Chapter 12 Drug treatment of cardiovascular disorders
INTRODUCTION
Diseases of the heart and circulatory system are the main cause of death in the UK and accounted for just over 216 000 deaths in 2004 (British Heart Foundation 2006). More than one in three people (37%) die from cardiovascular disease (CVD). The main forms of CVD are coronary heart disease (CHD) and stroke. About half (49%) of all deaths from CVD are from CHD, and more than a quarter (28%) are from stroke. Around one in five men and one in six women die from CHD. Death rates from CVD have been falling in the UK since the early 1970s. For people under 75 years, they have fallen by 38% in the past 10 years. Just under one in three of all deaths under 65 years resulting from social class inequalities is due to CHD. Death rates from CHD are highest in Scotland and the North of England, lowest in the South of England, and intermediate in Wales and Northern Ireland. The premature death rate for men living in Scotland is 57% higher than in the South West of England, and 103% higher for women.
The incidence of myocardial infarction (MI) varies around the UK, but on average the incidence rate for men aged between 30 and 69 is about 600 per 100 000 and for women is about 200 per 100 000. Using data from Morbidity Statistics from General Practice, the British Heart Foundation estimates that there are about 183 000 new cases of angina in all men living in the UK and about 161 000 in women. Studies of the incidence of heart failure are scarce, and different studies use different methods, particularly for diagnosing the condition. The Hillingdon Heart Failure Study found a crude incidence rate of 140 per 100 000 for men and 120 per 100 000 for women. Data from the 2003 Health Survey for England suggest that the prevalence of CHD in England is 7.4% in men and 4.5% in women. Prevalence rates increase with age, with around one in four men and one in five women aged 75 years and above living with CHD. It is estimated that there are just over 1.5 million men living in the UK who have had CVD (either angina or heart attack) and about 1.1 million women, giving a total of around 2.6 million.
ANATOMY AND PHYSIOLOGY
THE HEART
The heart lies between the lungs, behind the lower sternum, in front of the oesophagus and above the diaphragm, on which it rests. It is roughly conical in shape, with a base and an apex. It consists of four chambers: the right and left atria above, and the right and left ventricles below. The atria and ventricles are separated by, on the right side, the tricuspid valve and, on the left side, the mitral valve. The walls of the heart have three layers: outermost, a fibrous envelope called the pericardium; in the middle, a thick muscle known as the myocardium; and the innermost layer, a smooth lining called the endocardium (Fig. 12.1).

(From Waugh A, Grant A 2001 Ross and Wilson anatomy and physiology in health and illness, 9th edn. Churchill Livingstone, Edinburgh. With permission of Elsevier.)
Venous blood returns from various parts of the body to the heart. It enters the right atrium via the superior and inferior venae cavae and passes through the tricuspid valve to the right ventricle. The right ventricle pumps the blood to the lungs via the pulmonary artery. In the lungs, the blood is oxygenated and carbon dioxide is removed. The blood then returns via the four pulmonary veins into the left atrium, from where it passes through the mitral valve into the left ventricle. The left ventricle pumps the oxygenated blood through the aortic valve into the aorta and out into the body.
The heart derives its own blood supply from the two main coronary arteries that originate from the aorta just above the aortic valve.
The activity of the heart is rhythmical, consisting of contraction (systole) and relaxation (diastole). The impulse to contract is generated by a microscopic area of specialised cardiac muscle known as the sinoatrial (SA) node, situated at the junction of the superior vena cava and the right atrium. The wave of excitation spreads throughout the muscle layer of both atria, causing them to contract, forcing blood into the ventricles. The impulse is picked up by another small mass of specialised cardiac muscle called the atrioventricular (AV) node, situated in the septal walls of the right atrium. It is relayed by the fibres of Purkinje down the bundle of His and along the right and left branches, causing the ventricles to contract and drive blood into the pulmonary artery and the aorta. The heart then relaxes, refills with venous blood, and awaits the next stimulus for contraction. Although the heart initiates its own impulse to contract, the fine adjustments to its activity required to meet the body’s constantly changing needs derive from the autonomic nervous system (see Ch. 9). Sympathetic innervation increases the heart rate, and parasympathetic innervation slows the heart rate.
Every time the heart beats, approximately 70 mL of blood is pumped out of each ventricle. The heart rate is normally around 70 beats/min. These two figures multiplied together are termed the cardiac output.
THE BLOOD VESSELS
The blood is transported round the body via the blood vessels, which comprise arteries and arterioles, veins and venules, and capillaries. As a rule, arteries convey oxygenated blood and veins convey deoxygenated blood, which has a high percentage of carbon dioxide. Arteries convey blood away from the heart; veins transport blood back to the heart. Capillaries are tiny blood vessels in the periphery of the arteriovenous system. It is through the capillary walls that oxygen and nutrients pass to the tissues and cells and that waste products return from the cells into the circulation.
