Biological Response Modifiers
HISTORICAL OVERVIEW OF BIOLOGICAL RESPONSE AND IMMUNOLOGY
From a historical viewpoint, the study and understanding of immunology and the subsequent response of human biology succeeding a noxious event to prevent future insult have been understood for far longer than the knowledge of germ theory and of mutation and carcinogenesis (Bellanti, 1985). Dating as far back as the eleventh century, it was understood that after exposure to a virus such as smallpox, resistance to further infection would develop. The earliest active use of immunization was performed by Lady Mary Wortley Montague, who in 1721 inoculated her daughter against smallpox. (DeVita, Hellman, & Rosenberg, 1991). Early attempts at vaccination against toxins often resulted in infection and death leading to a lack of widespread belief in the technique. Later, work near the turn of the nineteenth century by Edward Jenner pioneered further work and the advancement of smallpox vaccination. Additional work done by Louis Pasteur led to a better understanding of the role and function of vaccine therapy, immunity, and the “germ theory” (Bellanti, 1985). Pasteur’s work eventually led to further work in the immunology field and a better understanding of disease biology and the responses to disease that occur after an invading foreign antigen has entered the system (Bellanti, 1985).
1. Humoral immunity: chemical products (antibodies) produced and released by cells that destroy the specific invading toxin (antigen)
2. Cellular immunity: immunoglobins, or effector cells, detect and destroy foreign toxins (Bellanti, 1985)
As a better understanding of immunology grew, theories and hypotheses as to the potential role for activation of the immune system may be beneficial in antitumor therapies. Some of the earliest of these hypotheses dates back to Paul Ehrlich and his postulations that tumors may express different cell structures and thus be recognizable to antibodies and cause increased humoral immune response (DeVita, Hellman, & Rosenberg, 1991). Unfortunately, early work in the field of tumor immunity was thwarted by the realization that antitumor activity demonstrated in mice was the result of an allogeneic rejection response to foreign “non-self” tissue as opposed to a direct antitumor immunity. This problem was later overcome by the development of various rodent models that allowed for implantation and culturing of tumor grafts without allogeneic rejection (DeVita, Hellman, & Rosenberg, 1991). These mouse and rat models are used as a staple in antitumor drug development and therapy research and as a first step in the preclinical approach of understanding a molecule’s potential for further study.
By the end of the 1960s, the rodent models had shown that there were direct antitumor responses being seen in the study of these models. As such, the first human studies were undertaken to determine whether these results could be verified and replicated. The cornerstone for further investigation into biological response modification came from studies reported in 1966 by Klein and colleagues, who demonstrated that circulating antibodies associated with antigens were located on the cell surface of Burkitt’s lymphoma cells (DeVita, Hellman, & Rosenberg, 1991). These results demonstrated the existence of specific tumor type–specific antigens that can potentially be targeted by the immune system for the treatment of various cancer types.
Until the 1970s the study of antibodies excreted by T lymphocytes to cause a direct tumor-specific antigen/antibody reaction for the treatment of cancer monopolized the majority of the focus and study of biological response modification. However, during this time, further understanding of the ability of certain lymphocytes to secrete other small proteins that had a direct impact on biological response and immune modulation began to be better understood (DeVita, Hellman, & Rosenberg, 2001a). This new class of proteins was termed cytokines. Cytokines are soluble glycoproteins that are typically secreted by lymphocytes and monocytes and are true hormones acting on cells in a paracrine or autocrine fashion to activate or deactivate downstream cellular function, activity, or division, among many other roles (DeVita, Hellman, & Rosenberg, 2001a).
COLONY-STIMULATING FACTORS
Colony-stimulating factors comprise a family of glycoproteins that help to regulate differentiation, proliferation, and activation of cells of the hematopoietic cell lineages. Various cytokines act on different cells of the cascade at different times during the differentiation and proliferation phases of their individual cell lives. These interactions can be paracrine or autocrine in nature and can have activity that is either direct or indirect through the activation and production of downstream ligands and cytokines.
The first of the colony-stimulating factors to be discovered was erythropoietin in 1906 by Carnot and Deflandre (Metcalf & Nicola, 1995). Further purification of erythropoietin was not accomplished until 1976 by Miyake et al. Since the early 1960s, continued advancement in the bioassay field has lead to the discovery and description of some 20 additional cytokines with activity on hematopoietic cells. Initially, tissue cultured hematopoietic cells were used as bioassay platforms from which the cytokines detected would later be tested on animal models to determine target activity and utility. Today, complementary deoxyribonucleic acid (cDNA) screening is used for the detection and development of cytokines, which allows for characterization of the active molecules and determination of the receptor sites on normal cells (Metcalf & Nicola, 1995).
