loss also increases. Fleecy clothing works by trapping a warm layer of air against the skin, thus reducing the heat lost by convection. The use of electric fans to cool clients with fevers is an example of this principle.
Homeostatic control of body temperature
Body temperature is regulated by a feedback loop. As discussed above, heat is constantly being produced by metabolic activity. In order for this heat production to become and remain homeostatically balanced, there has to be a heat loss of the same proportion. This is obtained by balancing the scales between heat input and heat output (Fig. 7.1).
![]() |
Figure 7.1 |
Physiological response to low temperatures
Thermoreceptors located in the periphery and central nervous system respond to a lowering of temperature and transmit impulses to the preoptic area of the brain, located in the hypothalamus, stimulating the heat promoting centre. As a consequence there is sympathetic nervous system stimulation resulting in vasoconstriction of the blood vessels in the skin, and shivering is increased (Fig. 7.2). The sympathetic nervous system response also triggers the release of epinephrine and thyroxine, both of which increase metabolic activity throughout the body, thus increasing heat production.
Sympathetic stimulation resulting in the release of epinephrine and thyroxine increases the basal metabolic rate by mobilizing the fat stores from adipose tissue and as a consequence generating heat. This process is known as chemical thermogenesis or non-shivering thermogenesis, and its function is vital in the newborn because their heat loss is large due to their large surface area, and in the early years the shivering response is poorly developed. This adipose tissue is called ‘brown fat’ and humans have only a small quantity and can therefore only raise their body heat production by 10–15%.
We also see goose bumps developing. In mammals and birds this response results in raising the fur or feathers to trap a layer of warm air against the body. As humans are largely hairless this mechanism has little effect in heat conservation and is largely redundant.
The other important set of responses to low temperatures are the non-physiological behavioural responses such as finding shelter, dressing in warm clothing, turning up the heating in a house. These factors are important to consider as it is often a failure of behaviour rather than a failure of physiology that results in unplanned and life-threatening heat loss.
Physiological response to high temperatures
A rise in temperature is recognized by peripheral and central thermoreceptors; they relay this information to the preoptic area of the hypothalamus and the heat losing centre is stimulated. As a result, there is parasympathetic stimulation; cutaneous vasodilatation, diaphoresis (sweating) and decreased metabolism ensue and heat loss is increased. Shivering is inhibited.
In addition to the physiological mechanisms, nurses must remember the important role played by a person’s behaviour. Cooling behaviours include taking off clothes, seeking shade and a cool environment, drinking fluids, and active cooling, for example jumping in a swimming pool.
Physical factors that affect thermoregulation
Thermoregulation failure
Under certain conditions the normal thermoregulatory mechanisms can be compromised. These include:
• fever (pyrexia)
• hyperthermia
• hypothermia.
Fever
Fever (pyrexia) is an elevation in body temperature up to 41°C. It can be caused by inflammation as a result of pyrogen/interleukin-1 released in response to the invasion of the body by microorganisms or other foreign substances (Seeley et al 2008). This initiates an upward displacement of the hypothalamic ‘set point’ for temperature control. As the set point has been raised the body responds by increasing temperature to this new level. We usually associate fever with infection and it cannot be denied that a fever is one of the signs of infection. However, any large scale tissue damage within the body will result in an inflammatory reaction and hence a rise in body temperature. You might see this in clients following major surgery, or following a myocardial infarction, where a spike in temperature might be seen in the first 24–36 hours after the event. A rise in temperature is said to help the body’s immune responses eliminate the pathogens. In addition, metabolism in all cells is increased as their temperature rises; thus healing and repair processes are accelerated.
Hyperthermia
This is caused by a failure of the thermoregulatory system. The body becomes dangerously overheated when there has been prolonged exposure to heat (often as a result of high ambient air temperatures); exercise in the heat is usually a contributory factor, as is high humidity and a failure to maintain fluid intake.