Like the heart, the walls of the blood vessels consist of three layers: the fibrous outer tunica adventitia; the middle muscular layer, the tunica media; and the smooth lining, the tunica intima. The outer coat of an artery allows it to stand open, whereas a vein collapses when it is cut. The proportion of muscle tissue depends on the size of the vessel, with much more in arteries than in veins. Some veins have valves that allow the blood to flow back to the heart but prevent flow in the opposite direction. The walls of the capillaries are only one cell thick; this readily facilitates gaseous and nutrient exchange (Fig. 12.2).
POSITIVE INOTROPIC DRUGS
DIGOXIN
Digoxin is used in the management of atrial flutter and fibrillation, as it reduces conduction through the AV node and the bundle of His, allowing fewer of the excitatory transmissions from the atria to pass and hence slowing ventricular rate and restoring rhythm. In chronic congestive cardiac failure, digoxin is given to increase the force of myocardial contraction, hence increasing cardiac output for any given filling pressure.
Mode of action.
The actions of digoxin are complex and revolve around its ability to inhibit sodium transport out of cells through inhibition of the enzyme Na+, K+-ATPase, which allows increased intracellular myocardial calcium concentrations. This, in turn, improves contractility.
Pharmacokinetics.
Digoxin may be given orally as either tablets or a liquid preparation. It may also be given intravenously, but this is relatively rare. Intravenous digoxin should always be given very slowly to minimise the risks of exposing the myocardium to localised high concentrations that may induce arrhythmias. Digoxin should be added to an intravenous infusion of sodium chloride 0.9% and administered using an intravenous infusion pump over 2 h. Because digoxin is 80% renally eliminated, the dose must be adjusted according to renal function in order to minimise the risks of toxicity. In the elderly, the renal clearance is lower than that in young healthy adults, so a lower maintenance dose is required. The elimination half-life of digoxin in a patient with normal renal function is 36 h, which means that up to 7 days may be required after any dosage alteration to ensure that a steady state has been reached.
Dose.
Digoxin is now rarely used for the rapid control of heart rate, as even with intravenous administration it may take many hours to reduce the heart rate. In patients who have mild heart failure, a loading dose is not required and a satisfactory plasma concentration can be achieved over a period of about a week using a dose of 125–250 micrograms orally twice a day, which may then be reduced having special regard to renal function. Because of its long half-life, maintenance doses need be given only once daily. The maintenance dose in atrial fibrillation can usually be governed by ventricular response, which should not normally be allowed to fall below 60 beats/min. Digoxin plasma concentrations during maintenance treatment are not necessary unless problems are suspected. They relate only partially to the therapeutic effect, because so many other factors can contribute to myocardial excitability (e.g. electrolyte concentrations, catecholamines and hypoxia). The likelihood of toxicity increases progressively through the range 1.5–3 micrograms/L.
Complications of digoxin therapy.
Digoxin treatment is particularly hazardous because the toxicity of digoxin is difficult to recognise and it has the potential to cause fatal arrhythmias. The drug’s toxicity is more pronounced in the presence of metabolic and electrolyte disturbances (especially hypokalaemia but also hypomagnesaemia, hypercalcaemia, alkalosis, hypothyroidism and hypoxia). The patient’s renal function should be taken into account when deciding the digoxin dose in order to minimise the risk of toxicity. Excessive digoxin dose should be considered in any patient who is unwell or who has suddenly deteriorated. Important signs of toxicity are abdominal pain, nausea/anorexia, tiredness/weakness, diarrhoea, confusion and any change in vision, mobility or mood. Toxicity can often be managed by discontinuing therapy and correcting hypokalaemia if appropriate. Serious occurrences require urgent specialist management. Digibind, digoxin-specific antibody fragments in injectable form, is available for reversal of life-threatening overdosage.
PHOSPHODIESTERASE INHIBITORS
Enoximone and milrinone are also used in the management of cardiac failure. They are both selective phosphodiesterase inhibitors exerting their effect on the myocardium. This increases cellular calcium ion concentration, enabling the muscle fibres to contract with greater force. The heart pumps out blood more efficiently, thus reducing the strain of congestion in a failing heart. Haemodynamic benefit has been noted after administration, but there is no evidence to date to show benefit on survival rates. They are both given intravenously for short-term use only.
DIURETICS
There are a number of different diuretics that produce the same end result but through a different mode of action (Fig. 12.3). In order to understand their differing modes of action, it is necessary to have a basic understanding of the physiology of the kidney.
