Antibiotics Part 1
Objectives
When you reach the end of this chapter, you will be able to do the following:
1 Discuss the general principles of antibiotic therapy.
2 Explain how antibiotics work to rid the body of infection.
3 Briefly compare the characteristics and uses of antiseptics and disinfectants.
4 List the most commonly used antiseptics and disinfectants.
10 Briefly discuss superinfection, including its etiology and prevention.
Drug Profiles
♦ azithromycin and clarithromycin, p. 625
♦ penicillin G and penicillin V potassium, p. 618
sulfamethoxazole/trimethoprim (co-trimoxazole), p. 616
♦ Key drug
Key Terms
Antibiotic Having the ability to destroy or interfere with the development of a living organism. The term is used most commonly to refer to antibacterial drugs. (p. 611)
Antiseptic One of two types of topical antimicrobial agents; a chemical that inhibits the growth and reproduction of microorganisms without necessarily killing them. Antiseptics are also called static agents. (p. 612)
Bactericidal antibiotics Antibiotics that kill bacteria. (p. 616)
Bacteriostatic antibiotics Antibiotics that do not actually kill bacteria but rather inhibit their growth. (p. 614)
Beta-lactam The designation for a broad class of antibiotics that includes four subclasses: penicillins, cephalosporins, carbapenems, and monobactams; so named because of the beta-lactam ring that is part of the chemical structure of all drugs in this class. (p. 616)
Beta-lactamase Any of a group of enzymes produced by bacteria that catalyze the chemical opening of the crucial beta-lactam ring structures in beta-lactam antibiotics. (p. 616)
Beta-lactamase inhibitors Medications combined with certain penicillin drugs to block the effect of beta-lactamase enzymes. (p. 616)
Colonization The establishment and growth of microorganisms on the skin, open wounds, or mucous membranes, or in secretions without causing an infection. (p. 611)
Community-associated infection An infection that is acquired by persons who have not been hospitalized or had a medical procedure recently. (p. 611)
Definitive therapy The administration of antibiotics based on known results of culture and sensitivity testing identifying the pathogen causing infection. (p. 612)
Disinfectant One of two types of topical antimicrobial agents; a chemical applied to nonliving objects to kill microorganisms. Also called cidal agents. (p. 612)
Empiric therapy The administration of antibiotics based on the practitioner’s judgment of the pathogens most likely to be causing an apparent infection; it involves the presumptive treatment of an infection to avoid treatment delay before specific culture information has been obtained. (p. 612)
Glucose-6-phosphate dehydrogenase (G6PD) deficiency An inherited disorder in which the red blood cells are partially or completely deficient in glucose-6-phosphate dehydrogenase, a critical enzyme in the metabolism of glucose. Certain medications can cause hemolytic anemia in patients with this disorder. This is an example of a host factor related to drug therapy. (p. 614)
Health care–associated infection An infection that is acquired during the course of receiving treatment for another condition in a health care facility. The infection is not present or incubating at the time of admission; also known as a nosocomial infection. (p. 611)
Host factors Factors that are unique to a particular patient that affect the patient’s susceptibility to infection and response to various antibiotic drugs. Examples include a low neutrophil count or a lack of immunoglobulins in the blood that carry antibodies. (p. 613)
Infections Invasions and multiplications of microorganisms in body tissues. (p. 611)
Microorganisms Microscopic living organisms (also called microbes). (p. 610)
Prophylactic antibiotic therapy Antibiotics taken before anticipated exposure to an infectious organism in an effort to prevent the development of infection. (p. 612)
Pseudomembranous colitis A potentially-necrotizing inflammatory bowel condition that is often associated with antibiotic therapy; often caused by the bacteria Clostridium difficile. A more general term that is also used is antibiotic-associated colitis. (p. 613)
Slow acetylation A common genetic host factor in which the rate of metabolism of certain drugs is reduced. (p. 614)
Subtherapeutic Generally refers to blood levels below therapeutic levels due to insufficient dosing. Also refers to antibiotic treatment that is ineffective in treating a given infection. Possible causes include inappropriate drug therapy, insufficient drug dosing, and bacterial drug resistance. (p. 612)
