Chapter 9 Gastrointestinal Health Breakdown
When you have completed this chapter you will be able to
INTRODUCTION
The gastrointestinal (GI) tract is essentially a tube running through the body with its lumen open to the outside (see Figure 9.1). The GI tract is the only (normal) input channel to the body for water and nutrients. Four major functions are associated with the GI tract: ingestion, digestion, absorption and defaecation1. Ingestion refers to the taking in of food and water and is a voluntary action. Digestion is the breakdown of food into absorbable particles. There are two main ways in which this happens:
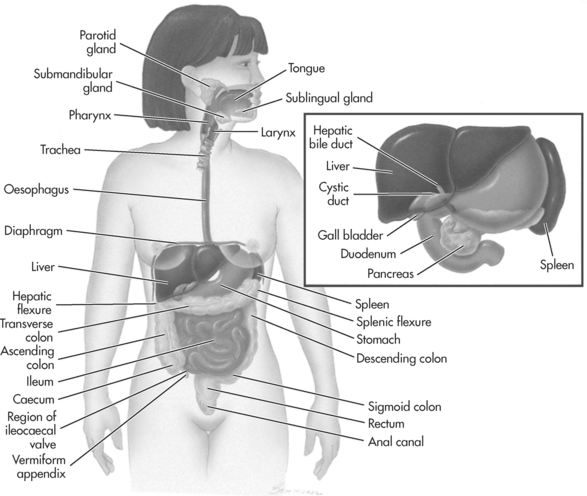
Figure 9.1 The gastrointestinal system
Source: Adapted from Thibodeau, GA & Patton, KT. 2003; Anatomy and Physiology (5th ed.), Mosby, St Louis, Fig. 25.1, p. 740.
Gut movements also help to mix food with the secreted solutions and so hasten digestion. Absorption is the process by which nutrients cross the gut lining and enter the body proper. Defaecation involves the excretion of unwanted or unusable ingested material together with shed epithelial cells from the gut lining plus some substances secreted into the gut, bile pigments for example, for excretion by this route2.
The GI tract or tube has the same basic structure throughout. It consists of layers of tissue, some with sub layers1. The innermost layer, forming a barrier between the outside and inside of the body is the mucosa, in most parts a single layer of epithelium rich in mucus-secreting goblet cells and in many parts also enzyme and hormone secreting cells. Immediately underlying the mucosa is a thin layer of smooth muscle, the muscularis interna, contraction and relaxation of which brings about movement of the mucosa. Beneath the mucosa is a layer of connective tissue containing blood vessels, lymphatics, and autonomic submucosal nerve plexuses. The muscularis externa, a layer of smooth muscle lies underneath this and forms the bulk of the GI tract wall. Generally it consists of two layers of muscle, an inner circular layer and an outer longitudinal layer. In some parts of the tract there is an additional oblique layer, and in some places there are thickenings of the circular layer forming sphincters, which prevent backflow and control the passage of food. There are autonomic myenteric nerve plexuses between the two muscle layers. The serosa forms a protective outer layer. In most parts of the GI tract within the abdomen, the outer layer is a double-layered serous membrane, the peritoneum1.
Put simply, the peritoneum may be regarded as a single continuous membrane. It lines the walls of the abdomino-pelvic cavity (as the parietal peritoneum) and also extends into the abdominal cavity and covers the external surfaces of most of the GI tract (as the visceral peritoneum). The peritoneal cavity is the area contained within the peritoneum and contains serous fluid, which acts as a lubricant. To provide a route for blood vessels, lymphatics and nerves supplying the GI, viscera two layers of peritoneum are fused, to form mesenteries. Most GI tract organs lie within the peritoneal cavity but some are retroperitoneal, for example the pancreas1.
Apart from movements involving the mouth, pharynx, and anus, GI tract movement is involuntary and mediated by smooth muscle1. Although not requiring a motor nerve stimulus for the initiation of contraction smooth muscle still contracts in a fairly ordered way and the gut has several pacemaker regions in the more proximal parts of the gut, which initiate waves of contraction (very similar to the pacemaker region in the heart)3. Smooth muscle contracts more slowly and sustains contraction longer than skeletal muscle. Its contraction is modified by autonomic nerves (hence the myenteric nerve plexuses) and by hormonal action. Parasympathetic (cholinergic) nerves increase contraction strength, duration and frequency, while sympathetic (adrenergic) nerves inhibit activity2.