PHYSIOLOGY OF THE KIDNEY
Each kidney is made up of approximately 1 million nephrons, each nephron comprising a glomerulus and a proximal and distal tubule that are connected by the loop of Henle (see Ch. 18). The glomerulus consists of a group of capillaries, and as the blood passes through these it is filtered. A large amount of water and dissolved salts is filtered from the blood and passes on to the tubules. In the tubules, a selective reabsorption takes place. Glucose is normally completely reabsorbed. Water and electrolytes, including sodium, potassium, chloride and bicarbonate, are selectively reabsorbed and pass back into the circulation. Urea, excess water, salts and other unwanted substances are excreted as urine. The exact amount of each substance excreted in the urine is controlled in order to maintain the composition of the body fluids at normal levels. The urine is further concentrated and, depending on the electrolyte balance, more sodium is absorbed in exchange for potassium. In the distal tubule, antidiuretic hormone (ADH, vasopressin) excreted by the posterior pituitary gland is an important controlling factor. Increased ingestion of water results in an increased urine flow. When water is absorbed from the gastrointestinal tract, it causes the plasma to become more dilute, and this in turn decreases the release of ADH by the posterior lobe of the pituitary gland. Less ADH reaches the kidney, and this causes the tubules to reabsorb less water so that more is excreted as urine (see Ch. 8 for renal drug excretion).
DISEASE STATES RESULTING IN OEDEMA
In health, the kidney maintains the composition of the blood within narrow limits. Disease can upset this delicate balance. When the blood supply to the glomerulus is reduced because of impaired blood circulation, filtration slows. However, tubular reabsorption continues at the normal rate, and excess water and salts accumulate in the body tissues, resulting in oedema. This can arise in cardiac failure. Oedema can also arise in renal disease (nephrotic syndrome) and cirrhosis of the liver.
Diuretics are used in the treatment of these conditions and also in the treatment of hypertension. They cause a net loss of sodium and water from the body by decreasing the reabsorption of sodium and chloride. Because a large proportion of the salt and water that passes into the tubule is reabsorbed, a small decrease in reabsorption can result in a marked increase in excretion.
THIAZIDE AND RELATED DIURETICS
Mode of action.
Thiazide diuretics act by inhibiting the reabsorption of sodium and chloride in the distal tubule of the nephron, resulting in increased sodium, chloride and water secretion. There is also an increased secretion of potassium. The mode of action in relieving hypertension is unclear.
Pharmacokinetics.
These diuretics are well absorbed orally and are excreted unchanged by the kidney. Compared with loop diuretics (see later), the potency is lower, with a slow onset and longer duration of action. Because the duration of action is about 12 h, a thiazide diuretic should be given in the morning.
Indications.
Thiazide diuretics such as bendroflumethiazide (bendrofluazide), cyclopenthiazide, indapamide, metolazone and xipamide are used to treat hypertension and oedema resulting from cardiac failure, liver disease and nephrotic syndrome. In the management of hypertension, a low dose of a thiazide (e.g. bendroflumethiazide 2.5 mg daily) produces a near-maximal blood pressure–reducing effect with very little biochemical disturbance. Higher doses may cause more marked changes in plasma potassium, uric acid, glucose and lipids, with no advantage in blood pressure control, and should not be used. Optimal doses for the control of heart failure may be larger, and long-term effects are of less importance. Metolazone is particularly effective when combined with a loop diuretic. Profound diuresis may occur, and therefore the patient should be monitored carefully.
Adverse effects.
The most important adverse effects are hypokalaemia, hyponatraemia and dehydration. Hypomagnesaemia may also occur. Impotence is reversible on withdrawal of treatment. Competition with uric acid for secretion into the proximal tubule may cause hyperuricaemia, and this may result in gout. Impaired glucose tolerance can occur. Hypotension, headache and dizziness may be experienced.
Hypokalaemia is a common side-effect of thiazide diuretics, particularly at higher doses. Combination products are available containing diuretic plus potassium, but the quantity of potassium is insufficient to correct hypokalaemia. When potassium supplements are necessary, these should be given routinely in tablet or liquid form. Alternatively, the addition of a potassium-sparing diuretic can alleviate the need for supplementation.
LOOP DIURETICS
Mode of action.
This group of diuretics gets its name because sodium and chloride reabsorption in the ascending limb of the loop of Henle is inhibited. In addition, potassium secretion in the distal tubule is increased (greater with furosemide (frusemide) than with bumetanide).
Pharmacokinetics.
Bumetanide is almost completely absorbed after oral administration, while furosemide is 60–70% absorbed. Bumetanide is partially metabolised in the liver, and about 80% of a dose is excreted in the urine – 50% as unchanged drug. Furosemide is excreted primarily unchanged in the urine. Onset of action occurs in 30 min after an oral dose and within a few minutes of intravenous administration. The duration of action lasts between 3 and 6 h after an oral dose.
Adverse effects.
