Chapter 15 Drug treatment of infections
After reading this chapter, you should be able to:
INTRODUCTION
The resistance of bacteria to antibiotics (p. 293) is increasingly becoming a very significant problem. The cost of resistance is measured not only in terms of failure of therapy but also in the increased costs of more expensive drugs needed to combat the resistant bacteria. In 1994, the World Health Organization Scientific Working Group on the Monitoring and Management of Bacterial Resistance to Antimicrobial Agents (Tenover and Hughes 1995) discouraged the unnecessary use of antibiotic prophylaxis in food animals and stated that ‘antimicrobial agents should not be used as a substitute for adequate hygiene in animal husbandry’.
Many conditions for which antibiotics are prescribed are either of a self-limiting nature or are viral in origin and, as such, do not require antibiotics. The causes of this inappropriate prescribing by clinicians include insufficient training or knowledge, difficulty in selection of the appropriate drug, lack of microbiological information, fear of litigation and patients’ expectations (Binyon and Cooke 2000).
HEALTHCARE-ASSOCIATED INFECTIONS
Approximately 9% of patients in UK hospitals suffer from an infection acquired during their hospital stay (Crowcroft and Catchpole 2002), many of which are due to multiresistant, Gram-positive and Gram-negative pathogens. The incidence of colonisation and infection with these pathogens continues to rise due to failures in hospital hygiene and selective pressures created by overuse of antibiotics.
Infection with these resistant pathogens can adversely affect clinical, microbiological and economic outcomes (Cosgrove and Carmeli 2003), and the costs associated with managing infections are considerable. In the UK, it has been estimated that costs increase threefold when hospital patients present with one or more healthcare-associated infections during an inpatient stay (Plowman 2000).
CLASSIFICATION OF BACTERIA
Bacteria can be broadly classified according to cell shape (Fig. 15.1), that is:
can be used in the classification of infectious bacteria (Table 15.1).
MICROBIOLOGY
Table 15.2 Causative pathogens in some common bacterial infections
Infection(s) | Bacterium or bacteria responsible |
---|---|
Respiratory infections | |
Exacerbation of chronic bronchitis | Haemophilus influenzae |
Streptococcus pneumoniae | |
Pneumonia | Streptococcus pneumoniae |
Staphylococcus aureus | |
Haemophilus influenzae | |
Urinary tract infections | Escherichia coli |
Proteus spp. | |
Klebsiella spp. | |
Streptococcus faecalis | |
Pseudomonas | |
Venereal disease | |
Gonorrhoea | Neisseria gonorrhoeae |
Non-specific urethritis | Chlamydia |
Skin/soft tissue infections | |
Intravenous catheter site | Staphylococcus aureus |
Staphylococcus epidermidis | |
Surgical wound | Staphylococcus aureus |
Gram-negative rods | |
Furuncle | Staphylococcus aureus |
Endocarditis | |
Acute | Staphylococcus aureus |
Streptococcus pyogenes | |
Gram-negative bacilli | |
Subacute | Streptococcus spp. |
Staphylococcus epidermidis | |
Gram-negative bacilli | |
Septicaemia | Staphylococcus aureus |
Streptococcus pneumoniae | |
Coliforms | |
Enterobacter spp. | |
Meningitis (in adults; many organisms may cause meningitis in neonates) | Streptococcus pneumoniae |
Neisseria meningitides | |
Food poisoning | Salmonellae |
Clostridium perfringens |
Other factors affecting the choice of antibiotic include:
ANTIBACTERIAL DRUGS
Antibacterial drugs act by a number of mechanisms (Fig. 15.2). They can be either bactericidal (kill bacteria) or bacteriostatic (arrest the growth of bacteria) (Box 15.1). Bacteriostatic agents, because they do not kill bacteria, rely on the host’s immune and cell defence mechanisms to clear the bacteria. If these defence mechanisms are compromised, a bactericidal drug may be preferable.
MINIMUM INHIBITORY CONCENTRATION OF ANTIBIOTICS
As a guide to the sensitivity of a specific micro-organism to an antibiotic, the minimum inhibitory concentration (MIC) is utilised. This is the lowest concentration of antibiotic that will inhibit the growth of a given strain of micro-organism under controlled conditions. The lower the concentration, the more potent the antibiotic. However, the MIC is determined in laboratory conditions. In vivo, the drug may have to pass from the plasma into infected tissue to destroy bacteria. The penetration of antibiotics into abscess cavities may be poor, and surgical drainage is often necessary. In either case, higher doses would be required to achieve the MIC.
ROUTINE PROPHYLAXIS
Routine antibiotic prophylaxis in surgical patients is needed only for those procedures associated with a high risk of postoperative infection (e.g. vaginal or colorectal surgery) or prosthetic insertions. Normally, prophylactic antibiotic treatment should not be extended beyond 48 h following surgery and should be given parenterally. Intravenous administration during induction, or intramuscular administration 30 min before surgery, usually ensures effective blood levels of antibiotic at the time when anticipated bacteraemia is likely to be highest. When gastrointestinal function is unimpaired during the postoperative period, oral administration of antibiotics provides an optimum non-invasive approach.