In the early stages loss of energy, cramps in the legs, arms or back with associated fatigue, dizziness and irritability emerge. As the body temperature rises further, more serious neurological and mental disturbances occur such as fitting or periods of unconsciousness. Due to the loss of large volumes of fluid as sweat there may be circulatory collapse with the client showing signs of hypovolaemic shock. As the temperature rises even higher there is a large reduction in sweating and coma usually occurs as the core body temperature approaches 42 °C (Montague et al 2005).
Heat exhaustion and heat stroke are sometimes defined as different stages of hyperthermia. However, such a distinction is usually arbitrary and difficult to define, and the treatment for both conditions follows the same principles (controlled cooling, rehydration, protection from the environment). There is also a risk that some people’s condition may be seen as not that serious – ‘it’s only heat cramps’. All clients with hyperthermia require expert care and attention.
Hypothermia
The effects of hypothermia vary with the speed of onset and how far the temperature falls. At 35°C there may be shivering, cold, pale, dry skin and apathy. A fall of body temperature to below 34°C causes mental confusion and sluggishness and further reductions result in dysfunction of the thermoregulatory system. Shivering in an attempt to gain heat ceases and loss of consciousness results. Muscle rigidity follows with associated cardiac dysrhythmias and eventual death (Holtzclaw 1993, cited by Montague et al 2005). The elderly, the very young and homeless people are particularly vulnerable during the winter months in the UK.
Alcohol and drugs can exacerbate hypothermia. The role of drugs and alcohol in causing hypothermia cannot be underestimated; it is quite possible to develop profound hypothermia in mid-summer by ingesting a cocktail of alcohol, street drugs and prescription medications either for pleasure or with the intention of suicide.
Children have a protective mechanism that is triggered if they fall into cold water. This response is referred to as the dive reflex, and results in a decreased heart rate and an increased cerebral and cardiac blood flow. As children are small in size they have a relatively smaller circulating blood volume, which means that the cold water in the lungs rapidly chills the blood in the pulmonary circulation which then results in brain cooling and a reduction in the brain’s oxygen (O2) requirements. Consequently, a child may survive immersion in cold water for longer periods of time than an adult.
THE CONTROL OF BLOOD PRESSURE
Blood pressure is usually recorded as a pair of figures, one higher than the other. Systolic blood pressure (the upper value) reflects the maximum pressure produced by the left ventricle during contraction, or systole. Diastolic blood pressure (lower value) represents the pressure in the artery at the end of relaxation of the left ventricle, known as diastole. The range of normal blood pressure (normotension) varies according to age (Table 7.1).
Age (years) | (mmHg) Systolic | Diastolic |
---|---|---|
Newborn | 80 | 46 |
10 | 103 | 70 |
20 | 120 | 80 |
40 | 126 | 84 |
60 | 135 | 89 |
Factors contributing to a change in blood pressure
Blood pressure is needed to drive blood around the circulatory system, ensuring delivery of oxygen and nutrients and the removal of wastes. This is known as perfusion. Blood flow varies in different regions of the body and in different organs; thus we might talk of renal perfusion or cerebral perfusion. The body uses a range of compensatory mechanisms to maintain adequate blood pressure and hence perfusion. If the needs of an organ or region of the body increase then the delivery of blood to that area will also have to increase to meet the needs.
The pumping action of the heart is one of the main factors that generates blood pressure. The speed with which the heart contracts each minute (heart rate) multiplied by the amount of blood it expels per contraction (stroke volume) is referred to as the cardiac output (i.e. cardiac output = heart rate × stroke volume), and should this increase, a corresponding increase in blood pressure will be observed (Seeley et al 2008).
Another factor that must be borne in mind is the degree of friction created when blood travels rapidly through the blood vessels. This is referred to as peripheral resistance. The widening (vasodilatation) or narrowing (vasoconstriction) of a blood vessel will result in either a reduction or increase in this friction of blood against the walls of the blood vessels and, as a consequence, a decrease or increase in the blood pressure. A division of the autonomic nervous system called the sympathetic nervous system regulates the size of blood vessels. The middle layer in the arteriolar wall, called the tunica media, is in a state of partial contraction as a result of continual activity by the sympathetic division of the autonomic nervous system. This is referred to as sympathetic tone and the tone derives from a group of cells in the vasomotor centre within the medulla oblongata in the brain. An increase or decrease in vasomotor centre (VMC) activity will lead to a corresponding increase or decrease in blood pressure (Fig. 7.3).