The most important adverse effects are hypokalaemia, hyponatraemia and dehydration. Hypokalaemia may be treated with potassium supplements or potassium-sparing diuretics. Other side-effects include hypotension, nausea, gastrointestinal disturbances, hyperuricaemia and gout. Tinnitus and deafness may occur with large parenteral doses and rapid administration, particularly with furosemide.
POTASSIUM-SPARING DIURETICS
Mode of action.
Amiloride and triamterene cause increased sodium and chloride excretion in the distal tubule, resulting in an increase in water excretion. The secretion of potassium in the distal tubule is inhibited (unlike thiazide and loop diuretics). The potassium-sparing diuretics have weaker diuretic and antihypertensive effects than other diuretics, but they have the advantage of conserving potassium. For this reason, they are often prescribed with thiazide or loop diuretics.
Pharmacokinetics.
Following oral administration, approximately 50% of triamterene and 20% of amiloride is absorbed. Triamterene is extensively metabolised by the liver, but amiloride is excreted unchanged in the urine.
Indications.
Amiloride and triamterene are used on their own or, more usually, in combination with thiazide or loop diuretics in the treatment of oedema, as they are weak diuretics. Dose will be dependent on indication and whether they are used in combination with other diuretics.
ALDOSTERONE ANTAGONIST: POTASSIUM-SPARING DIURETIC
Spironolactone and eplerenone are both aldosterone antagonists. Spironolactone is also used as a potassium-sparing diuretic.
Mode of action.
Spironolactone is metabolised to canrenone, which is an antagonist of the action of aldosterone on the distal tubule of the nephron. Aldosterone promotes the retention of sodium and the excretion of potassium by the kidneys. Canrenone reverses this effect, causing increased excretion of sodium and water and retention of potassium. Eplerenone prevents the binding of aldosterone. Eplerenone has relative selectivity in binding to mineralocorticoid receptors compared with its binding to glucocorticoid, progesterone and androgen receptors. As a consequence, the Scottish Medicines Consortium has recommended that eplerenone should be restricted to patients who cannot tolerate the hormonal side effects of spironolactone.
Pharmacokinetics.
Spironolactone is well absorbed (approximately 70%). It is metabolised to its active form, canrenone, in the liver. The onset of action occurs in 2–4 h. It has a long duration of action (up to 96 h), and its maximum effect takes several days to occur. The absolute bioavailability of eplerenone is unknown. Maximum plasma concentrations are reached after about 2 h, and a steady state is reached within 2 days. Absorption is not affected by food.
Indications.
Spironolactone is used to treat fluid retention due to cardiac failure, the nephrotic syndrome and hepatic disease. In the first two con-ditions, it is usually used in combination with a thiazide or loop diuretic. In oedema caused by excessive aldosterone activity (e.g. cirrhosis of the liver, primary aldosteronism), it counteracts these effects by competing with aldosterone for receptor sites. Eplerenone is used, in addition to standard therapy, to reduce the risk of cardiovascular mortality and morbidity in stable patients with left ventricular dysfunction and clinical evidence of heart failure after recent MI.
GENERAL PRECAUTIONS TO BE OBSERVED IN THE USE OF DIURETICS
Caution must be exercised when administering diuretics to patients with impaired liver or kidney function and also to patients suffering from diabetes. The patient should always be observed for signs of fluid and electrolyte imbalance.
Diuretics can cause acute toxic reactions in patients to whom digitalis glycosides or non-depolarising muscle relaxants have already been administered, by depleting serum potassium. They also enhance the effects of antihypertensive drugs (e.g. methyldopa, hydralazine), and this enables the dose of these drugs to be reduced when treating hypertension.
The thiazides and loop diuretics can lead to potassium depletion. In these cases, potassium supplements may have to be given.
POTASSIUM SUPPLEMENTS
The potassium loss produced by certain diuretics may lead to a severe degree of hypokalaemia, with its associated muscle weakness, mental disturbances, cardiac effects and increased risks of digitalis toxicity. Potassium supplements may be given with the thiazide and loop diuretics, but combination therapy with a potassium-sparing diuretic is usually preferred. Potassium chloride in solution is poorly tolerated because of its nauseous effects and it is not often used. Apart from the use of diuretics, deficiency of potassium may occur for various reasons (e.g. ulcerative colitis, diarrhoea, vomiting), but the most common cause is diuretic therapy.
ANTIARRHYTHMIC DRUGS
DISORDERS OF CONDUCTION
Under certain circumstances, the cycle of contraction and relaxation of the heart may be disturbed. These disturbances are known as cardiac arrhythmias. There are several types of arrhythmia for which antiarrhythmic drugs are used. An antiarrhythmic drug is a drug that is used to control or correct abnormal rhythms of cardiac action. These drugs may be used for several different types of cardiac arrhythmia, and it is essential that the specific type of arrhythmia is diagnosed by electrocardiogram prior to commencement of treatment.