The term colony-stimulating factor was coined subsequent to work done in 1966 by Bradley and Metcalf and Ichikawa et al studying macrophage and granulocyte cells cultured from bone marrow. In these trials, spontaneous growth was not observed. However, when additional tissues were added to the cultures, proliferation was demonstrated, leading to the hypothesis that there were factors stimulating an increase in proliferation of the granulocytes and macrophages similar to the effect of erythropoietin on erythrocytes demonstrated years earlier (Metcalf & Nicola, 1995).
There are, at present, four colony-stimulating factors approved for use in the treatment of cancer by the U.S. Food and Drug Administration. These are (DeVita, Hellman, & Rosenberg, 2001b) as follows:
• Granulocyte colony-stimulating factor (G-CSF)
• Granulocyte-macrophage colony-stimulating factor (GM-CSF)
Erythropoietins
Erythropoietin production and secretion is inversely affected by the oxygen-carrying capacity of the circulating red blood cells (DeVita, Hellman, & Rosenberg, 2001b). This negative feedback loop decreases secretion of EPO in times of high levels of oxygen-binding capacity and increases EPO secretion in times of lower oxygen-binding capacity, allowing for balance in the amount of circulating red blood cells to match the need of the body’s oxygen demand without overproduction of red blood cells. In some instances overproduction of erythropoietin resulting in erythrocytosis is observed in patients noted to have primary renal tumors causing local renal hypoxic conditions or tumors that oversecrete erythropoietin intrinsically (DeVita, Hellman, & Rosenberg, 2001b).
EPO is a useful and commonly used therapeutic agent in the treatment of chemotherapy-associated anemia. Normal human serum concentrations of EPO range from 4 to 30 units/L. Recombinant EPOs have a half life of 9 to 13 hours in regard to epoetin alfa and 27 to 89 hours in regard to darbepoetin alfa (Wilkes & Barton-Burke, 2005). In healthy human subjects, EPO levels demonstrably begin to rise when hematocrit levels fall below 35%; however, in patients with chronic anemia from malignancies, serum EPO levels may remain low (DeVita, Hellman, & Rosenberg, 2001b). As a result, the administration of recombinant EPO in this instance can have a positive effect on raising the percentage of circulating erythrocytes and a subsequent impact on oxygen saturation and a qualitative impact on fatigue levels experienced by patients.
The U.S. Food and Drug Administration in 1999 approved recombinant EPO for the treatment of anemia in cancer patients after several studies using multiple dosing schemes demonstrated statistically significant decreases in necessary red blood cell transfusions among patients who received epoetin alfa (14%) versus those who received placebo (28%) between day 29 and week 16 of therapy. The effects of EPO therapy are typically not seen until between 2 and 6 weeks of therapy and measured by an increase in the hemoglobin level. Continued monitoring of serum hemoglobin levels is necessary to prevent unintended erythrocytosis (Amgen, 2004 Procrit).
Granulocyte Colony-Stimulating Factor
G-CSF is a colony-stimulating factor that regulates cell proliferation, maturation, and function in the neutrophil cell lineage of the myeloid cell line. After the human gene for G-CSF located on chromosome 17q11-21 was identified through purification of bladder and head and neck cancer cells, the gene was cloned and recombinant technology used to produce usable amounts of drug. Although G-CSF may have downstream effects on other cell lineages and the potential function of those cells under certain circumstances, its primary function is the proliferation and maturation of the neutrophil cell lineage. This becomes apparent in the setting of mice with a knockout gene for G-CSF, where the mice become severely neutropenic. Hammond et al also demonstrated the selectivity of G-CSF for neutrophils in animal models, as reflected by severe neutropenic states caused by the induction of neutralizing antiantibodies for G-CSF in those models (DeVita, Hellman, & Rosenberg, 1991; DeVita, Hellman, & Rosenberg, 2001b).
G-CSF can be secreted by a variety of cells in the body including the following (DeVita, Hellman, & Rosenberg 2001b):
G-CSF can be readily detected in circulating serum at levels of 20 to 100 mg/dL. Circulating G-CSF levels and secretion are directly affected by inflammatory response to toxins and tissue damage and are inversely affected by the total number of circulating neutrophils. In severely neutropenic subjects, detectable levels of serum G-CSF can be in excess of 2,000 mg/dL (DeVita, Hellman, & Rosenberg, 2001b).
