Cancer Pathophysiology
Cancer is a genetic disease in which the regulation, characteristics, and functions of normal cells are altered. Genes provide the instructions for making proteins and regulate when and where a protein will be produced. Proteins perform many functions essential for normal cellular functioning. During protein synthesis, deoxyribonucleic acid (DNA) serves as codes for the production of amino acids and proteins. Genetic mutations may occur during DNA and protein synthesis, which allow malignant cells to gain a selection advantage over normal cells, enabling them to grow uncontrollably. Malignant transformation is influenced by multiple factors, including oncogenes, overexpression of growth factors (GFs), defective intracellular signal transducers, and cell membrane changes.
NORMAL CELLULAR BIOLOGY
Normal Cells
Genotypic Characteristics.
Gene storage and activity occurs in the nucleus of cells. Each normal cell contains all the genetic material (i.e., genes or DNA) that is appropriate for the species. During the M phase of the cell cycle, genes can be visualized as condensed matter, called chromosomes. Euploid is the term that signifies the normal number of chromosomes for a species. In humans, the euploid number of chromosomes is 46. The exception to this is the unfertilized egg and sperm, which contain 23 chromosomes. Therefore, normal human cells contain 46 chromosomes (22 pairs of [nonsex] autosomes, and one pair of sex chromosomes). The sex cells are considered haploid because they contain only half of each pair of chromosomes. Mature erythrocytes have extruded their nuclei during the maturation process and contain no chromosomes.
Embryonic Cells
Commitment.
At a predetermined point in early embryonic development, the cells initiate the steps to become differentiated. This is thought to occur on or about postconception day 8 (National Institutes of Health [NIH], 2001). In response to an unknown signal(s), each cell commits itself to a specific maturational outcome and positions itself within a group of cells that will eventually take on a specific morphology and functional behavior. Differentiation does not involve the loss of any genes because all committed cells retain the same number of genes present in the fertilized egg. Differentiation is a function of the selective suppression and expression of individual genes. Commitment also involves suppression (turning off) of the specific genes that regulated and directed the rapid growth of early embryonic cells and expression (turning on) of other specific genes to initiate cellular differentiation (e.g., nerve cells, bone cells, muscle cells). The genes that are selectively expressed determine the specific type of tissue that each embryonic cell will become.
GENETICS AND CANCER
Molecular Genetics
Genes, the smallest functional units of inherited information in living organisms, are the controlling factors for cellular development and function. Genes provide the instructions for making proteins, using different combinations of amino acids, and regulate when and where a protein will be produced (regulatory sequence). The process of making proteins is called protein synthesis. Proteins perform a host of biological functions. Some proteins, called enzymes, act to catalyze (speed up) chemical reactions within or between cells. Other proteins support the cytoskeleton of the cell, play a role in immune responses, or participate in the storage or transport of ligands. Some cells transport proteins that are used by other cells or used to direct the activity of other cells. In short, proteins play essential roles in cellular maintenance, growth, and function (see table below).
DNA Synthesis
DNA is a nucleic acid located in the nucleus of each cell as a condensed, compact structure that contains codes for the construction of every protein made in the body. The helix-shaped form of DNA is actually a pair of molecules consisting of polymers (long chains) of interlocking nucleotides called chromatin. The nucleotide molecules are chemical building blocks consisting of a phosphate, a five-carbon sugar, and a base (adenine [A], guanine [G], thymine [T], or cytosine [C]). The arrangement of the bases can be likened to a genetic alphabet that is used to create the language of intercellular and intracellular communication. (See figure on page 18).
The two strands of DNA are aligned as two complementary threads, held in close proximity by hydrogen bonds. These bonds are disrupted when the cell undergoes DNA or protein synthesis. The bases in the two adjoining strands can only pair up with one single predetermined base. Two nucleotides, paired together are termed a base pair. A pairs with thymine; T and G pair with C. The only possible combinations of the bases are A and T, T and A, C and G, and G and C. A on one strand of DNA can only pair up or mate with the T on the complementary strand. Therefore, if the sequence of nucleotides on one DNA strand is known, the order of the complementary DNA strand can be predicted accurately. One additional nucleotide, uracil (U) is rarely seen in DNA except in some viruses, where it can replace T (see figure on page 19). There are approximately three billion base pairs in the human genome. Base pairing ensures the structural integrity of DNA and is essential for the storage, retrieval, and transference of genetic information.