SUPRAVENTRICULAR ARRHYTHMIAS
As their name implies, these involve arrhythmias arising from above the ventricles and are normally tachyarrhythmias (i.e. faster than normal). The most common of these are atrial flutter and atrial fibrillation, which are often a result of ischaemic heart disease.
Atrial flutter and atrial fibrillation.
Atrial flutter is very rapid, but regular, contractions of the atria of the heart that the ventricles may follow exactly, producing a very fast, regular pulse. Atrial fibrillation is an arrhythmia that may develop following atrial flutter and involves very rapid (400–600 per min) disordered contractions of the atria. This results in irregular stimulation of the AV node and, coupled again with AV block, a fast irregular pulse. Either of these conditions may lead (sometimes rapidly) to exhaustion of the myocardium, other arrhythmias and cardiac failure. In both atrial flutter and atrial fibrillation, AV block develops because of, and its degree is determined by, the refractoriness of the bundle of His and the AV node. After each stimulation, there is a period during which the fibres cannot be restimulated, and this is known as the refractory period.
In atrial fibrillation and flutter, messages from the atria will reach the AV node during this refractory period and hence be missed. The length of the refractory period will determine how many atrial beats are blocked for each that is passed, and hence the ventricles may contract only for every second, third, fourth or more atrial beats.
Ectopic beats.
Ectopic beats, also called premature beats or extrasystoles, arise from a focus other than the pacemaker. They rarely require drug treatment, but it should be remembered that they may precipitate other types of arrhythmia. In certain cases, they do become troublesome and may be treated with beta-adrenoceptor blocking drugs.
Supraventricular tachycardias.
These tachycardias may arise for a number of reasons, for example following MI, in patients with thyrotoxicosis or in patients suffering from Wolff–Parkinson–White syndrome. (This is a condition whereby impulses are conducted not only through the AV node but also through an anomalous pathway connecting the atria to the ventricles.) Paroxysmal supraventricular tachycardia (coming on in sudden bursts) does not usually require drug treatment, and normal rhythm can often be achieved by, for example, respiratory manoeuvres, prompt squatting or pressure over one carotid sinus.
VENTRICULAR ARRHYTHMIAS
Ventricular tachycardia is potentially more dangerous than supraventricular tachycardias. These arrhythmias are common following acute MI or can be precipitated by supraventricular tachycardias. A ventricular ectopic beat may initiate ventricular tachycardia, followed by ventricular fibrillation with loss of cardiac output and death.
BRADYCARDIA
Bradycardia is a slowing of the heart, is supraventricular in origin, and may occur, for example, following MI or can be a side effect of beta-adrenoceptor blocking drugs.
THE CARDIAC ACTION POTENTIAL
Antiarrhythmic drugs are used to treat abnormal electrical activity of the heart. To understand the actions of these drugs, it is necessary first to examine the cardiac action potential (Fig. 12.4).

Fig. 12.4 Phases of an action potential in the Purkinje fibre cell and the cationic changes that take place.
Phase 4.
This is the resting phase in cells normally capable of spontaneous depolarisation (cells in SA node, AV node and His–Purkinje system). There is a voltage difference across the surface membrane of all myocardial cells that is called the resting trans-membrane voltage or potential. During the resting phase, there is a slow drift from the maximum negative potential (around 290 mV) to a potential of about 270 mV. This is due to a small influx of sodium ions into the cell and a small efflux of potassium ions. This depolarisation is spontaneous and continues until it reaches the threshold at which an action potential is initiated automatically (phase 0). Atrial and ventricular cells do not normally exhibit spontaneous depolarisation but remain at rest (diastole) until stimulated by a propagatory impulse.
Phase 0.
When the threshold potential is reached and the cell is stimulated, there is a very rapid influx of sodium ions into the cell, causing a rapid rise in transmembrane potential (depolarisation) until it reaches a given value (above 120 mV in Purkinje fibres). This inward current of sodium is very intense and very brief.
ANTIARRHYTHMIC DRUGS
These drugs limit cardiac electrical activity to normal conduction pathways and decrease abnormally fast heart rates. They may be classified in a number of ways:
A drug may show more than one of the classes of antiarrhythmic action. The main function of this classification is to define drugs with similar modes of action and to identify possible antiarrhythmic compounds by their effects on cardiac conduction. The clinical value of this classification is limited, and it excludes some antiarrhythmic agents such as the cardiac glycosides.
TREATMENT OF SUPRAVENTRICULAR ARRHYTHMIAS
Adenosine causes rapid reversion of sinus rhythm of paroxysmal supraventricular tachycardias. It is given by rapid intravenous injection into a large peripheral vein. The dose is 3 mg over 2 s, with cardiac monitoring. If required, this is followed by 6 mg after 1–2 min, then by 12 mg after a further 1–2 min. Adverse effects include transient facial flush, dyspnoea, lightheadedness, choking sensation and nausea.