Superinfection (1) An infection occurring during antimicrobial treatment for another infection, resulting from overgrowth of an organism not susceptible to the antibiotic used. (2) A secondary microbial infection that occurs in addition to an earlier primary infection, often due to weakening of the patient’s immune system function by the first infection. (p. 613)
Teratogens Substances that can interfere with normal prenatal development and cause one or more developmental abnormalities in the fetus. (p. 614)
Therapeutic Referring to antibiotic therapy that is given in sufficient doses so that the concentration of the drug in the blood or other tissues renders it effective against specific bacterial pathogens. (p. 612)
http://evolve.elsevier.com/Lilley
• Answer Key—Textbook Case Studies
• Critical Thinking and Prioritization Questions
• Review Questions for the NCLEX® Examination
Anatomy, Physiology, and Pathophysiology Overview
Microbial Infection
Microorganisms are everywhere, both in the external environment and in parts of the internal environment of our bodies. They can be harmful to humans, or they can be beneficial under normal circumstances but become harmful when conditions are altered in some way. A person is normally able to remain healthy and resistant to infectious microorganisms because of the existence of certain host defenses. These defenses include actual physical barriers, such as intact skin or the ciliated respiratory mucosa, or physiologic defenses, such as the gastric acid in the stomach and immune factors such as antibodies. Other defenses are the phagocytic cells (macrophages and polymorphonuclear neutrophils) that are part of the mononuclear phagocyte system.
Every known major class of microbes contains organisms that can infect humans. This includes bacteria, viruses, fungi, and protozoans. The focus of this chapter is common bacterial infections.
Recall from microbiology that bacteria may take a number of different shapes. This property of bacteria is called their morphology (Figure 38-1), and they are often grouped based on this property. Bacteria may also be grouped according to other common recognizable characteristics. One of the most important ways of categorizing different bacteria is on the basis of their response to the Gram stain procedure. Bacterial species that stain purple with Gram staining are classified as gram-positive organisms. Bacteria that stain red are classified as gram-negative organisms. This seemingly simple difference proves to be very significant in guiding the choice of antibiotic therapy.
Gram-positive organisms have a very thick cell wall, known as peptidoglycan, and they also have a thick outer capsule. Gram-negative organisms have a cell wall structure that is more complex, with a smaller outer capsule and peptidoglycan layer and two cell membranes: an outer and an inner membrane (Figure 38-2). These differences usually make gram-negative bacterial infections more difficult to treat, because the drug molecules have a harder time penetrating the more complex cell walls of gram-negative organisms.
When the normal host defenses are somehow compromised, that person becomes susceptible to infection. The microorganisms invade and multiply in the body tissues, and if the infective process overwhelms the body’s defense system, the infection becomes clinically apparent. The patient usually manifests some of the following classic signs and symptoms of infection: fever, chills, sweats, redness, pain and swelling, fatigue, weight loss, increased white blood cell (WBC) count, and the formation of pus. Not all patients will exhibit signs of the infection. This is especially true in elderly and immunocompromised patients.
To help the body and its normal host defenses combat an infection, antibiotic therapy is often required. Antibiotics are most effective when their actions are combined with functioning bodily defense mechanisms. Oftentimes patients will become colonized with bacteria. Although bacteria are present in open wounds, in secretions, on mucous membranes, or on the skin, these patients do not have any overt signs of infection. Colonization does not require antibiotic treatment. However, it is not uncommon for these colonizations to be treated, which may be one way in which drug-resistant organisms emerge.
