Chapter 12 Haematological Health Breakdown
When you have completed this chapter you will be able to
INTRODUCTION
This chapter presents a review of the anatomy and physiology of blood and haematopoiesis, including components of plasma, blood cell types, haemostasis, and blood groups. Using a case-study approach, two general categories of common blood and haemopoeitic health breakdowns are then presented. These are red blood cell and coagulation disorders. Issues related to dysfunction of white blood cells or bone marrow, are discussed in Chapter 14 (see sections on leukaemia and myeloma).
HAEMOPOEITIC SYSTEM OVERVIEW
Blood is a viscous (sticky) fluid and its red colour is familiar to most people. The main reason blood is viscous is because it is not strictly true simply to call it a fluid. It is fact a liquid (plasma) with cells suspended in it (which is why blood is classified as a connective tissue). This can be demonstrated easily by taking a sample of blood, adding some heparin to it to prevent it from clotting and then spinning it in a centrifuge. After spinning a test tube of blood for a few minutes it can be seen that it separates into two parts. The top of the test tube will be seen to contain a yellowish fluid – the plasma, while at the bottom of the tube will be seen a solid red mass which can be demonstrated to be composed of cells. If one looks carefully at the test tube it will be seen that the plasma occupies approximately 55% of the total volume, and the cells approximately 45% of the total volume1. The measurement of of the percentage of cells to plasma is the haematocrit (Hct). The cell mass may also have a very thin top layer (less than 1% of the total volume) which consists of white blood cells (WBCs), whilst the rest of the cell mass will be red blood cells1,2.
These red blood cells (erythrocytes; RBCs) account for 99% of cells in the blood. They are non-nucleated; biconcave discs approximately 7 μm in diameter. This shape gives the red cell a larger surface area to volume ratio, which enhances cell flexibility and ensures minimal membrane tension during volume changes, when the cells give up oxygen in the tissues and take it up in the lungs1,2. This flexibility enables the RBC to elongate when passing through capillaries, further increasing surface area for gas transport. Repeated deformation contributes to the limited life of erythrocytes.
Red cell production (erythropoiesis) occurs in red bone marrow in adults; this is usually confined to the sternum (breastbone) and the iliac crest (hipbone). In children nearly all long bones contain red bone marrow. In the fetus red cell production also occurs in the liver and spleen. As children reach maturity red bone marrow is gradually replaced with yellow bone marrow until in the adult only the two sites of red cell production noted above remain. However, in times of need, for example following an accident with loss of blood, some yellow bone marrow may convert back to red marrow to assist in the replacement of lost red cells1,2.
This condition is sometimes encountered in people who have chronic obstructive airways limitation (CAL), (see Chapter 6). Some essential dietary requirements for red cell production are iron, folic acid and vitamin B121,2.
Because red cells have no nucleus they have a limited lifespan. Generally mature cells survive in the blood for up to 120 days. Aged red cells are extracted from the blood by macrophages of the reticuloendothelial system (i.e., macrophages in red bone marrow, liver, spleen, lymph nodes and loose connective tissue) and broken down. Globin is degraded into amino acids (which are recycled) while haem is converted to bilirubin (transported to the liver and excreted in bile) and iron is also recovered and recycled1,2.
Agranulocytes are divided into two groups – lymphocytes and monocytes. Lymphocytes may be small (7–9 μm) or large (8–16 μm) in diameter. Lymphocytes have a large dense nucleus and play a key role in humoral and cell-mediated immunity (see Chapter 13). Monocytes are the largest blood cells and are referred to as tissue macrophages (i.e., they have a largely phagocytic function1,2).
Thrombocytes (platelets) are also contained in the blood and are really small pieces of cytoplasm, 2–3 μm in diameter. They are derived from large cells in the red bone marrow called megakarocytes, and play an important role in the clotting of the blood1,2.