MONITORING THE PATIENT’S RESPONSE
The patient’s response to antibiotic therapy is monitored with regard to:
SUPERINFECTION
Superinfection may occur as a result of the following.
BACTERIAL RESISTANCE TO ANTIBIOTICS
The resistance of bacteria to antibiotics is a problem that has continued to grow in parallel with the development of new antibiotics. Bacterial resistance reflects antibiotic use and is more of a problem when controls on antibiotic use are lax. The sensible use of antibiotics reduces this. There are several mechanisms by which resistance may emerge.
TRANSFERRED RESISTANCE
There are several mechanisms of antibiotic resistance.
METHICILLIN-RESISTANT STAPHYLOCOCCUS AUREUS
In order to control and minimise the spread of MRSA, there must be compliance with the following:
TREATMENT OF MRSA INFECTION
Patients who demonstrate clinical signs of infection will require treatment with the appropriate antibiotics. The agent used will depend on the site of infection (Table 15.3). Some of these organisms are sensitive only to vancomycin or teicoplanin. Strains may be susceptible to rifampicin, sodium fusidate, tetra-cyclines, aminoglycosides and macrolides. Treatment is guided by the sensitivity of the infecting strain. Swabs are taken from the nose and throat to establish a diagnosis (Figs 15.3 and 15.4).
Site | Treatment |
---|---|
Nasal carriage only | Nasal decolonisation only |
Throat carriage | Nasal and throat decolonisation |
Axilla or groin carriage | Nasal and body decolonisation |
Linezolid and quinupristin with dalfopristin
Linezolid and the combination of quinupristin and dalfopristin are active against MRSA, but these antibacterial drugs should be reserved for organisms resistant to other antibacterials or for patients who cannot tolerate other antibacterial drugs. Linezolid is available both orally (600 mg every 12 h) and by intravenous infusion. Thrombocytopenia, anaemia, leucopenia and pancytopenia have been reported, and weekly monitoring of full blood counts is recommended. Quinupristin with dalfopristin is available as an intravenous infusion. A novel broad-spectrum glycycline antibiotic (tigecycline) is active against Gram-positive, Gram-negative and anaerobic organisms including multidrug-resistant strains (see p. 301).
THE PENICILLINS
The most significant and adverse effect of the penicillins is hypersensitivity, which manifests as rashes and, on occasion, anaphylaxis. Allergy to one penicillin indicates allergy to them all, because the hypersensitivity is related to the basic penicillin structure.
Diarrhoea often occurs during oral penicillin therapy.
BENZYLPENICILLIN AND PHENOXYMETHYLPENICILLIN
Benzylpenicillin is effective in a wide range of infections, including those shown in Table 15.4.
Table 15.4 Infections for which benzylpenicillin is effective
Organism | Disease |
---|---|
Beta-haemolytic streptococci | Septicaemia, tonsillitis |
Viridans streptococci | Subacute bacterial endocarditis |
Streptococcus pneumoniae | Pneumonia |
Neisseria meningitidis | Meningococcal meningitis |
Clostridium tetani | Tetanus |
Clostridium perfringens | Gas gangrene |
Treponema pallidum | Syphilis |
Neisseria gonorrhoeae | Gonorrhoea |
Borrelia burgdorferi | Lyme disease |
The dose range and side effects of benzylpenicillin and phenoxymethylpenicillin are shown in Table 15.5.
PENICILLINASE-RESISTANT PENICILLINS
Some organisms are resistant to penicillin because of their ability to produce the enzyme penicillinase, which destroys penicillin. Flucloxacillin is available for treatment of staphylococcal infections when resistant organisms are particularly common (see Table 15.6). Flucloxacillin is available in oral as well as injectable form and is well absorbed.
Table 15.6 Penicillinase-resistant penicillin
Drug | Adult dose range | Notes |
---|---|---|
Flucloxacillin | Oral: 250 mg 6-hourly at least 30 min before food. | Side-effects as for benzylpenicillin; doses can be doubled in severe infections |
IM: 250 mg 6-hourly. | ||
Slow IV injection or infusion: 0.25–1 g every 6 h. |
BROAD-SPECTRUM PENICILLINS
Co-amoxiclav is a combination of amoxicillin and beta-lactamase inhibitor, clavulanic acid. The clavulanate molecules penetrate the bacterial cell and combine with the beta-lactamase molecules. This inactivates the beta-lactamases, leaving the amoxicillin free to exert a full bactericidal effect. This makes the combination active against beta-lactamase-producing bacteria that are resistant to amoxicillin: Staphylococcus aureus, Escherichia coli and Haemophilus influenzae, as well as many Bacteroides and Klebsiella species. The broad-spectrum penicillins are summarised in Table 15.7.