Health Care–Associated Infection
A community-associated infection is defined as an infection that is acquired by a person who has not recently (within the past year) been hospitalized or had a medical procedure (e.g., dialysis, surgery, catheterization). A health care–associated infection, previously known as a nosocomial infection, is defined as an infection that a patient acquires during the course of receiving treatment for another condition in a health care facility. The infection was not present or incubating at the time of admission but occurs greater than 48 hours after admission. Health care–associated infections are one of the top ten leading causes of death in the United States. They tend to be more difficult to treat because the causative microorganisms have been exposed to strong antibiotics in the past and are the most drug resistant and the most virulent. The particular organisms that cause hospital-associated infections have changed over time, with methicillin-resistant Staphylococcus aureus (MRSA) now being the most common. Other serious pathogens include Enterococcus, Klebsiella, Acinetobacter, and Pseudomonas aeruginosa. Most of these microorganisms are now resistant to many of the commonly used antibiotics.
Health care–associated infections develop in approximately 10% of hospitalized patients, and the cost of treating these infections amounts to $4 to $11 billion annually. Most of these infections (70% or more) are either urinary tract infections (UTIs) or postoperative wound infections. Often they are acquired from various devices, such as mechanical ventilators, intravenous (IV) infusion lines, catheters, and dialysis equipment. Areas of the hospital associated with the greatest risk for acquiring a hospital-associated infection are the critical care, dialysis, oncology, transplant, and burn units. This is because the host defenses of the patients in these areas are typically compromised, which makes them more vulnerable to infection. Over 70% of hospital-associated infections are preventable. The most common mode of transmitting hospital-associated infections is by direct contact. Handwashing is the single most important thing health care professionals can do to prevent the spread of these potentially deadly infections. Because health care–associated infections cause numerous deaths and cost billions of dollars, The Joint Commission has made the prevention of health care associated–infections a national patient safety goal. In addition, they have added prevention of catheter-associated UTIs as a 2012 national patient safety goal.
Other methods of reducing hospital-associated infections include the use of disinfectants and antiseptics. A disinfectant is able to kill organisms and is used only on nonliving objects to destroy organisms that may be present. Disinfectants are sometimes called cidal agents. An antiseptic generally only inhibits the growth of microorganisms but does not necessarily kill them and is applied exclusively to living tissue. Antiseptics are also called static agents. The differences between antiseptics and disinfectants in a clinical sense are summarized in Table 38-1. Topical antimicrobial drugs are discussed further in Chapter 56.
TABLE 38-1
ANTISEPTICS VERSUS DISINFECTANTS
ANTISEPTICS | DISINFECTANTS | |
Where used | Living tissue | Nonliving objects |
Potency | Lower | Higher |
Activity against organisms | Primarily inhibits growth (bacteriostatic) | Kills (bactericidal) |
Pharmacology Overview
The selection of antimicrobial drugs requires clinical judgment and detailed knowledge of pharmacologic and microbiologic factors. Antibiotics have three general uses: empiric therapy, definitive therapy, and prophylactic or preventative therapy. Antibiotic drug therapy begins with a clinical assessment of the patient to determine whether he or she has the common signs and symptoms of infection. The patient is assessed during and after antibiotic therapy to evaluate the effectiveness of the drug therapy, monitor for adverse drug effects, and make sure the infection is not recurring.