Using your biological sciences textbook, review the effects on the homeostatic control of blood pressure of:
• The baroreceptor control system.
• A drop in blood flow through the kidney (renal perfusion).
Review the relative effects of each of these mechanisms when:
• You get patients up suddenly from a lying position to a standing position and they complain of feeling dizzy and faint.
• A victim of a stabbing loses a large amount of blood.
![]() |
Figure 7.3 |
THE PULSE
Contraction of the left ventricle forces blood into the aorta and as a result creates distension and elongation in the arterial wall. As a consequence of this distension, the wave passing along an artery can be felt whenever it is pressed carefully against a bone in places such as the wrist (radial and ulnar pulses), ankle (posterior tibial pulse), neck (carotid pulse) and groin (femoral pulse) areas (Fig. 7.4). In babies less than 1 year of age heart rate is measured by listening with a stethoscope to the apex beat. This is defined as the heart beat which is heard at the apex or lower tip of the heart. The nurse listens for the characteristic ‘lub-dub’ sounds and counts each full cardiac cycle for 1 minute to determine the apical rate (Lewis & Timby 1993). In young children the brachial pulse is the most common site for measurement of pulse.
![]() |
Figure 7.4 (from Montague et al 2005, with kind permission of Elsevier). |
When trying to establish information about a patient’s pulse, it is important to consider three factors:
• rate
• rhythm
• strength of the pulse wave.
Pulse rate
Pulse rate (Table 7.2) indicates the speed with which the heart is beating, and it varies with age. A rapid pulse is called a tachycardia and may be triggered by excitement, anxiety or exertion; however, it is also indicative of problems such as haemorrhage or fever. Conversely, a slow pulse is referred to as bradycardia. In health, a well-trained athlete may exhibit bradycardia because their heart muscle is very efficient. Bradycardia can be indicative of heart and neurological disease and is also caused by stimulation of the parasympathetic nervous system.
Age | Approximate range | Approximate average |
---|---|---|
Newborn | 120–160 | 140 |
1 month to 12 months | 80–140 | 120 |
2 years | 80–130 | 110 |
2 years to 6 years | 75–120 | 100 |
6 years to 12 years | 75–110 | 95 |
Adolescence | 60–100 | 80 |
Adulthood | 60–100 | 80 |
Pulse rhythm
The normal pulse is regular and the time between each beat is constant. If the normal rhythm of ventricular contraction alters, the interval between beats is interrupted. This can be due to a heart beat occurring earlier (premature) or later or a missed beat. An irregular pulse pattern is called an arrhythmia or dysrhythmia. Some dysrhythmias are there all the time and are continuous; others come and go and are intermittent, and may be triggered by certain events, or even something as simple as a cup of coffee! Sinus arryhthmia is a change in rhythm more commonly found in children and is associated with an increase in heart rate on inspiration and a decrease on expiration (Montague et al 2005). Other changes are often associated with cardiovascular disease and can be life-threatening if cardiac output is seriously affected (see Ch. 4, ‘Resuscitation and emergency care’).
Pulse strength
Pulse strength can be separated into two important factors:
• volume
• tension.
Pulse volume is the degree of distension felt in the arterial wall in response to the pressure wave exerted on ventricular contraction. It usually relates to the amount of blood pumped out with each contraction.
An altered pulse volume is often described as being:
• Weak and thready – here the pulse is difficult to feel and disappears if slight pressure is exerted.
• Bounding and full – here the pulse is easy to feel; it is pronounced and strong and does not disappear with moderate pressure (Torrance & Elley 1997).
