Gene Therapy and Pharmacogenomics
Objectives
When you reach the end of this chapter, you will be able to do the following:
1 Understand the basic terms related to genetics and drug therapy.
4 Discuss the different gene therapies currently available.
5 Differentiate between direct and indirect forms of gene therapy.
7 Briefly discuss pharmacogenomics and pharmacogenetics.
8 Discuss the evolving role of professional nurses as related to gene therapy.
Key Terms
Acquired disease Any disease triggered by external factors and not directly caused by a person’s genes (e.g., an infectious disease, noncongenital cardiovascular diseases). (p. 96)
Alleles The two or more alternative forms of a gene that can occupy a specific locus (location) on a chromosome (see chromosomes). (p. 95)
Chromatin A collective term for all of the chromosomal material within a given cell. (p. 96)
Chromosomes Structures in the nuclei of cells that contain threads of deoxyribonucleic acid (DNA), which transmit genetic information, and are associated with ribonucleic acid (RNA) molecules and synthesis of protein molecules. (p. 95)
Gene The biologic unit of heredity; a segment of a DNA molecule that contains all of the molecular information required for the synthesis of a biologic product such as an RNA molecule or an amino acid chain (protein molecule). (p. 95)
Gene therapy New therapeutic technologies that directly target human genes in the treatment or prevention of illness. (p. 97)
Genetic disease Any disorder caused directly by a genetic mechanism. (p. 96)
Genetic material DNA or RNA molecules or portions thereof. (p. 95)
Genetic polymorphisms (PMs) Variants that occur in the chromosomes of 1% or more of the general population (i.e., they occur too frequently to be caused by a random recurrent mutation). (p. 98)
Genetic predisposition The presence of certain factors in a person’s genetic makeup, or genome (see next page), that increase the individual’s likelihood of eventually developing one or more diseases. (p. 96)
Genetics The study of the structure, function, and inheritance of genes. (p. 96)
Genome The complete set of genetic material of any organism. It may be contained in multiple chromosomes (groups of DNA or RNA molecules) in higher organisms; in a single chromosome, as in bacteria; or in a single DNA or RNA molecule, as in viruses. (p. 96)
Genomics The study of the structure and function of the genome, including DNA sequencing, mapping, and expression, and the way genes and their products work in both health and disease. (p. 96)
Genotype The particular alleles present at a given site (locus) on the chromosomes of an organism that determine a specific genetic trait for that organism (compare phenotype). (p. 96)
Heredity The characteristics and qualities that are genetically passed from one generation to the next through reproduction. (p. 96)
Human Genome Project (HGP) A scientific project of the U.S. Department of Energy and National Institutes of Health to describe in detail the entire genome of a human being. (p. 97)
Inherited disease Genetic disease that results from defective alleles passed from parents to offspring. (p. 96)
Nucleic acids Molecules of DNA and RNA in the nucleus of every cell. DNA makes up the chromosomes and encodes the genes. (p. 95)
Personalized medicine The use of molecular and genetic characterizations of both the disease process and the patient for the customization of drug therapy. (p. 99)
Pharmacogenetics A general term for the study of the genetic basis for variations in the body’s response to drugs, with a focus on variations related to a single gene. (p. 98)
Pharmacogenomics A branch of pharmacogenetics (see earlier) that involves the survey of the entire genome to detect multigenic (multiple-gene) determinants of drug response. (p. 98)
Phenotype The expression in the body of a genetic trait that results from a person’s particular genotype (see earlier) for that trait. (p. 96)
Proteome The entire set of proteins produced from the information encoded in an organism’s genome. (p. 97)
Proteomics The detailed study of the proteome, including all biologic actions of proteins. (p. 97)
Recombinant DNA (rDNA) DNA molecules that have been artificially synthesized or modified in a laboratory setting. (p. 97)
http://evolve.elsevier.com/Lilley
• Answer Key—Textbook Case Studies
• Critical Thinking and Prioritization Questions
• Review Questions for the NCLEX® Examination
Genetic processes are a highly complex part of physiology and are far from completely understood. Genetic research is one of the most active branches of science today, involving many types of health care professionals, including nurses. Expected outcomes of this research include a deeper knowledge of the genetic influences on disease, along with the development of gene-based therapies. The practice of nursing requires an understanding of genetic concepts as well as genetically related health issues and therapeutic techniques. The goal of this chapter is to introduce some of the major concepts in this very complex and emerging branch of health science. In 1996, the National Coalition for Health Professional Education in Genetics (NCHPEG) was founded as a joint project of the American Medical Association, the American Nurses Association, and the National Human Genome Research Institute (http://www.nchpeg.org). The purpose of NCHPEG is to promote the education of health professionals and the public regarding advances in applied genetics.