Often the signs and symptoms of an infection appear long before a causative organism can be identified. When this happens and the risk of life-threatening or severe complications is high (e.g., suspected acute meningitis), an antibiotic is given to the patient immediately. The antibiotic selected is one that can best kill the microorganisms known to be the most common causes of the infection. This is called empiric therapy. Before the start of empiric antibiotic therapy, specimens are obtained from suspected areas of infection to be cultured in an attempt to identify a causative organism. It must be emphasized that culture specimens must be obtained before drug therapy is initiated whenever possible. Otherwise, the presence of antibiotics in the tissues may result in misleading culture results. However, sometimes it is not possible to obtain a sample (especially sputum) in a reasonable amount of time, and antibiotic therapy is begun without a sample in that situation. If an organism is identified in the laboratory, it is then tested for susceptibility to various antibiotics. The results of these tests can confirm whether the empiric therapy chosen is appropriate for eradicating the organism identified. If not, therapy can be adjusted to optimize its efficacy against the specific infectious organism(s). Once the results of culture and sensitivity testing are available (usually in 48 to 72 hours), the antibiotic therapy is then tailored to treat the identified organism by using the most narrow-spectrum, least toxic drug based on sensitivity results. This is known as definitive therapy. Broad-spectrum antibiotics are those that are active against numerous organisms (gram-positive, gram-negative, and anaerobic). Narrow-spectrum antibiotics are effective against only a few organisms. Once the results of culture and sensitivity testing are available, it is always better to use an antibiotic that targets the specific organism identified (i.e., a narrow-spectrum antibiotic). Overuse of broad-spectrum antibiotics contributes to resistance. The goal of therapy is to use the most narrow-spectrum drug when possible based on sensitivity results.
Antibiotics are also given for prophylaxis. This is often the case when patients are scheduled to undergo a procedure (i.e., surgery) in which the likelihood of dangerous microbial contamination is high during or after the procedure. Prophylactic antibiotic therapy is used to prevent an infection. The risk of infection varies depending on the procedure being performed. For example, the risk of infection in a patient undergoing coronary artery bypass surgery (with standard preoperative cleansing of the body) is relatively low compared with that in a person undergoing intraabdominal surgery for the treatment of injuries sustained in a motor vehicle accident. In the latter case, contamination with bacteria from the gastrointestinal (GI) tract is more likely to be present in the abdominal cavity. This would constitute a contaminated or “dirty” surgical field, and therefore the likelihood of clinically serious infection would be much higher. Antibiotic therapy would likely be required for a longer period after the procedure. To be effective, prophylactic antibiotics need to be given before the procedure, generally 30 minutes before the incision to ensure adequate tissue penetration. The Surgical Care Improvement Project (SCIP) is a national performance improvement project that provides hospitals with evidence-based recommendations on the administration of prophylactic antibiotics. More information can be found at www.jointcommission.org/surgical_care_improvement_project/.
To optimize antibiotic therapy, the patient is continuously monitored for both therapeutic efficacy and adverse drug effects. A therapeutic response to antibiotics is one in which there is a decrease in the specific signs and symptoms of infection compared with the baseline findings (e.g., fever, elevated WBC count, redness, inflammation, drainage, pain). Antibiotic therapy is said to be subtherapeutic when these signs and symptoms do not improve. This can result from use of an incorrect route of drug administration, inadequate drainage of an abscess, poor drug penetration to the infected area, insufficient serum levels of the drug, or bacterial resistance to the drug. Antibiotic therapy is considered toxic when the serum levels of the antibiotic are too high or when the patient has an allergic or other major adverse reaction to the drug. These reactions include rash, itching, hives, fever, chills, joint pain, difficulty breathing, or wheezing. Relatively minor adverse drug reactions such as nausea, vomiting, and diarrhea are quite common with antibiotic therapy and are usually not severe enough to require drug discontinuation.
Superinfection can occur when antibiotics reduce or completely eliminate the normal bacterial flora, which consist of certain bacteria and fungi that are needed to maintain normal function in various organs. When these bacteria or fungi are killed by antibiotics, other bacteria or fungi are permitted to take over and cause infection. An example of a superinfection caused by antibiotics is the development of a vaginal yeast infection when the normal vaginal bacterial flora is reduced by antibiotic therapy and yeast growth is no longer kept in balance. Antibiotic use is strongly associated with the potential for the development of diarrhea. Antibiotic-associated diarrhea is a common adverse effect of antibiotics. However, it becomes a serious superinfection when it causes antibiotic-associated colitis, also known as pseudomembranous colitis or simply C. difficile infection. This happens because antibiotics disrupt the normal gut flora and can cause an overgrowth of Clostridium difficile. The most common symptoms of C. difficile colitis are watery diarrhea, abdominal pain, and fever. Whenever a patient who was previously treated with antibiotics develops watery diarrhea, the patient needs to be tested for C. difficile infection. If the results are positive, the patient will need to be treated for this serious superinfection. Infections with C. difficile are increasingly becoming resistant to standard therapy.