The intertwined double strands of DNA are antiparallel (run in opposite directions) with the asymmetric ends (referred to as “five prime” [5′] and “three prime” [3′]) winding around a helix axis in a right-handed spiral. In a vertical double helix, 3′ is designated as “ascending” and 5′ is designated as “descending.” A polymerizing enzyme attaches itself to one strand and descends from the 5′ to the 3′ direction. As the enzyme moves along the strand, it “reads” the base sequence and forms a new string of DNA complementary to the template strand. After the strands of DNA are replicated, they condense into supercoiled chromosome sets that split to become part of two new daughter cells.
Common Terms Used in Molecular Genetics
Component | Function |
---|---|
Amino acid | Basic building units from which all proteins formed. There are 20 standard and two nonstandard amino acids in the genetic code. |
Base pairs | The DNA nucleotide is composed of a molecule each of phosphoric acid, sugar, and a base. The bases are coded with the letters A, T, G, and C. These letters represent, respectively, the chemicals adenine, thymine, guanine, and cytosine. |
Cadherin | Protein expressed on the surface of the cell. Plays an important role in cell adhesion |
Codon | A group of three bases in an RNA strand located on mRNA. Each codon represents a particular amino acid. Each codon on mRNA has a complementary anticodon on tRNA so that tRNA recognizes the correct binding site. |
DNA | Deoxyribonucleic acid. Codes for amino acids, which form proteins that carry out cell functions. Composed of a phosphate, base (cytosine, guanine, adenine, guanine), and a sugar (deoxyribose). |
Enzyme | A protein that catalyzes (speeds up) chemical reactions in cells |
Exon | Region of a gene that contains part of the code for producing protein. Each exon codes for a specific portion of the complete protein. |
Gene | Repository of heredity. Located in DNA and RNA and encoded with genetic information. |
Growth factor | Protein that binds to receptors of the surface of a cell that stimulates cellular proliferation and differentiation |
Hilacases | Enzymes that split the hydrogen bonds that connect the two strands of DNA together |
Integrin | Protein that plays a role in the attachment of a cell to the ECM and in signal transduction from the ECM to the cell |
Intron | Section of RNA that is deleted because it is not needed during protein synthesis |
Ligand | A molecule that interacts with a protein by binding to the protein |
Nucleic acid | A complex molecule made up of nucleotide chains that transmit genetic information (e.g., DNA, ribonucleic acid) |
Nucleotide | Basic unit of a polynucleic chain made up of a phosphate, a base (cytosine, guanine, adenine, guanine), and a sugar. Cytosine pairs with guanine; adenine pairs with thymine. Uracil takes the place of thymine in RNA. |
Peptide | Small molecules formed by the linking (in a specific sequence) of amino acids |
Polymer | Molecules composed of repeating structural units connected by covalent chemical bonds (e.g., proteins are polymers of amino acids) |
Protein | Organic compound consisting of amino acids joined by peptide bonds. Proteins are polypeptide molecules or consist of polypeptide subunits. |
RNA | Ribonucleic acid. Composed of a phosphate, a base (uracil, cytosine, adenine, guanine), and a sugar (ribose) |
mRNA | Messenger ribonucleic acid. Carries a copy of the genetic information stored in DNA to the ribosomes, to be made into protein |
rRNA | Ribosomal RNA. A structural component of the ribosome that has complementary sequences that bind to regions of mRNA. |
tRNA | Transfer ribonucleic acid. Carries the amino acid components of a protein to the appropriate place as coded for on mRNA |
Protein Synthesis
Protein synthesis consists of two phases: transcription and translation. Initially, the cells receive a message to produce a specific protein. During transcription, the portion of the DNA that contains the genetic code for producing the protein uncoils (“unzips”) as occurs during cell division. Nucleotides then move along the strand of exposed gene and transcribe the subunits of DNA. The new strand is an RNA molecule referred to as messenger RNA (mRNA). The exposed DNA “zips” closed and remains in the nucleus of the cell. Before the transcripted mRNA moves into the cytoplasm, nuclear enzymes remove introns (noncoding sections, also called “junk DNA”) and splice together exons (the working sequences that code for proteins) (see figure on page 20).
Next, the genetic copies (i.e., mRNA) enter the cytoplasm through channels (pores) in the nucleus, bind to ribosomal RNA (rRNA) found in the cytoplasm, and are decoded. The mRNA is encoded with information on the particular arrangement of amino acids that will make up each protein. During translation, a molecule of transfer RNA (tRNA) matches up to the strand of mRNA and carries the correct amino acids to the ribosome (see figure on page 20). Three nucleotide bases (codons) are read at a time, each representing a specific amino acid (see figure on page 20). As each codon is decoded, the corresponding amino acid is activated. The amino acids are brought into the proper sequence once the entire message is read, and the newly formed polypeptide chain folds into its final three-dimensional shape. The total number of amino acids in a specific protein and the exact code that links them together determines the nature and activity of the protein. Different sequences of amino acids change the shape of the proteins and therefore their functions (see figure on page 21).