Plasma forms approximately 55% of the blood. Its composition is 90% water, 8% protein, and 2% organic compounds and ions. As plasma is mainly water it carries many water-soluble substances around the body. The plasma also contains ‘plasma proteins’ – albumin, globulins and fibrinogen. Albumin is the most abundant of the plasma proteins (40g/L), is produced in the liver and is a major contributor to the colloid osmotic pressure of blood. Albumin carries (in loose combination) hormones, bilirubin, fatty acids, bile salts, heavy metals and some drugs. It also buffers H+ ions and transports a small amount of CO2. Alpha-globulin (α-globulin) and beta-globulin (β-globulin) are also made in the liver and they transport various hormones, vitamins and minerals. Gamma-globulin (γ-globulin; also called immunoglobulins – some of the β-globulins are also immunoglobulins), are made in plasma cells. There are five types and each has a different function in immunity. Fibrinogen is also made in the liver. This is a large protein (the largest of all the plasma proteins) which is converted to insoluble fibrin during the clotting process1,2.
HAEMOSTASIS
Tissue injury results in the release of products that cause local vasospasm and constriction of smooth muscle in the surrounding vessels resulting in an initial reduction in blood flow to the injured area. This compensatory mechanism lessens blood loss and assists in coagulation or clotting of the blood1,2. Injury also exposes collagen fibres in the sub-endothelial tissue, resulting in adherence of platelets and activation of several clotting factors. After adhering to the collagen, platelets release a number of substances: serotonin 5-HT (causes further vasoconstriction); ADP (which causes platelet aggregation and starts the process of formation of a plug at the site of the vessel damage); and thromboxane A2 (which also enhances platelet aggregation and vasoconstriction). Aspirin inhibits the synthesis of thromboxane1,2.
There are two paths by which coagulation occurs – the extrinsic and intrinsic pathways. Normally both pathways are required for haemostasis. The extrinsic pathway is activated principally by tissue factor (thromboplastin; factor III) and thromboxane A2, but may involve other factors that are released by damaged tissue. The intrinsic pathway is activated by substances ‘intrinsic’ to the vascular system when there is abnormal exposure of collagen fibres in the endothelial layer of the damaged vessel. Each pathway begins in a different way, but both lead to the final common path where the conversion of an active precursor prothrombin (factor II) to thrombin, and then thrombin to soluble fibrinogen (factor I) results in fibrin formation and coagulation1,2.
Some important points to remember about coagulation are that:
BLOOD GROUPS
Blood transfusion was attempted many times in the past. Sometimes it was successful but more often resulted in the death of the recipient. Why? Well, it is now common knowledge that there are different blood types and that donor and recipient blood have to be compatible for a successful transfusion. Incidentally, if you stop and think about it, a blood transfusion is a type of organ transplant and was indeed the first successful organ transplant1,2.
Our modern system of blood typing (or grouping) dates back to the work of Landsteiner who in 1900 showed that all human blood could be divided into four groups on the basis of two antigens – A and B – present on the surface of the red cell. He further showed that there were also two antibodies corresponding to these antigens, present in the plasma – α and β. Thus based on this system four blood groups are possible (see Table 12.1)1,2.
Group | Red Cells (Antigen) | Plasma (Antibodies) |
---|---|---|
A | A | β |
B | B | α |
AB | A and B | – |
O | – | α and β |
The incidence of these blood groups in the general population in Australia and New Zealand is A (38%), B (10%), O (49%), and AB (3%)3,4. There are however racial variations. For example, indigenous peoples have higher proportions of group A and lower proportions of group B3,4, and group AB is more common in Asian people1,2.
Donor cells must be compatible with the recipient’s plasma. A little thought will show that it is possible to transfuse blood between groups safely as illustrated in Table 12.21,2. Thus, group O are ‘universal donors’ and group AB are ‘universal recipients’.
When incompatible blood is transfused the antibody(ies) present in the plasma react with the antigens on the red cell surface and cause them to agglutinate (stick together), lyse (break up) and thus liberate haemoglobin into the circulation. This causes kidney damage and death1,2.
Since 1900 many other antigens and antibodies which affect compatibility have been discovered. There are now at least fifteen well established blood group systems. The most important of the other antigens is a red cell antigen D – otherwise known as the Rhesus factor (it was first discovered in the Rhesus monkey). Individuals who possess the D antigen are said to be Rh positive (85% of the general population); those who do are Rh negative (15% of the general population). There is no natural antibody to D but if an Rh− person is given Rh+ blood they will develop antibodies and any subsequent transfusion with Rh+ blood will result in an incompatibility reaction1,2.