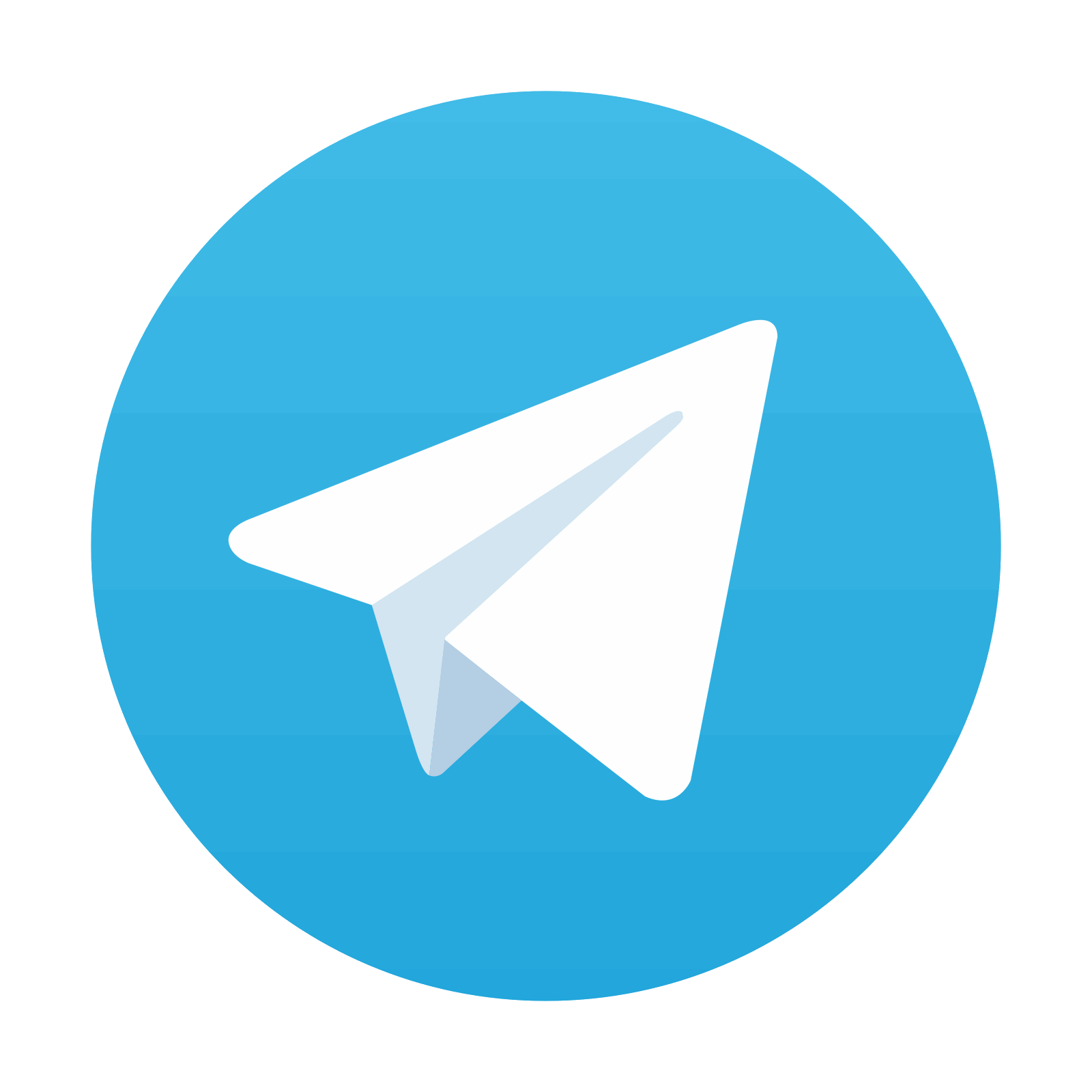
Stay updated, free articles. Join our Telegram channel

Full access? Get Clinical Tree
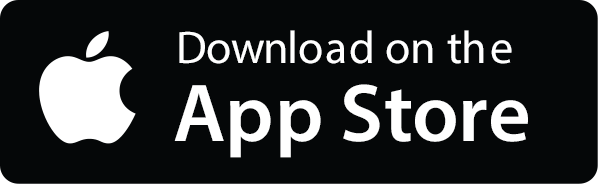
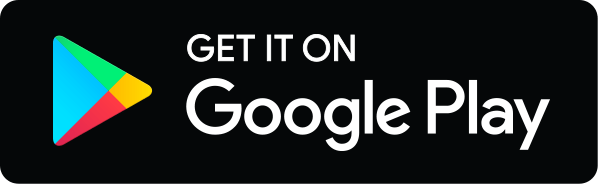