Cardiac glycosides are the treatment of choice in slowing ventricular response in atrial flutter or atrial fibrillation.
Verapamil is usually effective for supraventricular tachycardias. An initial intravenous dose may be followed by oral treatment. It should not be injected into patients recently treated with beta-blockers, because of the risk of hypotension and asystole.
TREATMENT OF SUPRAVENTRICULAR AND VENTRICULAR ARRHYTHMIAS
Amiodarone should be initiated only under hospital or specialist supervision. It is used for treatment of other arrhythmias when previous treatments have failed (e.g. paroxysmal supraventricular, nodal and ventricular tachycardias; atrial fibrillation and flutter; and ventricular fibrillation). It may also be used to treat tachycardia associated with the Wolff–Parkinson–White syndrome (this is a congenital abnormality occurring in about 0.2% of the population and results from an additional conducting system between the atria and the ventricles). Amiodarone can be given orally or by intravenous infusion. It has a long half-life (30–45 days). Its onset of action may take 1–2 weeks, and effects can be seen months after the drug is withdrawn. Most patients on amiodarone develop corneal microdeposits, usually reversible on withdrawal of the drug. This may lead to drivers being dazzled by headlights at night. As it contains 37% iodine, it can affect thyroid hormone metabolism. Pulmonary toxicity may also be a problem and can result in pneumonitis and fibrosis.
The antiarrhythmic properties of beta-blockers are conferred mainly through their β1-blocking actions, which oppose the electrophysical effects of catecholamines and raise the threshold for ventricular fibrillation. The different beta-blockers have different selectivity for the two receptors β1 and β2. For example, atenolol, acebutolol and bisoprolol are relatively β1-selective when compared with propranolol, oxprenolol and sotolol, which have equal activity for β1 and β2. Beta-blockers are useful in pre-venting arrhythmias induced by exercise, emotion or anaesthesia, and for controlling the ventricular rate in atrial fibrillation. Intravenous esmolol gives rapid beta blockade of short duration (a few minutes) and is particularly useful for the rapid control, for instance, of perioperative tachyarrhythmias. Sotalol has specific antiarrhythmic properties. It may reverse or prevent recurrence of atrial fibrillation and paroxysmal junction tachycardia associated with the Wolff–Parkinson–White syndrome (see below) and may prevent recurrent life-threatening ventricular arrhythmias. However, sotalol may itself cause serious ventricular arrhythmia, including torsades de pointes, especially in patients with depressed left ventricular function, hypokalaemia, or if given with other drugs that prolong the QT interval. Sotalol should be reserved for patients with serious arrhythmias likely to benefit specifically from its antiarrhythmic actions.
Disopyramide is very effective against ventricu-lar extrasystoles and is also used for ventricular arrhythmias, especially where MI is suspected or has been proven. It suppresses the frequency of ectopic ventricular beats as well as the frequency and duration of self-limiting bursts of ventricular tachycardia. It can be given orally or by intravenous infusion. Too rapid an infusion rate can lead to hypotension and cardiac failure. It has anticholinergic side effects, including dry mouth and blurred vision.
Flecainide is of value for serious symptomatic ventri-cular arrhythmias and paroxysmal atrial fibrillation. It delays intracardiac conduction, but it may precipitate serious arrhythmias in certain patients. It should be initiated in hospital under specialist supervision.
Procainamide is used to control ventricular arrhythmias. The rate of metabolism will depend if the patient is a fast or slow acetylator. (Variation in the N-acetyltransferase gene divides people into slow acetylators and fast acetylators [see Ch. 8], with very different half-lives and blood concentrations of such important drugs as isoniazid as well as procainamide.)
Quinidine may be effective in suppressing supraventricular and ventricular arrhythmias and is rarely used now. It is best used under specialist advice.
TREATMENT OF VENTRICULAR ARRHYTHMIAS
Lidocaine is the first choice in an emergency. In patients without gross circulatory impairment, 100 mg is given as a bolus over a few minutes (50 mg in lighter patients or those whose circulation is severely impaired); this is followed immediately by infusion of 4 mg/min for 30 min, 2 mg/min for 2 h, then 1 mg/min. The infusion concentration is reduced further if continued beyond 24 h. It is effective in suppressing ventricular tachycardia and reducing the risk of ventricular fibrillation following MI. It can be given only by slow intravenous injection, as it is not available in an oral formulation due to a high first-pass metabolism effect. Although effective when used in an emergency, it has not been shown to reduce mortality when used prophylactically. In patients with cardiac or hepatic failure, doses may need to be reduced to avoid convulsions, depression of the central nervous system, or depression of the cardiovascular system. It can cause central nervous system disturbances, including convulsions, drowsiness and paraesthesia.