There are two main G-CSFs in use in the United States today:
Filgrastim is the parent molecule of this class of cytokine. Randomized clinical trials demonstrate that the use of filgrastim results in the following (Amgen, Neupogen 2004):
• A reduction in occurrence of febrile neutropenia
• A significant decrease in hospital length of stay for infection
• A decrease in overall use of antibiotics
• A reduction in the absolute neutrophil count recovery time for patients with acute myeloid leukemia (AML) undergoing induction or consolidation therapy
• A reduction in the median number of days with severe neutropenia in patients receiving myeloablative chemotherapy for bone marrow transplant
• Significant elevation of colony-forming units of granulocytes and macrophages and CD34+ cells in the setting of stem cell collection and mobilization. An increase in these cells is a predictor of engraftment and time to platelet recovery after transplantation.
The pegylated formulation of filgrastim, pegfilgrastim, is a covalent conjugate of filgrastim in which a glycol molecule has been bound to filgrastim and as such results in reduced renal clearance and a resultant prolonged persistence in vivo. This molecular change results in an increase in average half-life to 15 to 80 hours with pegfilgrastim, allowing for single-dose administration after each course of chemotherapy associated with a significant risk of febrile neutropenia. (Amgen, Pegfilgrastim 2005).
Pegfilgrastim has been demonstrated through three phase III clinical trials to produce equivalent reductions in days of severe neutropenia compared with filgrastim in patients receiving chemotherapy associated with a high risk of neutropenia (Amgen, Pegfilgrastim 2005). Because of its prolonged half-life, pegfilgrastim should not be used within the 14 days leading up to the administration of chemotherapy.
Granulocyte-Macrophage Colony-Stimulating Factor
First cloned by Wong and colleagues in 1985, GM-CSF, located on chromosome 5q31, is another potent cytokine that has a direct impact on hematopoiesis as well as many other implications for the care of patients with cancer (DeVita, Hellman, & Rosenberg, 2001b). GM-CSF is excreted by a number of cell lines including the following:
The most prolific production of GM-CSF naturally occurring in the human body is that secreted by activated T lymphocytes (DeVita, Hellman, & Rosenberg, 2001b).
GM-CSF affects a diverse population of cell lineages in the hematopoietic cascade, including all myeloid cell lines, and affects myeloid dendritic cell activation proliferation and differentiation. As a result, GM-CSF increased circulating numbers of neutrophils, eosinophils, and macrophages (DeVita, Hellman, & Rosenberg, 2001b).
Pharmacokinetic studies of GM-CSF have shown a significant increase in sustained circulating levels of GM-CSF in the serum when administered by subcutaneous injection versus intravenous administration. As such, subcutaneous administration is the preferred route (DeVita, Hellman, & Rosenberg, 2001b).
GM-CSF has been and continues to be aggressively studied in the care of malignancies. It has been approved clinically for use after induction chemotherapy in AML, mobilization and after autologous peripheral blood progenitor cells, myeloid reconstitution after autologous bone marrow transplantation (BMT), myeloid reconstitution after allogeneic BMT, and in the setting of bone marrow transplant failure and engraftment delay (Berlex, 2004).
The rationale for use of GM-CSF in the treatment of mucositis comes in part by the ability of GM-CSF to activate keratinocytes and healthy well-functioning neutrophils in the epithelial lining of the gastric mucosa, including the oral pharynx. This increase in cell activation promotes healthy cell turnover and population-decreasing mucosal toxicity. A study conducted by McAleese and colleagues with a population of 29 participants demonstrated a significant reduction in radiation-related mucositis in patients being treated for laryngeal cancer with radiation therapy along with molgrastim (McAleese et al., 2006). Several other trials looking at the potential utility and benefit of cytokines and colony-stimulating factors, including GM-CSF, have been performed, demonstrating varying results (Bülttzingslöwen et al., 2006). This area needs further investigation and continues to be evaluated for optimal drug, dose, administration, and timing.
GM-CSF is a main component in the study of gene- and vaccine-based therapy for the treatment of malignancies. GM-CSF has been shown to enhance antibody response in favor of TH1, which is associated with myeloid dendritic cells and antitumor effect (Oppenheim & Fujiwara, 1996). Several clinical trials are in process to better determine the efficacy and utility of dendritic cell–based vaccine therapies. In the majority of these trials, GM-CSF is used to activate, culture, and mature naive dendritic cells for use as potent effector cells preloaded with specific tumor antibodies to elicit T-lymphocyte activation and downstream biological responses against those tumors positive for the antigen (Oppenheim & Fujiwara, 1996; Wang et al., 2006).