Neoplasia
Neoplasia is a term designated to signify a new growth of cells in the body. Although all neoplasms are considered to be abnormal, they are designated as either benign (noncancerous) or malignant (cancerous). All tumors, whether benign or malignant, share two things in common: a parenchyma (functional part) that contains proliferating neoplastic cells and a stroma (supportive structure) consisting of connective tissue and blood vessels (Kumar, Abbas, & Fausto, 2005).
Benign Neoplasia.
Benign neoplastic cells enter and progress through the phases of the cell cycle in the same fashion as normal cells. Benign neoplastic cells arise from normal cells and tend to retain most of the properties of the cells from which they arose. At the microscopic level, benign cells are euploid, containing the normal chromosome complement. However, because their behavior is not completely normal, it is likely that some alteration in gene regulation is present. Benign neoplastic cells are characterized by increased proliferation or decreased apoptosis (programmed cell death). A major difference in growth characteristics between benign neoplastic cells and normal cells is that density-dependent contact inhibition has been lost. Therefore, benign neoplastic cells continue to grow by expansion into the surrounding tissue.
Benign neoplastic tissues are typically well differentiated. In essence, they are morphologically and functionally similar to the normal tissues from which they arose. They retain a small nuclear/cytoplasmic ratio and continue to synthesize fibronectin, as do normal cells. Like normal cells, benign neoplastic cells adhere tightly and do not metastasize. Even so, benign neoplastic growth can cause physiological dysfunction and death. For example, a benign tumor can exert pressure on nerves and blood vessels or obstruct lumens (e.g., trachea, colon) (Kumar, Abbas, & Fausto, 2005).
Malignant Neoplasia.
Proteins play an important role in the development of malignant disease. The normal cell cycle is regulated by proteins called cyclins, cyclin-dependent kinases, and cyclin-dependent kinase inhibitors. The role of these proteins is to provide cell-cycle checkpoints to control the signals that coordinate cell reproduction. Checkpoints occur in late G1 before DNA synthesis, during the S phase when DNA content is duplicated, and in G2 before cells become completely committed to mitosis. These checkpoints occur at times during the cell cycle when cells are most vulnerable to the harmful effects of DNA (genetic) damage. The checkpoints allow cells to make DNA repairs and remove damaged molecules before they threaten the survival of the organism (Reed, 2005; Rettig & Sawicki, 2001; Skaar & DeCaprio, 2006).
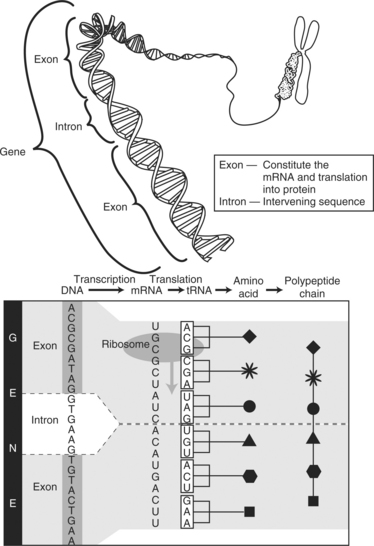
Protein synthesis—transcription and translation.
From the National Institutes of Health: National Human Genome Research Institute: www.genome.org.
Normal cells have multiple copies of specific DNA sequences and proteins at the ends of their chromosomes, called telomeres. Each time a cell divides, it loses a telomere, thus shortening the chromosome. When the chromosome is shortened to a predetermined length, the cell is signaled to enter the resting stage, also known as senescence. The cell remains viable but stops reproducing (Kashima et al., 2006). Normal cells in a culture plate have a limited life span and a specific number of doubling times before they enter senescence. In addition, they display contact inhibition. Once the cells reach the boundary of the culture dish, they stop proliferating.
Normal cells are anchorage dependent. With the exception of hematopoietic cells, they must be attached to a surface (substratum) to proliferate. Malignant (cancer) cells, by contrast, are immortal. In a culture medium, growth is not confined to the surface of the culture dish. When malignant cells reach the edges of the culture dish, they continue to grow on top of each other. Malignant neoplastic cells are also capable of growing without a supporting substratum. They grow easily in suspension in an agarose or other medium, whereas normal cells must adhere to the surface of the culture plate to grow. Thus, malignant cells have a loss of anchorage dependence.