Food is moved along the GI tract by peristalsis3, a wave of contraction passing along the gut from proximal to distal. This wave of contraction may involve the whole gut or just a segment, and contributes to mixing of the gut contents. Other types of contraction contribute to the breakdown of food into smaller particles as well as helping to mix the contents of the gut3. Segmentation is a movement in which muscle in different parts of the gut contract simultaneously, making the gut look like a string of sausages. On relaxation, this movement may be repeated by contraction of different areas of muscle.
Once food has been ingested the digestive process begins. Mechanical digestion begins in the mouth with chewing and movement of the food with the tongue1. This starts the breakdown process and mixes food with saliva, which begins the process of chemical digestion. Salivary secretion is a parasympathetic reflex and involves cerebral as well as local stimuli. The sight, smell, taste or even thought of food will cause secretion of saliva as will the presence of food in the mouth3. Saliva contains water (97–99%), ions, mucus, an enzyme (salivary amylase), antibodies, and the antibacterial compound lysozyme. Salivary amylase begins the breakdown of starches (polysaccharides) to smaller molecules (oligosaccharides).
Swallowing (deglutition) is a complex process involving the tongue, soft palate, pharynx and oesophagus2. Food is first compacted into a bolus by the tongue. It is then ‘tipped’ back into the oropharynx by the tongue. The tip of the tongue is raised against the hard palate. This is the voluntary or buccal phase of swallowing. After this point food is out of our voluntary control.
The pharyngeal-oesophageal phase of swallowing is controlled by the swallowing centre in the medulla oblongata2. The bolus is gripped by the muscles of the wall and ‘squeezed’ downwards. All routes except the correct one, the oesophagus, being blocked off. The tongue blocks off the mouth; the soft palate rises to block off the nasopharynx; and the larynx rises so that the epiglottis blocks off the respiratory passages. The bolus of food then passes down the oesophagus into the stomach by peristaltic action.
Once in the stomach the bolus comes into contact with gastric juice, secreted by various cells in the gastric glands1. Mucous neck cells (found at the necks of gastric pits) secrete special mucus, which protects the stomach lining from the effects of its acid secretions. Parietal (oxyntic) cells found among the mucous cells of the neck secrete hydrochloric acid, which keeps the pH of the stomach at approximately 2.0. Zymogenic (chief) cells located at the base of the glands secrete pepsinogen (an inactive form of a proteolytic enzyme). When food enters the stomach, at first the pH rises because the protein content buffers H+ but as the pH falls salivary amylase ceases to act. The acid pH converts pepsinogen to pepsin – an enzyme which breaks proteins into large polypeptides. The acid continues the further breakdown of the food and effectively kills most microorganisms present.
Gastric juice is secreted in three phases3. The sight, smell, taste or thought of food cause gastric secretions to occur. This is mediated via a branch of the vagus nerve and begins before food enters the stomach. Distension of the stomach by food initiates local gut reflexes and ‘long loop’ reflexes, involving the central nervous system, which result in the secretion of more gastric juice. The presence in the stomach of partially digested protein, a high pH, or caffeine, stimulate the secretion of gastrin, a hormone secreted by the enteroendocrine cells of the gastric mucosa. Gastrin is secreted into the systemic circulation. However, it only affects the secretory cells of the gastric glands causing them to release both HCl and pepsinogen. HCl secretion is also increased by histamine, released by the enteroendocrine cells of the gastric mucosa in response to the presence of food in the stomach.
When food first enters the duodenum there is a release of intestinal gastrin, from enteroendocrine cells in the duodenum1. Gastrin circulates systemically and brings about a further increase in gastric secretion. Later as larger amounts of H+ and fats enter the duodenum there is inhibition of gastric secretion via both nervous and hormonal factors (secretin, cholecystokinin [CCK] and gastric inhibitory peptide [GIP]). Gastric secretion is also inhibited by a fall in pH in the stomach.
The stomach continually compresses, kneads and twists to mix the contents, producing chyme1. Muscular activity is also enhanced by the same hormonal and nervous reflexes that enhance gastric secretion (and is similarly inhibited by the same inhibiting factors). There are receptors for the hormones on the gastric smooth muscle.