Since the 1960s, published literature has described the role of nursing in genetics and genetic research. The Genetics Nursing Network was formed in 1984 and later became the International Society of Nurses in Genetics (ISONG). In 1997, the American Nurses Association designated genetics nursing as an official nursing specialty. In 2001, ISONG approved formation of the Genetic Nursing Credentialing Commission (GNCC). The growing understanding of genetics is quickly creating demand for clinicians in all fields who can educate patients and provide clinical care that tailors health care services to each patient’s inherent genetic makeup. This reality also calls for increasing the level of genetics education in nursing school curricula as well as continuing nursing education. Interestingly, the study of genetics has become commonplace in secondary and even primary education.
Basic Principles of Genetic Inheritance
Nucleic acids are biochemical compounds consisting of two types of molecules: deoxyribonucleic acid (DNA) and ribonucleic acid (RNA). DNA molecules make up the genetic material that is passed between all types of organisms during reproduction. In some viruses (e.g., human immunodeficiency virus), it is actually RNA molecules that pass the virus’s genetic material between generations; however, this is an exception to the norm. A chromosome is a long strand of DNA that is contained in the nuclei of cells. DNA molecules, in turn, act as the template for the formation of RNA molecules, from which proteins are made. Humans normally have 23 pairs of chromosomes in each of their somatic cells. Somatic cells are the cells in the body other than the sex cells (sperm cells or egg cells), which have 23 single (unpaired) chromosomes. One pair of chromosomes in each cell is termed the sex chromosomes, which can be designated as either X or Y. The sex chromosomes are normally XX for females and XY for males. One member of each pair of chromosomes in somatic cells comes from the father’s sperm and one from the mother’s egg. Alleles are the alternative forms of a gene that can vary with regard to a specific genetic trait. Genetic traits can be desirable (e.g., lack of allergies) or undesirable (e.g., predisposition toward a specific disease). Each person has two alleles for every gene-coded trait: one allele from the mother, the other from the father. An allele may be dominant or recessive for a given genetic trait. The particular combination of alleles, or genotype, for a given trait determines whether or not a person manifests that trait, or the person’s phenotype. Genetic traits that are passed on differently to male and female offspring are said to be sex-linked traits because they are carried on either the X or Y chromosome. For example, hemophilia genes are carried by females but manifest as a bleeding disorder only in males. Hemophilia is an example of an inherited disease; that is, a disease caused by passage of a genetic defect from parents to offspring. A more general term is genetic disease, which is any disease caused by a genetic mechanism. Note, however, that not all genetic diseases are inherited. Chromosomal abnormalities (aberrations) can also occur spontaneously during embryonic development. In contrast, an acquired disease is any disease that develops in response to external factors and is not directly related to a person’s genetic makeup. Genetics can play an indirect role in acquired disease, however. For example, atherosclerotic heart disease is often acquired in middle or later life. Many people have certain genes in their cells that increase the likelihood of this condition. This is known as a genetic predisposition. In some cases, a person may be able to offset his or her genetic predisposition by lifestyle choices, such as consuming a healthy diet and exercising to avoid developing heart disease.
Current literature differentiates “old genetics,” which focused on single-gene inherited diseases such as hemophilia, from the “new genetics.” The new genetic perspective recognizes that common diseases, including Alzheimer’s disease, cancer, and heart disease, are the product of complex relationships between genetic and environmental factors. These environmental factors, such as diet or toxic exposures, can initiate or worsen disease processes. Research into disease treatment is beginning to look at genetically tailored therapy.
Discovery, Structure, and Function of Dna
Genetics is the study of the structure, function, and inheritance of genes. Heredity refers to the qualities that are genetically transferred from one generation to the next during reproduction. A major turning point in the understanding of genetics came in 1953, when Drs. James Watson and Francis Crick first reported the chemical structures of human genetic material and named the primary biochemical compound deoxyribonucleic acid (DNA). They later received a Nobel Prize for their discovery.
It is now recognized that DNA is the primary molecule in the body that serves to transfer genes from parents to offspring. It exists in the nucleus of all body cells as strands in chromosomes, collectively called chromatin. As described in Chapter 40, DNA molecules contain four different organic bases, each of which has its own alphabetical designation: adenine (A), guanine (G), thymine (T), and cytosine (C). These bases are linked to a type of sugar molecule known as deoxyribose. In turn, these sugar molecules are linked to a “backbone” chain of phosphate molecules, which results in the classic double-helix structure of two side-by-side spiral macromolecular chains. An important related biomolecule is ribonucleic acid (RNA). RNA has a chemical structure similar to that of DNA, except that its sugar molecule is the compound ribose instead of deoxyribose and it contains the base uracil (U) in place of thymine. RNA more commonly occurs as a single-stranded molecule, although in some genetic processes it can also be double-stranded. In double-stranded structures, the base of each strand binds (via hydrogen bonds) to that of the other strand in the space between the two strands. This binding is based on complementary base pairings determined by the chemistry of the base molecules themselves. Specifically, adenine can only bind with thymine or uracil, whereas cytosine can only bind with guanine.