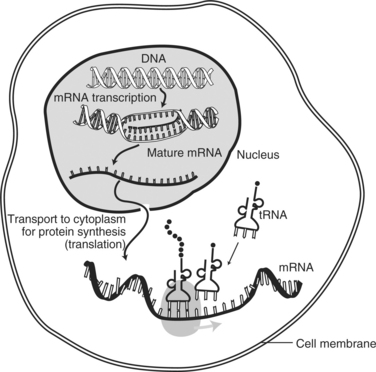
Protein synthesis within the cell.
From the National Institutes of health: National Genome Institute: www.genome.gov.
MALIGNANT TRANSFORMATION: CARCINOGENESIS
The process by which normal cells become cancer cells is called malignant transformation or carcinogenesis. Carcinogenesis is a multistep process that may take years to complete, and it is governed by a series of genetic or epigenetic changes. Genetic mutations may occur by deletion, duplication, inversion, insertion, or translocation (Baltzell, Eder, & Wrensch, 2005; Calvo, Petricoin, & Liotta, 2005; Rettig & Sawicki, 2001; Smith, Marks, & Lieberman, 2005) (see figure on page 22).
Genetic mutations are not uncommon and are usually repaired by cellular mechanisms. Cancer arises when defects in the genes that regulate cellular proliferation and differentiation, suppress tumor growth, target irreparably damaged cells for apoptosis, or repair damaged DNA are not corrected. As a result of these defects, cancer cells are not governed by the signals that regulate cell growth and may consequently exhibit uncontrolled proliferation (Calvo, Petricoin, & Liotta, 2005; Hoeijmakers, 2001; Taniwaki et al., 2006). Although cancer is sometimes caused by passing a defective gene to offspring, it is most often caused by mutations that occur over the course of a lifetime. These somatic (acquired, sporadic) mutations may result from a variety of factors that include physical, environmental, or chemical agents; ultraviolet (UV) radiation; viruses; or errors in replication that can cause base changes or breaks in the DNA strands. Acquired mutations are not passed on to offspring because these mutations are only found in the malignant neoplastic cell (Smith, Marks, & Lieberman, 2005; Stetler-Stevenson, 2005).
Oncogenes
Two types of genes are responsible for the development of cancer: oncogenes and tumor suppressor genes. Oncogenes are mutations of normal genes called proto-oncogenes that govern growth factors (GFs), GF receptors (GFRs), signal transducers, transcription factors, apoptosis regulators, and cell cycle regulators (see table on page 22). Defects in any of these can provide a “gain of function” and cause a cell to grow out of control. For example, GFs serve as ligands that bind to receptors on the surface of cells. If a GF, or GFR, is overproduced by an oncogene, the target cells may experience uncontrolled proliferation (Carpenter & Cantley, 2005; Connolly et al., 2006; Smith, Marks, & Lieberman, 2005). Other oncogenes can affect signal transduction in cells and activate a series of protein kinases, leading to uncontrolled cell proliferation. However, the mutation only occurs in one copy of a proto-oncogene.