Mexiletine may be given as a slow intravenous injection if lidocaine is ineffective, and it has a similar action. Unlike lidocaine, it is well absorbed from the gastrointestinal tract. Adverse cardiovascular and central nervous system effects may limit the dose tolerated; nausea and vomiting may prevent an effective dose being given by mouth. Its effect is antagonised by hypokalaemia.
MANAGEMENT OF ARRHYTHMIAS
Management of an arrhythmia, apart from treatment of the associated heart failure, requires precise diagnosis of the type of arrhythmia, and an electrocardiograph is essential. In atrial fibrillation, the ventricular rate can usually be controlled with digoxin. A beta-blocker or verapamil may be added if control is inadequate. In valvular or myocardial disease, anticoagulants may be indicated. In atrial flutter, the ventricular rate can similarly be controlled by digoxin. Synchronised direct current shock can be utilised when reversion to sinus rhythm is indicated.
Paroxysmal supraventricular tachycardia usually remits spontaneously or can be returned to sinus rhythm by reflex vagal stimulation, by prompt squatting or respiratory control. If drug treatment is required, intravenous adenosine is usually the first choice. Digitalisation, an intravenous beta-blocker, intravenous verapamil or direct current shock may be tried.
When arrhythmias occur after MI, lidocaine should be given intravenously. Bradycardia, especially if complicated with hypotension, should be treated with intravenous atropine sulphate (0.3–1 mg).
β-ADRENOCEPTOR BLOCKING DRUGS
Adrenaline (epinephrine) and noradrenaline (nor-epinephrine), which are produced by the adrenal glands and at sympathetic nerve endings, exercise their physiological actions via a and beta adrenoceptors (see Ch. 9).
Stimulation of β1 adrenoceptors in the heart and coronary arteries will lead to an increase in heart rate, increase in conduction velocity and force of contraction in the heart, and vasodilatation of coronary arteries. Excitation of β2 adrenoceptors will lead to dilatation of peripheral arteries.
Beta adrenergic blocking agents interfere with catecholamine binding at beta adrenoceptors. Several beta-blockers (acebutolol, atenolol, bisoprolol and metoprolol) are said to be cardioselective. These agents have the ability to antagonise the action of catecholamines at β1 receptors at doses smaller than those required to block β2 receptors. They are not, however, cardiospecific. They have a smaller effect on airways’ resistance but are not free of this side-effect. Others block both β1 and β2 receptors (i.e. cardiac plus bronchial plus peripheral blood vessel receptors) and are called non-selective beta-blockers. Blocking β2 receptors causes bronchospasm, which may be of little consequence in normal subjects but in asthmatic patients may make bronchospasm worse and increase dyspnoea. Beta-blockers should be used only in patients with asthma or in those with a history of obstructive airways disease when no alternative treatment is available.
Some beta-blockers (pindolol, oxprenolol, acebutolol and celiprolol) demonstrate various degrees of intrinsic sympathomimetic activity, which represents the capacity of beta-blockers to stimulate as well as block adrenergic receptors. These drugs cause a slight agonist response at the beta receptor while blocking the effect of endogenous catecholamine. Patients given a drug with intrinsic sympathomimetic activity experience a smaller reduction in resting heart rate than those receiving a beta-blocker without intrinsic sympathomimetic activity. They tend to cause less bradycardia and less coldness of the extremities than the other beta-blockers, which is a problem particularly in patients with peripheral vascular disease.
Some beta-blockers are water-soluble and some are lipid-soluble. Lipophilic beta-blockers are able to cross the blood–brain barrier and exert effects on the central nervous system. Nightmares and hallucinations are more of a problem with the lipophilic agents. The most water-soluble are atenolol, celiprolol, nadolol and sotalol. They are less likely to enter the brain and may therefore cause fewer sleep disturbances and nightmares. Water-soluble beta-blockers are excreted by the kidneys, and dose reduction may be required in renal impairment.
All beta-blockers slow the heart; the output of blood is reduced and the work done by the heart is thus decreased. They should not therefore be given to patients with heart block.
Labetalol is a mixed alpha-and non-selective beta-adrenergic antagonist that reduces peripheral resistance but has little effect on heart rate or cardiac output. Positive hypotension occurs. Labetalol may be useful in hypertension of pregnancy and in patients with renal failure.
Beta-blockers are contraindicated in asthma or obstructive airways disease, second- or third-degree heart block, sinus bradycardia, sick sinus syndrome, severe peripheral arterial disease and uncompensated cardiac failure.
The side effects of beta-blockers include fatigue, cold extremities, bronchoconstriction, interference with autonomic and metabolic responses to hypoglycaemia, bradycardia, heart block, negative inotropic effect and impotence.