Interleukin-11 (oprelvekin, Neumega)
IL-11 is a cytokine/growth factor produced by several mesenchymal cell families that has a direct effect on hematopoiesis. Cells that produce IL-11 naturally include keratinocytes, osteoblasts, fibroblasts, and bone marrow stromal cells. (DeVita, Hellman, & Rosenberg, 2001b). The predominant function of IL-11 is its direct impact on megakaryocopoiesis and thrombopoiesis and the proliferation, maturation, and function of those cell lines. (DeVita, Hellman, & Rosenberg, 2001b; Wyeth Pharmaceuticals, 2006). As a result, there is a direct impact on thrombocytopenia as a result of the chemotherapeutic insult. IL-11 is clinically approved for the prevention of severe thrombocytopenia after myelosuppressive chemotherapy in patients with nonmyeloid malignancies and patients receiving nonmyeloablative chemotherapy regimens (Brown et al., 2001). IL-11 is expressed on gene 19q13-4-13.4 of the human genome and was first cloned by Yang and colleagues at the Genetics Institute at Case Western Reserve in the early 1990s.
In clinical studies, IL-11 has been shown to improve platelet nadir and decrease the time to platelet recovery in patients receiving chemotherapeutic regimens. Use of oprelvekin results in a decreased need for platelet transfusions (Wyeth Pharmaceuticals, 2006).
The use of IL-11 is strictly contraindicated in patients receiving myeloablative chemotherapy because of a significantly elevated occurrence of edema, conjuctival bleeding, hypotension, and tachycardia seen in this patient population. Administration of IL-11 is also associated with significant risk of allergic reaction, including anaphylaxis, and should be discontinued immediately at the first sign of a drug-related allergic response (Wyeth Pharmaceuticals, 2006).
IL-11 therapy should begin within 6 to 24 hours after chemotherapy completion. However, safety and efficacy of IL-11 has not been determined in patients undergoing chemotherapy administration associated with prolonged or delayed myelosuppression, as is the case with mitomycin-c and nitrosourea. (Wyeth Pharmaceuticals, 2006).
Keratinocyte Growth Factor (palifermin, Kepivance)
Keratinocyte growth factor (KGF), also known as fibroblast growth factor-7, is a growth factor originating from mesenchymal cells, fibroblasts, and microvascular endothelial cells. It acts in a paracrine fashion activating epithelial cell repair and protection as a response to inflammatory cytokines and steroidal hormones (Finch & Rubin, 2006; MacDonald & Hill, 2002). In the study of KGF in myeloid malignancies, early evidence has suggested that its addition to chemotherapy can prevent gastrointestinal graft-versus-host disease by acting as a kind of cytokine protectant and reducing the generation of proinflammatory cytokines (MacDonald & Hill, 2002). In addition, KGF has been shown to help promote T-cell engraftment and reconstitution, leading to a belief that KGF may play an important role in the prevention of epithelial toxicity in the treatment of myeloid malignancies (MacDonald & Hill, 2002).
Keeping the direct effect on epithelial tissues in mind, studies have begun to look at the protective properties of KGF in the setting of chemotherapy- and radiation-induced mucositis. In patients with hematological malignancies, trials have demonstrated a direct correlation between KGF administration and a reduction in the severity and duration of oral mucositis as a result of intense chemotherapy regimens. (Finch & Rubin, 2006). In vitro and in vivo studies have shown KGF to be an important cytoprotectant through its ability to positively affect epithelial cell differentiation, proliferation, and migration as well as having beneficial effects on epithelial cell survival, repair, and detoxification after exposure to cytotoxins (Finch & Rubin, 2006). Results of a phase III trial of transplant patients with hematological malignancies receiving high-dose radiation and chemotherapy before transplantation showed a significant reduction in the incidence and duration of severe oral mucositis (P < 0.001) in the arm that received drugs. This has led to U.S. Food and Drug Administration approval for use of KGF in this setting.
Trials looking at the potential benefit of KGF in epithelial solid tumors are currently in progress or planned, including RTOG 0435 comparing KGF with placebo in patients with head and neck cancer undergoing chemoradiation therapy for the prevention of mucositis. A concern that has arisen with the study of KGF in epithelial malignancies is that, given the protective nature of this protein in the chemotherapy setting toward epithelial tissues, will the resultant protection cross over to the malignant cells themselves and shield them from the wanted effects of the therapies being used (Finch & Rubin, 2006; MacDonald & Hill, 2002; Oelmann et al., 2004)? Further studies are needed to determine the answer.