If an Rh− woman carries an Rh+ fetus then at birth some of the baby’s red cells may infiltrate the mother’s circulation. If this happens she will develop D antibodies. If she carries a subsequent Rh+ child, those antibodies will cross the placenta and will cause an incompatibility reaction in the fetus. The baby may die in utero, or may be born severely jaundiced (from excessive bilirubin due to the breakdown of haemoglobin from lysed red cells)1,2. Women who are Rh− can be immunised to prevent Rh sensitisation.
In about 80% of people the A and B antigens are also present in secretions like tears, saliva, sweat, semen and breast milk. These people are cal,led ‘secretors’; the remaining 20% of the population are known as ‘non-secretors’. Thus it is possible to classify secretors into ABO groups, a matter of great importance in forensic science1,2.
RED BLOOD CELL DISORDERS
ANAEMIA
Anaemia is a term that describes a decrease in or a dysfunction of red blood cells (RBC). Anaemia can be an acute or chronic condition. There are several causes of anaemia which can be classified as: a) RBC loss without RBC destruction; b) deficient RBC production; c) and increased RBC destruction over production5,6. Table 12.3 lists the common causes of anaemia)5,6.
Common Causes of Anaemia | |
RBC | |
Depressed RBC production | |
Increased RBC destruction over production (haemolytic anaemias) |
WHAT IS THE PATHOPHYSIOLOGY?
Homeostatic mechanisms normally ensure that the cardiac output delivers enough oxygen-carrying blood to the tissues to maintain normal functioning. The processes of ventilation, respiration and cardiac output are discussed more fully in Chapter 5 and Chapter 6. At rest, the body requires 250 mL of oxygen per minute to maintain normal functioning. Under normal conditions a cardiac output of 5 L/min maintains the required level of 250 mL of oxygen per minute more than adequately (in a young male adult, the standard for discussing human physiology)7. A less than adequate supply of oxygen leads to tissue hypoxia and hypoxaemia (reduced oxygen saturation). The kidneys play a vital role in the detection of hypoxaemia. The renal peritubular interstitial cells are sensitive to blood oxygen concentration and in response to hypoxaemia, produce erythropoietin (EPO). In combination with other factors, EPO increases RBC production by stimulating the bone marrow to produce pre-erythrocyte cells (reticulocytes)2,7. The number of RBCs per cubic millimetre of blood can be counted, and along with the haematocrit or packed cell volume, provides an indication of the numbers of RBCs in the circulation2,7.
Iron deficiency anaemia is the most common anaemia globally5. As noted earlier, iron is a component of haemoglobin and is essential for an optimal oxygen-carrying capacity by RBCs. A full blood count (FBC) would show microcytic (small cell) and hypochromic (pale colour) RBCs with a decreased mean cell volume5. Deficiency in either vitamin B12 or folic acid leads to megaloblastic (large cell) anaemias. These chronic forms of anaemia would show large RBCs, a very low haemoglobin, but possibly with a normal haematocrit due to the large size of red cells5.
Haemolytic anaemia (increased destruction over production of RBC) may occur as a result of internal (e.g., immune disorders) or external factors such as chemical (e.g., certain medications); physical (e.g., excess heat as in severe burns); or infectious agents (e.g., bacterial toxins, malaria)5,6. Sickle cell anaemia is an inherited haemoglobinopathy6, which demonstrates sickle-shaped RBCs. This mishape impairs the RBC ability to adsorb oxygen and the RBC are seen as damaged which leads to clumping of the sickle cells and possibly a vascular occlusion can occur. This occlusion is related to the ability of the ‘sickled’ cells to clump together and block blood vessels5,7.