Peristaltic activity in the pylorus causes release of small amounts (3–5 mL) of chyme into the duodenum up to 2 to 3 times each minute2. Gastric emptying is inhibited by chyme rich in fats. Liquids pass through the stomach fairly rapidly – generally the stomach empties within 4 hours of a meal – but a fatty meal may delay this by a further 2 hours or so3.
Only a few fat-soluble substances are absorbed in the stomach (e.g., aspirin, alcohol, some drugs)1. The oxyntic cells also secrete intrinsic factor – a glycoprotein essential for the absorption of vitamin B12. These two substances combine in the stomach and are absorbed in the small intestine2. The glands in the duodenum secrete an alkaline-rich juice, which neutralises the acid chyme coming from the stomach1. In the duodenum chyme also mixes with pancreatic juice and bile.
The exocrine cells of the pancreas secrete from 1200 to 1500mL/day1. Pancreatic juice contains water, enzymes and ions, especially HCO3− which further contributes to the neutralisation of the acid chyme. The pancreatic enzymes are concerned with the digestion of all food types. A major component of pancreatic juice is a series of proteases or protein splitting enzymes – trypsinogen, chymotrypsinogen and procarboxypeptidase. These are all initially inactive. Trypsinogen is activated by enterokinase, normally bound to the surface of the duodenal lining cells, to form trypsin. Once present trypsin in turn activates chymotrypsinogen to form chymotrypsin, then chymotrypsin in turn activates procarboxypeptidase to form carboxypeptidase. The pancreas also secretes amylases, to digest carbohydrates; lipases, to digest fats; and nucleases, to digest nucleic acids.
Stimulation of the vagus nerve, brought about by eating and by the presence of food in the stomach, also causes some pancreatic secretion3. The presence of HCl in the duodenum brings about secretion of two hormones: secretin, which causes the secretion of pancreatic HCO3−; and cholecystokinin (CCK), which causes secretion of pancreatic digestive enzymes.
Bile is secreted by the liver and stored and concentrated in the gall bladder1. It is released in small quantities in response to vagal stimulation and in large quantities in response to CCK. Five hundred to 1000 mL of bile is secreted each day1. Bile is a yellow-green, alkaline fluid containing bile salts, which are cholesterol derivatives and the bile pigments, bilirubin and biliverdin. The latter are waste products from the breakdown of haemoglobin. Bile salts emulsify fats and so make their digestion easier. They are also important in fat absorption. Bile salts are not excreted but are reabsorbed in the small intestine and recycled. Bile pigments colour and deodorise faeces and so are excreted.
Chyme is moved further on into first the jejunum and then the ileum1. The intestinal glands here secrete 1 to 2 L of juice per day3. The intestinal juice is mainly water but also contains a great deal of mucus as well as digestive enzymes. Intestinal digestive enzymes are bound to the microvilli on the surfaces of the intestinal epithelial cells, and, for that reason are often called ‘brush border’ enzymes. These are found mainly in the duodenum and are most active here.
Digestive processes are essentially completed in the small intestine3. Proteins are finally broken down to amino acids (or often pairs of amino acids). Carbohydrates are broken down to monosaccharides, while fats are broken down to fatty acids and glycerol.
Daily we ingest up to 10 L of food and drink, but only about 500mL to 1 L reaches the large intestine1. The rest is absorbed in the small intestine. Absorption occurs all along the small intestine but is mostly completed by the time chyme reaches the ileum. At the end of the ileum all that remains is water, indigestible food material (e.g., cellulose) and millions of bacteria. This all passes into the large intestine or colon.
Most nutrients are absorbed through the mucosa of the villi3. Water-soluble products, such as amino acids and monosaccharides are transported into and across the lining cells of the intestine and then enter capillary blood in the villus. Lipids, however, diffuse passively across the mucosa and are taken up by the lacteal (lymph vessel) and carried to the blood via the lymph system. The veins draining the stomach and small intestine empty into the hepatic portal vein and the blood passes through the liver before returning to the systemic circulation1. The liver is able to extract and process absorbed nutrients from the blood as required.