Another type of superinfection occurs when a second infection closely follows the initial infection and comes from an external source (as opposed to normal body flora). A common example is a case in which a patient who has a viral respiratory infection develops a secondary bacterial infection. This is likely due to weakening of the patient’s immune system function by the primary viral infection. Although the viral infection will not respond to antibiotic therapy, antibiotics may be needed to treat the secondary bacterial infection. This situation calls for some diagnostic finesse on the part of the prescriber, who needs to avoid prescribing unnecessary antibiotics for a viral infection. The presence of colored sputum (e.g., green or yellow) may or may not be a sign of a bacterial superinfection during a viral respiratory illness. Patients will often expect to receive an antibiotic prescription even when they show no signs of a bacterial superinfection. From their perspective, they know they are “sick” and want “some medicine” to expedite their recovery from illness. This can create both diagnostic confusion and an emotional dilemma for the prescriber.
Over the decades, many easily treatable bacterial infections have become resistant to antibiotic therapy. One major cause of this phenomenon is considered to be the overprescribing of antibiotics, often in the clinical situations described earlier. Antibiotic resistance is now considered one of the world’s most pressing public health problems. Another factor that contributes to this problem is the tendency of many patients not to complete their antibiotic regimen. Patients must be counseled to take the entire course of prescribed antibiotic drugs, even if they feel that they are no longer ill.
Food-drug and drug-drug interactions are common problems when antibiotics are taken. One of the more common food-drug interactions is that between milk or cheese and tetracycline, which results in decreased GI absorption of tetracycline. An example of a drug-drug interaction is that between quinolone antibiotics and antacids or multivitamins with iron, which leads to decreased absorption of quinolones. This is especially important, as will be discussed later, because quinolone antibiotics are used orally to treat serious infections. If they are not absorbed, treatment failure is likely to ensue.
Other important factors that must be understood to use antibiotics appropriately are host-specific factors, or host factors. These are factors that pertain specifically to a given patient, and they can have an important bearing on the success or failure of antibiotic therapy. Some of these host factors are age, allergy history, kidney and liver function, pregnancy status, genetic characteristics, site of infection, and host defenses.
Age-related host factors are those that apply to patients at either end of the age spectrum. For example, infants and children may not be able to take certain antibiotics such as tetracyclines, which affect developing teeth or bones; quinolones, which may affect bone or cartilage development in children; and sulfonamides, which may displace bilirubin from albumin and precipitate kernicterus (hyperbilirubinemia) in neonates. The aging process affects the function of various organ systems. As people age, there is a gradual decline in the function of the kidneys and liver, the organs primarily responsible for metabolizing and eliminating antibiotics. Therefore, depending on the level of kidney or liver function of a given older adult, dosage adjustments may be necessary. Pharmacists often play a role in evaluating the dosages of antibiotics and other medications to ensure optimal dosing for a given patient’s level of organ function.