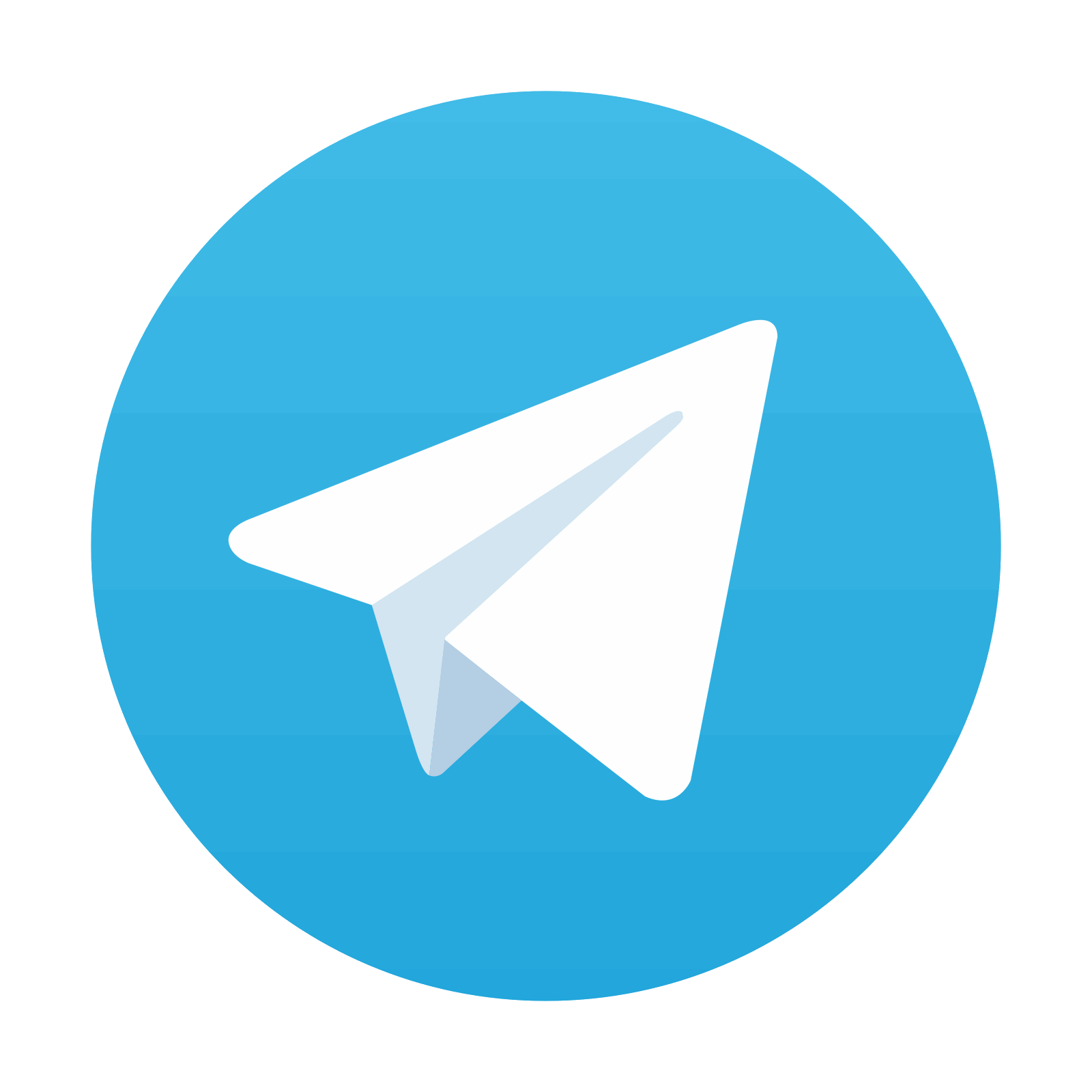
Stay updated, free articles. Join our Telegram channel

Full access? Get Clinical Tree
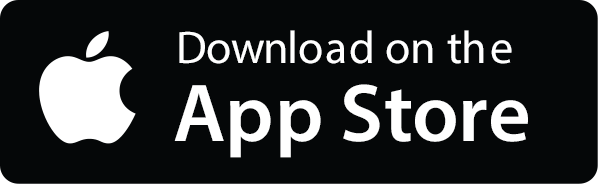
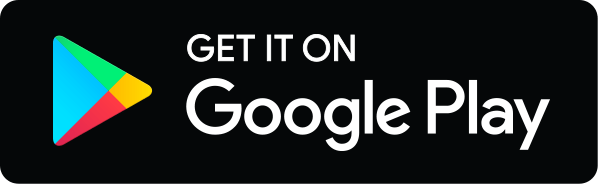