Oncogene | Function | Malignancy |
---|---|---|
abl | Tyrosine kinase activity | Chronic myelogenous leukemia |
akt-2 | Cell cycle progression | Ovarian and pancreatic cancer |
c-myc | DNA synthesis | Breast, cervical, colorectal, and lung cancer; leukemia, neuroblastoma, glioblastoma |
egfr | Tyrosine kinase | Squamous cell carcinoma |
erbB | Tyrosine kinase | Brain tumors; squamous cell carcinoma |
erbB-2 (formerly neu) | Tyrosine kinase | Breast and ovarian cancer, cancer of the salivary gland |
ets-1 | Transcription factor | Lymphoma |
fos | Transcription factor | Osteosarcoma |
HER-2/neu | Fusion gene | Breast and cervical cancer |
hst | Growth factor | Breast cancer; squamous cell carcinoma |
int-2 | Growth factor | Breast cancer; squamous cell carcinoma |
jun | Transcription factor | Sarcoma |
kit | Tyrosine kinase | Sarcoma |
L-myc | DNA synthesis | Lung cancer |
mdm-2 | p53 inhibitor | Sarcoma |
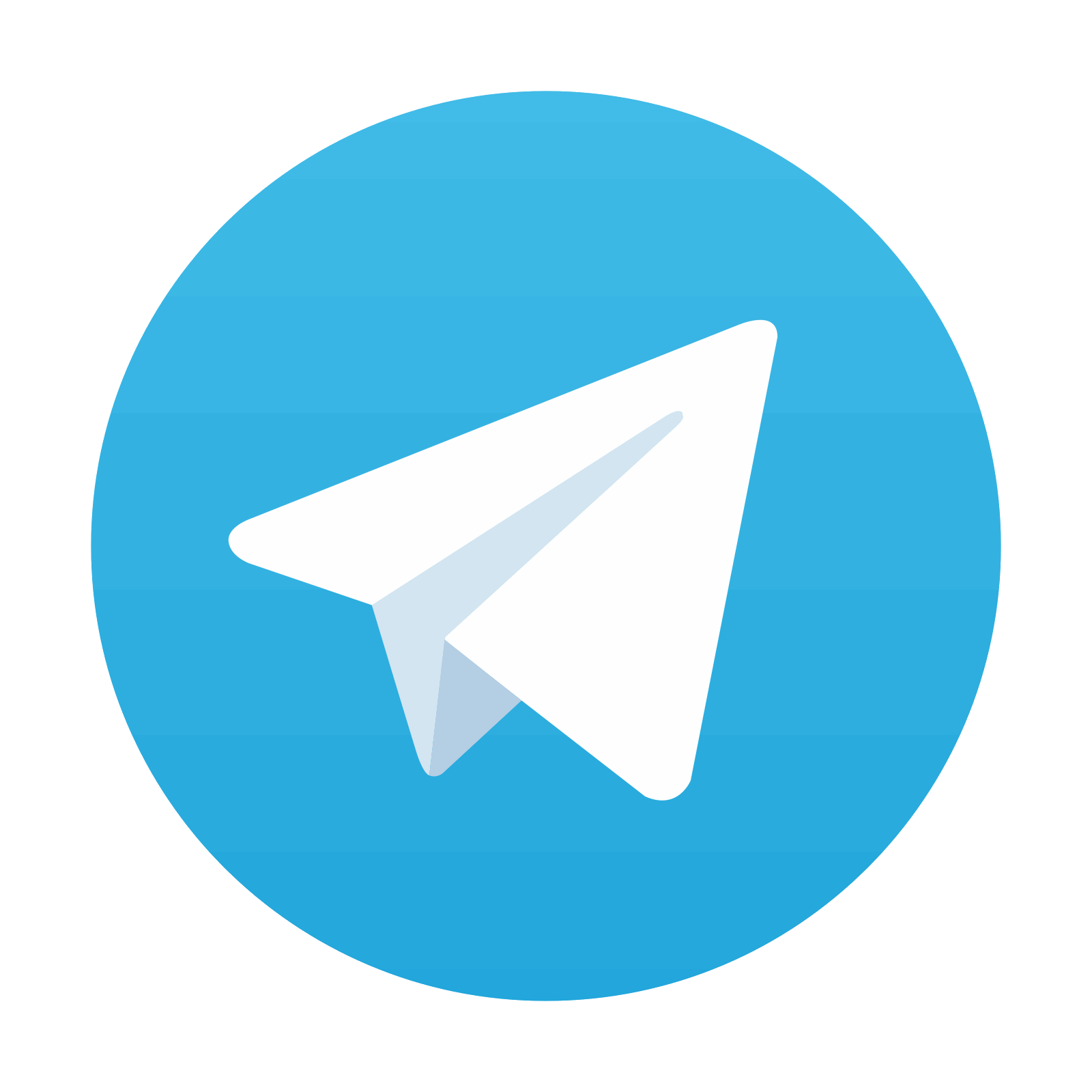
Stay updated, free articles. Join our Telegram channel

Full access? Get Clinical Tree
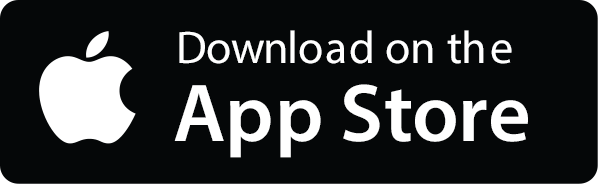
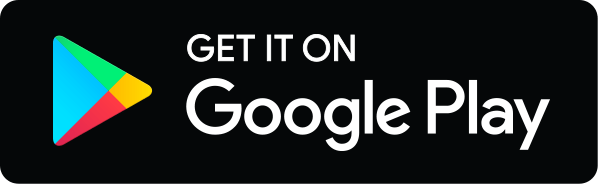