A patient history of allergic reaction to an antibiotic is important in the selection of the most appropriate antibiotic. Penicillins and sulfonamides are two broad classes of antibiotic to which many people have allergic anaphylactic reactions. Symptoms of anaphylaxis include flushing, itching, hives, anxiety, fast irregular pulse, and throat and tongue swelling. The most dangerous such reaction is anaphylactic shock, in which a patient can suffocate from drug-induced respiratory arrest. Although this outcome is the most extreme, the potential for it does underscore the importance of consistently assessing patients for drug allergies and documenting any known allergies clearly in the medical record. All reported drug allergies are to be taken seriously and investigated further before a final decision is made about whether to administer a given drug. Many patients will say that they are “allergic” to a medication when in fact what they experienced was a common mild adverse effect such as stomach upset or nausea. Patients who report drug allergies need to be asked open-ended questions to elicit descriptions of prior allergic reactions so that the actual severity of the reaction can be assessed. The most common severe reactions to any medication that need to be noted in the patient’s chart are any difficulty breathing; significant rash, hives, or other skin reaction; and severe GI intolerance. Although some antibiotics are ideally taken on an empty stomach, eating a small amount of food with the medication may be sufficient to help the patient tolerate it and realize its therapeutic benefits.
Pregnancy-related host factors are also important to the selection of appropriate antibiotics, because several antibiotics can pass through the placenta and cause harm to the developing fetus. Drugs that cause development abnormalities in the fetus are called teratogens. Their use by pregnant women can result in birth defects.
Some patients also have certain genetic abnormalities that result in various enzyme deficiencies. These conditions can adversely affect drug actions in the body. Two of the most common examples of such genetic host factors are glucose-6-phosphate dehydrogenase (G6PD) deficiency and slow acetylation. The administration of antibiotics such as sulfonamides, nitrofurantoin, and dapsone to a person with G6PD deficiency may result in the hemolysis, or destruction, of red blood cells. Patients who are slow acetylators have a physiologic makeup that causes certain drugs to be metabolized more slowly than usual in a chemical step known as acetylation. This can lead to toxicity from drug accumulation (see Chapter 4).
The anatomic site of the infection is a very important host factor to consider when deciding not only which antibiotic to use but also the dosage, route of administration, and duration of therapy. Some antibiotics do not penetrate into the site of infection, such as the lung or bone or abscesses, which can lead to treatment failures.
Consideration of these host factors helps prescribers and pharmacists to ensure optimal drug selection for each individual patient. Continued patient assessment and proper monitoring of antibiotic therapy increase the likelihood that this therapy will be safe and effective.
Antibiotics
Antibiotics are classified into broad categories based on their chemical structure. The common categories include sulfonamides, penicillins, cephalosporins, macrolides, quinolones, aminoglycosides, and tetracyclines. In addition to chemical structure, other characteristics that distinguish classes of drugs include antibacterial spectrum, mechanism of action, potency, toxicity, and pharmacokinetic properties. The four most common mechanisms of antibiotic action are: (1) interference with bacterial cell wall synthesis, (2) interference with protein synthesis, (3) interference with replication of nucleic acids (deoxyribonucleic acid [DNA] and ribonucleic acid [RNA]), and (4) antimetabolite action that disrupts critical metabolic reactions inside the bacterial cell. Figure 38-3 portrays these mechanisms in combating bacterial infections and indicates which mechanism is used by several major antibiotic classes.
Perhaps the greatest challenge in understanding antimicrobial therapy is remembering the types and species of microorganisms against which a given drug can act. The list of microorganisms that a given drug has activity against can be quite extensive and can seem daunting to the inexperienced practitioner. Most antimicrobials have activity against only one type of microbe (e.g., bacteria, viruses, fungi, protozoans). However, a few drugs do have activity against more than one class of organisms.
The field of infectious disease treatment is continually evolving, largely because of the continual emergence of resistant bacterial strains. For this reason, drug indications change frequently, often from year to year, as various bacterial species become resistant to previously effective antiinfective therapy. It is always appropriate to check the most current reference materials or consult with colleagues (e.g., nurses, pharmacists, prescribers) when questions remain. Pharmacists are excellent resources regarding antibiotics. Many hospitals now have pharmacists who are specially trained in the treatment of infectious diseases.