A nucleotide is the structural unit of DNA and consists of a single base and its attached sugar and phosphate molecules. A nucleoside is the base and attached sugar without the phosphate molecule. A relatively small sequence of nucleotides is called an oligonucleotide (the prefix oligo- means “a small number”). Certain new drug therapies involve synthetic analogues of both nucleosides and nucleotides (see Chapters 40, 45, 46, and 47). A related field is targeted drug therapy. Targeted drug therapy focuses on modifying the function of immune system cells (T cells and B cells) and biochemical mediators of immune response (cytokines). However, it is expected to focus on modifying specific genes as well. Current examples of targeted drug therapy are presented in Chapters 45, 46, 48, and 49. One of these drugs, the ophthalmic antiviral drug fomivirsen, is an oligonucleotide with a chemical structure that is opposite (complementary) to that of a critical part of the messenger RNA (mRNA) of the cytomegalovirus. For this reason, it is called an antisense oligonucleotide, and it is the first of this new class of drugs.
An organism’s entire DNA structure is its genome. This word is a combination of the terms gene and chromosome, and it refers to all the genes in an organism taken together. Genomics is the relatively new science of determining the location (mapping), structure (DNA base sequencing), identification (genotyping), and expression (phenotyping) of individual genes along the entire genome, and determining their function in both health and disease processes.
Protein Synthesis
Protein molecules drive the functioning of all biochemical reactions. Protein synthesis is the primary function of DNA in human cells. There is a direct relationship between DNA nucleotide sequence and corresponding amino acid sequences. This allows for precision in protein synthesis. Interestingly, it is estimated that only 2% to 3% of the human genome is involved in protein synthesis. Amino acid sequences control the shape of protein molecules, which ultimately affects their ability to function in the body. Mutations, undesired changes in DNA sequence, can affect the shape of protein molecules and impair or destroy their functioning.
In the cell nuclei, the double strands of DNA uncoil and separate, and a strand of mRNA forms on each separate DNA strand through complementary base pairing as described earlier in the chapter. This process is called transcription of the DNA. These mRNA molecules then detach from their corresponding DNA strands, leave the cell nucleus, and enter the cytoplasm, where they are then “read,” or translated, by the ribosomes. Ribosomes are composed of a second type of RNA known as ribosomal RNA (rRNA), as well as several accessory proteins. Individual sequences of three bases along the mRNA molecule serve to code for specific amino acid molecules. This translation process involves molecules of a third type of RNA, transfer RNA (tRNA). The tRNA molecules transport the corresponding amino acid molecules to the site of ribosomal translation along the mRNA strand in sequence according to the three-base codes along the mRNA strand. This in turn results in the creation of chains of multiple amino acids (polypeptide chains), which are known as protein molecules. The specificity of this genetic code is very important for proper protein synthesis, and the process is similar for all living organisms—plant and animal.
There are countless specific amino acid sequences (polypeptides) that result in the synthesis of many thousands of types of protein molecules. Proteins include hormones, enzymes, immunoglobulins, and numerous other biochemical molecules that regulate processes throughout the body. They are involved in both healthy physiologic processes and the pathophysiologic processes of many diseases. The biomedical literature continues to identify and describe many proteins that are part of disease processes. Manipulation of genetic material, as in gene therapy (see later in the chapter), can theoretically modify the synthesis of these proteins and therefore aid in the treatment of disease. This emerging science continues to give rise to novel terminology. The entire set of proteins produced by a genome is now known as the proteome. Proteomics is the study of the proteome, including protein expression, modification, localization, and function, as well as the protein-protein interactions that are part of biologic processes. This science is expected to provide new drug therapies in the future. Furthermore, most clinically approved drugs interact with body proteins such as cell membrane receptors, hormones, and enzymes.
Human Genome Project
In 1990, an unprecedented genetic research project began in the United States, the Human Genome Project (HGP). It was coordinated by the U.S. Department of Energy and the National Institutes of Health (NIH). The project was completed in 2003, two years ahead of schedule. The goals of this project were to identify the estimated 30,000 genes and 3 billion base pairs in the DNA of an entire human genome. Additional goals included developing new tools for genetic data analysis and storage, transferring newly developed technologies to the private sector, and addressing the inherent ethical, legal, and social issues involved in genetic research and clinical practice. However, the ultimate goal was to develop improved prevention, treatment, and cures for disease. When the HGP began, there were 100 known human disease-related genes. By its completion, there were 1400.