Tension is specifically related to blood pressure. More force is needed when compressing the artery to feel for a pulse when the blood pressure is high. Conversely, less force is required when the blood pressure is low. When automated equipment is used to measure the pulse rate, such as a pulse oximeter, it is important to manually check rhythm and strength as the equipment will not detect this.
In the practice laboratory:
• Measure and comment on the rate, rhythm and strength of the pulse rate of your peer group.
• Note any differences related to age and other factors such as recent exercise.
In your practice placements:
• Observe the different sites for taking the pulse in different client groups.
• Under supervision, practise taking the pulse of individual clients and note any changes and the reason for those changes in rate, rhythm and strength.
RESPIRATORY HOMEOSTASIS
This section is concerned with the physiology of breathing and gaseous exchange, and the way that respiratory and cardiovascular systems work together to maintain homeostasis.
The balance of respiration
The primary purpose of respiration is to supply the cells of the body with oxygen (O2) and remove carbon dioxide (CO2). The three basic processes involved are:
• Pulmonary ventilation (otherwise known as breathing), which is concerned with air passing into (inspiration) and out of (expiration) the respiratory passageways in an exchange with the atmosphere.
• External respiration, which refers to the exchange of respiratory gases between the lungs and the blood.
• Internal respiration, which is the exchange of respiratory gases between the blood and the cells of the body.
The process of pulmonary ventilation relies upon two characteristics of gases such as air. Firstly, gases move from areas of high pressure to areas of lower pressure along pressure gradients. Secondly, there is an inverse relationship between volume and pressure – i.e. if you increase the volume the pressure goes down and if you decrease the volume of a fixed amount of gas the pressure rises. This universal property of gases is termed Boyle’s law (Cree & Rischmiller 2001). In the respiratory system there is a continuous movement of air into and out of the lungs along pressure gradients. Upon inspiration, contraction of the diaphragm and the intercostal muscles cause the volume of the chest cavity, and hence the lungs, to increase. This causes the pressure inside the lungs to drop below atmospheric pressure. This leads to a movement of air from the atmosphere to the lungs during inspiration. However, on expiration, when the diaphragm and the intercostal muscles return to their normal position, the pressure gradient is reversed and air moves out of the lungs.
Once the tissues of the body have used the O2 it is essential not only that the waste product CO2 is removed, but also that O2 concentrations are replenished. Gas exchange in the lungs is the process whereby there is a movement of O2 into the blood in the pulmonary blood capillaries, while CO2 moves outwards into the lung alveoli. This movement of gases is brought about by their movement along concentration gradients; this is where gases move by diffusion from areas of higher concentration to areas of lower concentration (Cree & Rischmiller 2001). In mixtures of gases, concentration is often measured as partial pressure in mmHg or kPa rather than in grams or litres. Figure 7.5 refers to the partial pressures of O2 (pO2) and CO2 (pCO2 ). Because the pO2 in the alveoli is greater than in the pulmonary blood vessels, O2 moves down the concentration gradient. The diffusion of O2 from alveoli to blood capillaries allows deoxygenated blood to be converted to oxygenated blood. The diffusion of CO2 from the blood capillary to the alveoli works on exactly the same principle as O2 diffusion. Due to the relatively high partial pressure of CO2 (pCO2) in the pulmonary blood capillary and the low pCO2 in the alveoli, CO2 diffuses down its concentration gradient (see Fig. 7.5).