Sulfonamides
Sulfonamides were one of the first groups of drugs used as antibiotics. Although there are many compounds in the sulfonamide family, only sulfamethoxazole combined with trimethoprim (a nonsulfonamide antibiotic), known as Bactrim, Septra, or co-trimoxazole and often abbreviated as SMX-TMP, is used commonly in clinical practice. Sulfisoxazole combined with erythromycin (a macrolide antibiotic) is occasionally used in pediatrics. Sulfasalazine, another sulfonamide, is used to treat ulcerative colitis and rheumatoid arthritis and is not used as an antibiotic.
Mechanism of Action and Drug Effects
Sulfonamides do not actually destroy bacteria but rather inhibit their growth. For this reason, they are considered bacteriostatic antibiotics. They inhibit the growth of susceptible bacteria by preventing bacterial synthesis of folic acid. Folic acid is a B-complex vitamin that is required for the proper synthesis of purines, one of the chemical components of nucleic acids (DNA and RNA). Chemical components of folic acid include paraaminobenzoic acid (PABA), pteridine, and glutamic acid. Specifically, in a process known as competitive inhibition, sulfonamides compete with PABA for the bacterial enzyme tetrahydropteroic acid synthetase, which incorporates PABA into the folic acid molecule. Because sulfonamides are capable of blocking a specific step in a biosynthetic pathway, they are also considered antimetabolites. Microorganisms that require exogenous folic acid (not synthesized by the bacterium itself) are not affected by sulfonamide antibiotics. Therefore, these drugs do not affect folic acid metabolism in human cells. Trimethoprim, although not a sulfonamide, works via a similar mechanism, inhibiting dihydrofolic acid reduction to tetrahydrofolate, which results in inhibition of the enzymes of the folic acid pathway.
Indications
Sulfonamides have a broad spectrum of antibacterial activity, including activity against both gram-positive and gram-negative organisms. These antibiotics achieve very high concentrations in the kidneys, through which they are eliminated. Therefore, sulfamethoxazole/trimethoprim is often used in the treatment of UTIs. The combination of these two drugs allows for a synergistic (see Chapter 2) antibacterial effect. Commonly susceptible organisms include strains of Enterobacter species (spp.), Escherichia coli, Klebsiella spp., Proteus mirabilis, Proteus vulgaris, and S. aureus. Unfortunately, however, resistant bacterial strains are a growing problem, as is the case with other antibiotic classes. Results of culture and sensitivity testing help to optimize drug selection in individual cases. This combination drug is also used for respiratory tract infections. However, it is now less effective against streptococci infecting the upper respiratory tract and pharynx. Another specific use for sulfamethoxazole/trimethoprim is prophylaxis and treatment of opportunistic infections in patients with human immunodeficiency virus (HIV) infection, especially infection by Pneumocystis jirovecii, a common cause of HIV-associated pneumonia. Sulfamethoxazole/trimethoprim is also a drug of choice for infection caused by the bacterium Stenotrophomonas maltophilia. Sulfamethoxazole/trimethoprim has become common treatment for outpatient Staphylococcus infections, due to the high rate of community-acquired methicillin-resistant S. aureus (MRSA) infections. MRSA and other resistant organisms are discussed in Chapter 39.
Contraindications
Use of sulfonamides is contraindicated in cases of known drug allergy to sulfonamides. Chemically related drugs such as the sulfonylureas (used to treat diabetes; see Chapter 32), thiazide and loop diuretics (see Chapter 28), and carbonic anhydrase inhibitors (see Chapter 28) are generally considered relatively safe in a patient who has a sulfonamide allergy. However, the cyclooxygenase-2 inhibitor celecoxib (Celebrex) should not be used (see Chapter 44) in patients with a known sulfonamide allergy. It is important to differentiate between sulfites and sulfonamides. Sulfites are commonly used as preservatives in everything from wine to food to injectable drugs. A person allergic to sulfonamide drugs may or may not also be allergic to sulfite preservatives. The use of sulfonamides is also contraindicated in pregnant women at term and in infants younger than 2 months of age.