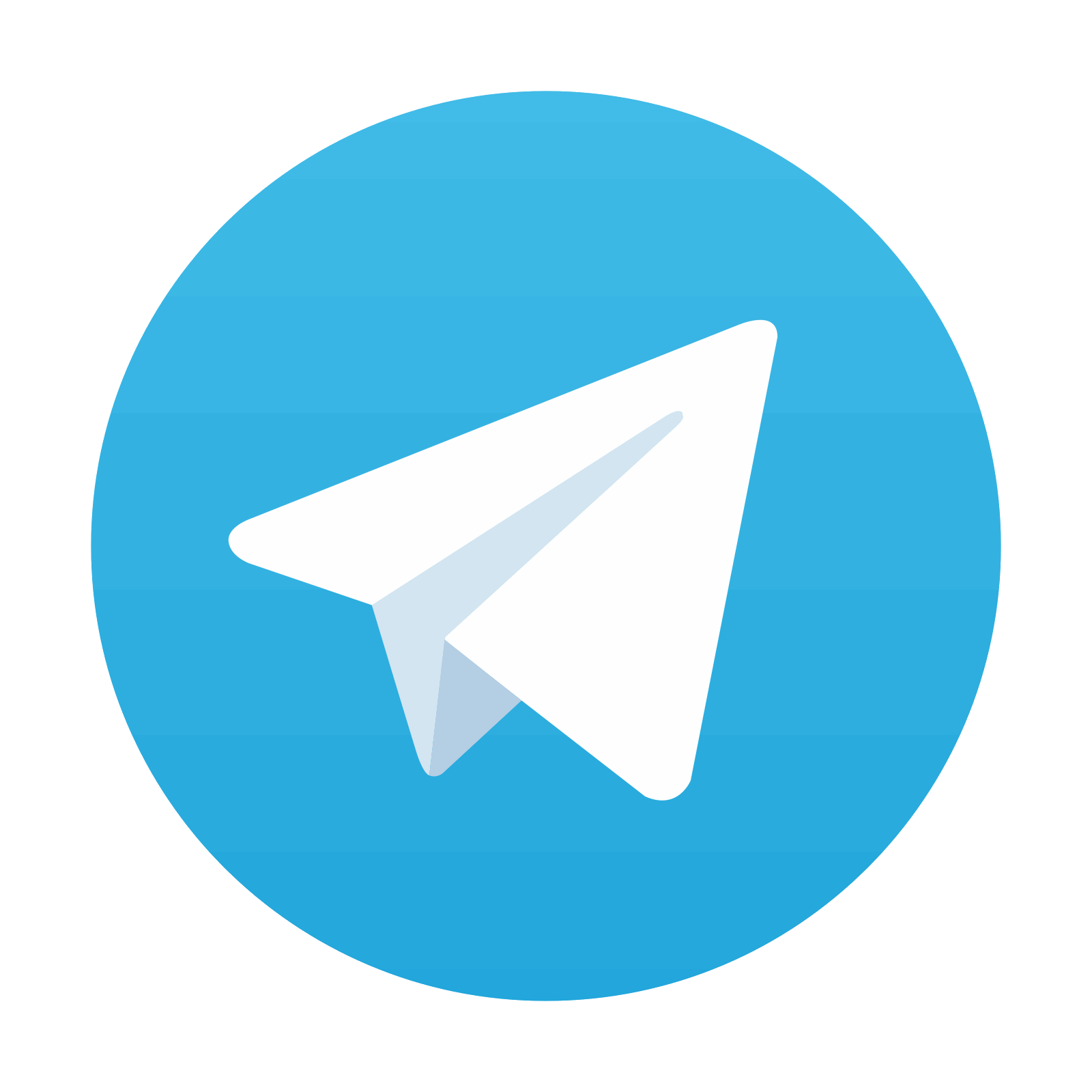
Stay updated, free articles. Join our Telegram channel

Full access? Get Clinical Tree
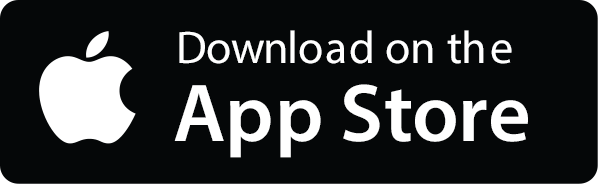
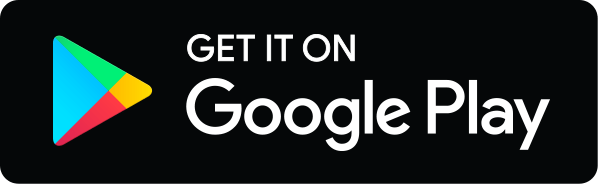