Chyme entering the colon contains few nutrients3. The colon absorbs water, ions and some vitamins. The terminal ileum and colon contain large quantities of bacteria. Some bacteria enter the GI tract via the anus, while others survive passage through the stomach and small intestine. The more neutral pH of the ileum and colon may encourage their growth. These bacteria present in the colon play important roles in body metabolism. They digest some of the remaining carbohydrates in chyme, in the process producing some irritating acids and gases. The human body produces about 500 mL of gas each day. These gases are eventually passed as flatus. Bacteria in the colon also synthesise some B-complex vitamins and most of the vitamin K required by the body3.
Two main types of muscular activity are observed in the colon3. Haustral contractions are slow segmenting movements. Distension of a haustrum causes a contraction, which moves the contents along the colon into the next haustrum. This type of movement also mixes the contents and aids in water absorption. Mass movements are long, slow moving, contractile waves, which move over the entire length of the colon 3 or 4 times a day1. These mass movements usually occur during or just after eating (the gastrocolic reflex).
Material that reaches the anus is semi-solid1. Most of the water still present when chyme enters the caecum, has been absorbed by the colon and the material present is now called faeces. Faeces are forced into the rectum by mass movements. The rectum is usually empty and when faeces enter, the rectal wall becomes stretched, which initiates the defaecation reflex.
This is a spinal cord mediated parasympathetic reflex3. It involves contraction of the walls of the sigmoid colon and rectum and relaxation of the internal anal sphincter (smooth muscle). The external sphincter can be controlled since it is composed of skeletal muscle and so defaecation can be delayed for a time. Expulsion of faeces is aided by contraction of the levator ani muscle and raising the intra-abdominal pressure. The latter is achieved by the valsalva manoeuvre, essentially exhaling against a closed glottis2.
CHOLECYSTITIS
WHAT IS THE PATHOPHYSIOLOGY?
Cholecystitis is inflammation of the gallbladder, most commonly caused by a build up of bile in the gallbladder when the cystic duct is obstructed by gallstones1. The common (though not exclusive) risk factors for the disease are known as the four Fs: female, forties, fat, and fertile2.
The gallbladder holds bile that has been produced by the liver, before it is used by the gut. Bile is made from cholesterol and becomes more concentrated in the gallbladder. Sometimes the cholesterol precipitates, forming crystals and gallstones. This can occur if the bile becomes too concentrated, or if there is an oversupply of cholesterol3. Stone formation is a slow process. It is approximately eight years from the beginning of stone development to the onset of symptoms, and another four years to cholecystectomy4.
WHAT ARE THE CLINICAL MANIFESTATIONS?
Commonly intense sudden pain in the right upper quadrant is experienced. The pain is recurrent in nature, occurring after fatty meals and classically, the pain increases on deep inspiration2. The abdominal pain typically radiates to the right shoulder and scapula. Murphy’s sign is also frequently seen in cholecystitis. It is characterised by the patient holding their breath, in response to pain, when their abdomen is palpated in the right subcostal region5.
DIAGNOSTIC TESTS
In the patient’s blood results, a raised bilirubin level and raised liver function tests (LFT) will be present. The white blood cell count may be increased2. Ultrasound, computerised tomography (CT) and cholangiography (dye and X-rays) may be used to diagnose the disease. An ultrasound of the abdomen is the least invasive of these procedures and is able to be done at the bedside6.
WHAT IS THE TREATMENT?
Initially, intravenous fluids to correct or prevent dehydration are commenced, and analgesia is given, usually morphine2. The patient is made nil by mouth. Surgical intervention is the normal treatment involving the removal of the gall bladder. This is called a cholecystectomy and is done either laparoscopically or through a laparotomy. Laparoscopic techniques are safer, cause the patient less pain and are the preferred method of cholecystectomy4. Traditionally surgery was delayed for several weeks, however early surgery is now advocated by most clinicians7.
APPLIED PHARMACOLOGY
Antibiotics are given if peritonitis or sepsis is suspected. This may occur if infective organisms or bile leaks from gallbladder rupture into the sterile peritoneal cavity. But in an uncomplicated case of acute cholecystitis antibiotics are usually not given2.
NURSING IMPLICATIONS
The main nursing considerations are analgesia and hydration. The analgesics used are typically opioids, such as morphine, given via the intravenous or intramuscular route. The intravenous route is favoured as smaller doses can be given at frequent intervals. Giving smaller doses of morphine at close intervals maintains a steadier therapeutic blood level, and thus better pain relief. ‘An order for intramuscular morphine every four hours is unlikely to provide satisfactory analgesia for more than 20% of that interval’8. Antiemetic cover should also be given.