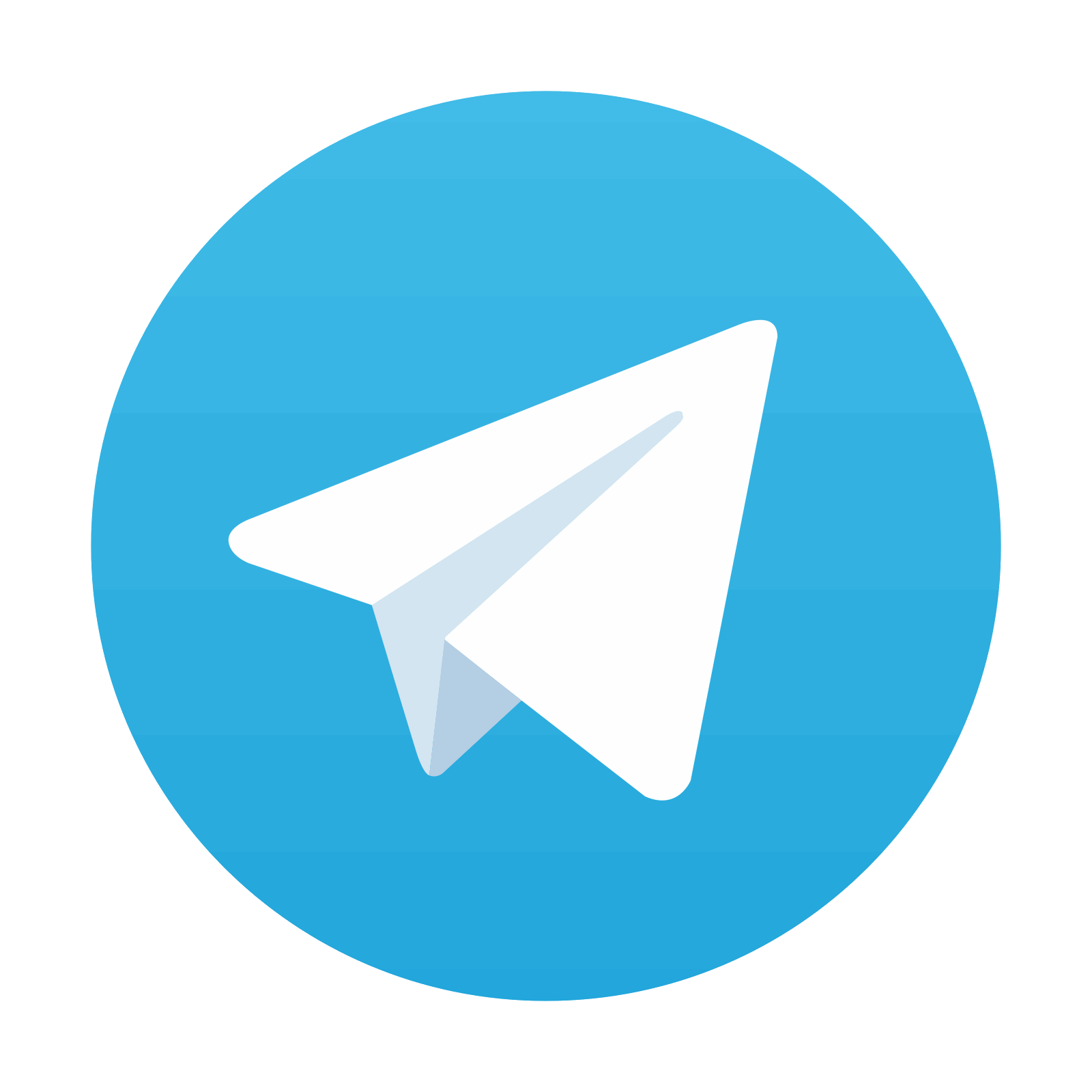
Stay updated, free articles. Join our Telegram channel

Full access? Get Clinical Tree
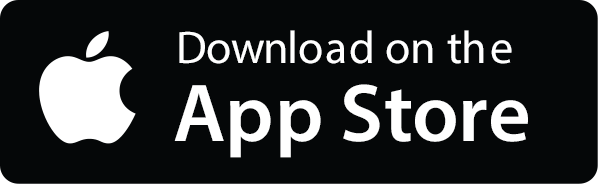
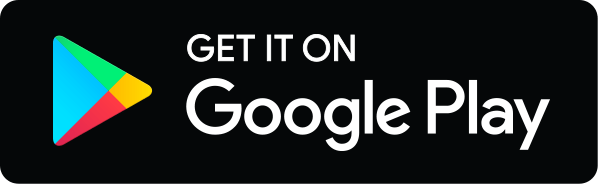