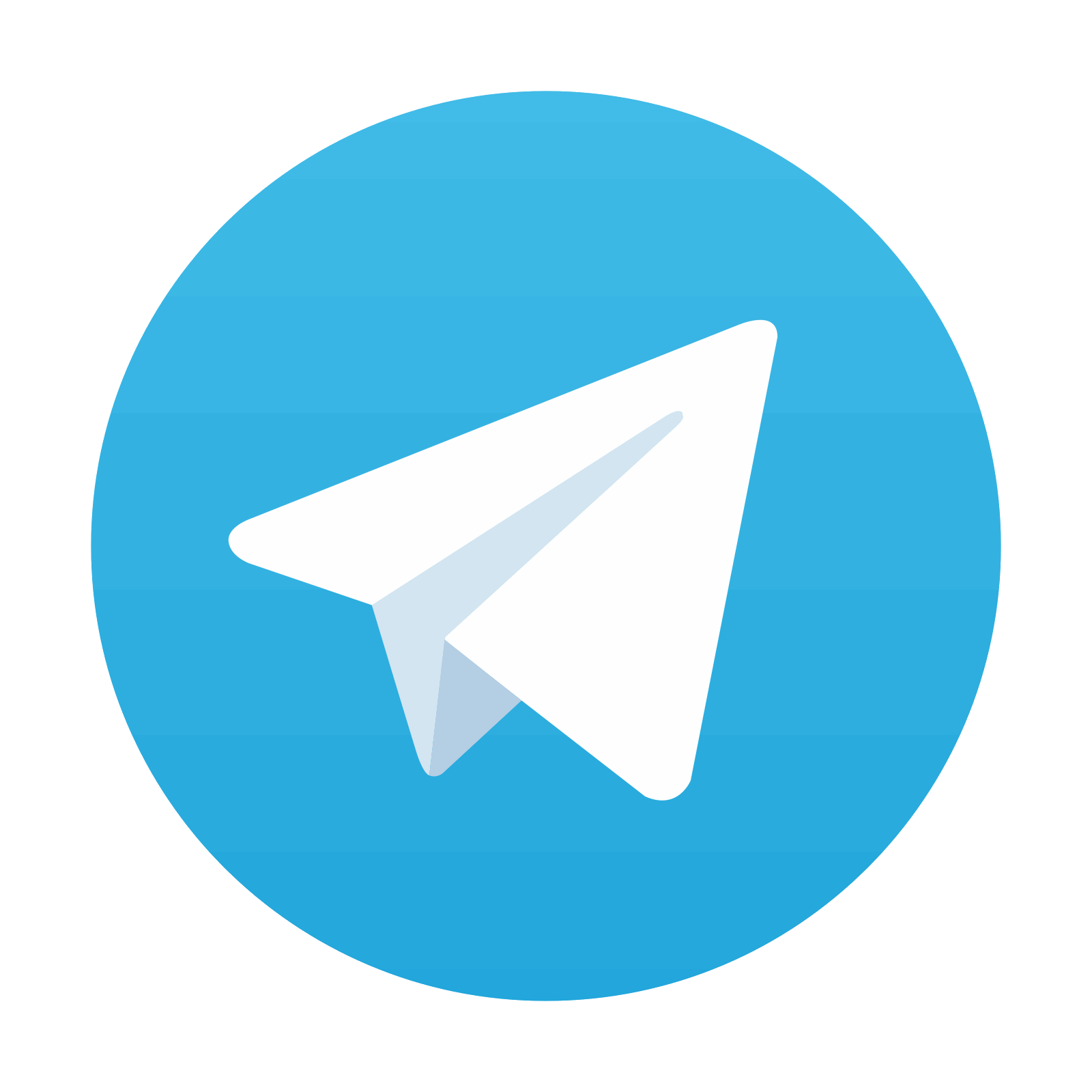
Stay updated, free articles. Join our Telegram channel

Full access? Get Clinical Tree
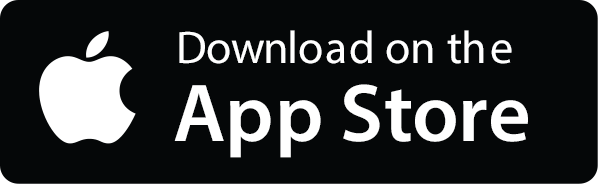
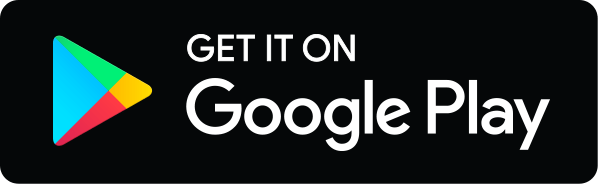