CIRRHOSIS
WHAT IS THE PATHOPHYSIOLOGY?
The changing of the microscopic architecture of the liver in cirrhosis causes many complications. All functions of the liver, including filtering of blood draining from the small intestine, and the production of albumin and blood clotting products are decreased9. The small nodules and recurring strips of connective tissue toughen the normally spongy liver. If the liver is too solid for blood to flow through it, back pressure will occur. This will cause distension of the veins that flow to the liver from the gut10. This distension is called portal hypertension and leads to the creation of varices and collateral blood flow. Oesophageal varices are prone to massive bleeding,11 which often presents as haematemesis.
WHAT ARE THE CLINICAL MANIFESTATIONS?
The disease has a long latent period as the liver becomes slowly less efficient. Ascites (accumulation of protein-rich fluid in the peritoneum due to obstruction of the portal circulation) and jaundice (yellow-green discolouration of the skin and sclerae due to rising bilirubin levels, reflecting deteriorating liver function) are common manifestations. Dramatic haematemesis can also occur. Generalised weakness and weight loss are frequently observed. Eventually complete liver failure can ensue, leading to coma and death12. This is believed to be caused by high levels of ammonia in the blood. As the liver normally breaks down ammonia, the high blood levels seen with this disease precipitate hepatic encephalopathy.
DIAGNOSTIC TESTS
Initially liver function tests may be normal. Decreased albumin and increased prothrombin time indicate worsening liver function. Prothrombin is a blood-clotting factor produced by the liver. Anaemia is common as alcohol directly suppresses red blood cell production in the bone marrow13.
WHAT IS THE TREATMENT?
The only definitive treatment for cirrhosis is liver transplantation9. The majority of interventions in cirrhosis management are supportive, as donor livers for transplantation are scarce. Good nutrition and abstinence from alcohol are imperative. Ascites can usually be controlled with diuretics and a diet that is salt restricted9. Oesophageal varices, visualised with an endoscope, may be injected with adrenaline, or banded with small rubber bands, in order to achieve haemostasis. This technique in combination with intravenous pharmacotherapy is most effective. Intravenous shunts are infrequently used as they carry a mortality rate between 40 and 100%10. A shunt is inserted from the liver to the inferior vena cava, to reduce the pressure from the distended veins. Occasionally a balloon tamponade device (a special nasogastric tube which has balloon inflation components) is inserted. The balloons put direct pressure on the bleeding varices. This type of nasogastric tube is called by one of three names: a Sengstaken Blakemore tube, Linton tube, or a Minnesota tube. This is typically a bridging procedure to more definitive medical care14.
APPLIED PHARMACOLOGY
Pharmacotherapy is a first-line approach to controlling variceal bleeding. Vasopressin, a potent vasoconstrictor, may be used to try to control bleeding. It reduces blood flow to the abdominal organs11. Its use is controversial and not recommended in the elderly as it can cause cardiac and bowel ischaemia9. Terlipressin is a synthetic form of vasopressin, with much longer half-life but less potent vasoconstriction effects than vasopressin. It is an effective treatment for variceal bleeding, and it has a lower mortality rate15.
Somatostatin and octreotide are two intravenously administered peptides that can also reduce bleeding from oesophageal varices. Although these two drugs reduce blood loss, they have not been found to reduce overall mortality16. The mechanism of action is not known, however they may reduce portal pressure by having an effect on vasoactive peptides, such as glucagon11. There is some evidence that milk thistle (Silybum marianum) may exert a protective effect on the cirrhotic liver; however the mechanism of action is not understood17.
NURSING IMPLICATIONS
Sudden abstinence from alcohol can cause severe withdraw symptoms (delirium tremens). This can make nursing care of the patient with cirrhosis challenging. Symptoms include tremor, hallucinations and agitation. Gross ascites can cause respiratory distress by increasing upward pressure on the diaphragm which compromises lung expansion. The patient may require oxygenation and ventilatory support. Haematemesis can be remarkable and difficult to control. It is common for the patient to be haemodynamically unstable11. Timely resuscitation and administration of blood products is important.