Gene Therapy
Background
Gene therapy is an experimental technique that uses genes to treat or prevent disease. It allows doctors to treat a disorder by inserting a gene into a patient’s cells instead of using drugs or surgery. Researchers are testing several approaches to gene therapy, including:
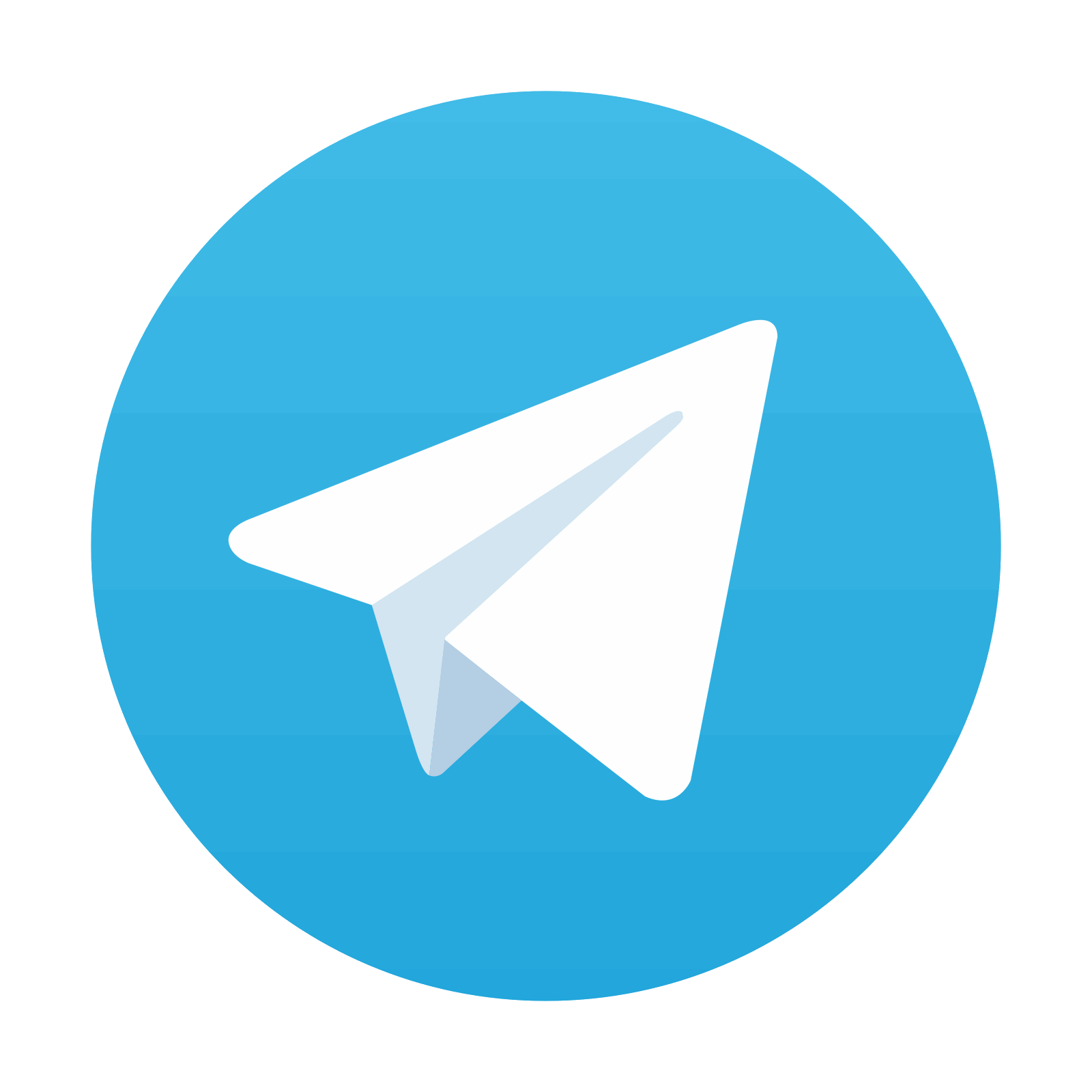
Stay updated, free articles. Join our Telegram channel

Full access? Get Clinical Tree
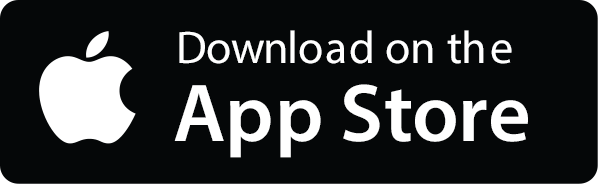
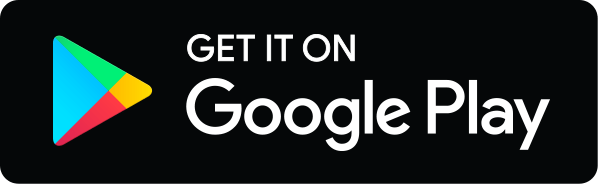