Adverse Effects
Sulfonamide drugs are a common cause of allergic reaction. Patients will sometimes refer to this as “sulfa allergy” or even “sulfur allergy.” Although immediate reactions can occur, sulfonamides typically cause delayed cutaneous reactions. These reactions frequently begin with fever followed by a rash (morbilliform eruptions, erythema multiforme, or toxic epidermal necrolysis). Photosensitivity reactions are another type of skin reaction that is induced by exposure to sunlight during sulfonamide drug therapy. In some cases, such reactions can result in severe sunburn. Such reactions are also common with the tetracycline class of antibiotics discussed later in this chapter, as well as with various other drug classes and may occur immediately or have a delayed onset. Other reactions to sulfonamides include mucocutaneous, GI, hepatic, renal, and hematologic complications, all of which may be fatal in severe cases. It is believed that sulfonamide reactions are immune mediated and involve the production of reactive drug metabolites in the body. Reported adverse effects of the sulfonamides are listed in Table 38-2.
TABLE 38-2
SULFONAMIDES: REPORTED ADVERSE EFFECTS
BODY SYSTEM | ADVERSE EFFECTS |
Blood | Agranulocytosis, aplastic anemia, hemolytic anemia, thrombocytopenia |
Gastrointestinal | Nausea, vomiting, diarrhea, pancreatitis, hepatotoxicity |
Integumentary | Epidermal necrolysis, exfoliative dermatitis, Stevens-Johnson syndrome, photosensitivity |
Other | Convulsions, crystalluria, toxic nephrosis, headache, peripheral neuritis, urticaria, cough |
Interactions
Sulfonamides can have clinically significant interactions with a number of other medications. Sulfonamides may potentiate the hypoglycemic effects of sulfonylureas in diabetes treatment, the toxic effects of phenytoin, and the anticoagulant effects of warfarin, which can lead to hemorrhage. Sulfonamides may increase the likelihood of cyclosporine-induced nephrotoxicity. Patients receiving any of the above drug combinations may require more frequent monitoring. Sulfonamides, and all antibiotics, may also reduce the efficacy of oral contraceptives. Instruct patients taking these drugs to use additional contraceptive methods.
Dosages
For dosage information on selected sulfonamides, see the table on p. 616.
DOSAGES
Selected Sulfonamide Combination Drug Products
DRUG (PREGNANCY CATEGORY) | PHARMACOLOGIC CLASS | USUAL DOSAGE RANGE∗ | INDICATIONS |
Sulfamethoxazole/trimethoprim (co-trimoxazole, SMX-TMP, SMZ-TMP) (Bactrim, Septra) (C) | Sulfonamide and folate antimetabolite | Adult and pediatric IV/PO: 8-20 mg/kg/day divided twice a day (dose is in terms of trimethoprim component) | UTI, shigellosis enteritis; higher doses for nocardiosis and Pneumocystis jirovecii infection |
Adult PO: 160 mg TMP/800 mg SMX twice daily (every 12 hr) | P. jirovecii prophylaxis, acute exacerbation of chronic bronchitis | ||
Pediatric PO: 6-10 mg/kg/day (trimethoprim component) | Otitis media |
IV, Intravenous; PO, oral; TMP, trimethoprim; UTI, urinary tract infection.
∗Dosage ranges are typical but are not necessarily exhaustive due to space limitations. Clinical variations may occur. Check current drug handbook for exact dosages for specific indications.
Drug Profile
Sulfonamides work by interfering with bacterial synthesis of the essential nutrient folic acid. Most sulfonamide therapy today uses the combination drug sulfamethoxazole/trimethoprim.
sulfamethoxazole/trimethoprim (co-trimoxazole)
Co-trimoxazole (Bactrim) is a fixed-combination drug product containing a 5:1 ratio of sulfamethoxazole to trimethoprim. It is available in both oral and injectable dosage forms.
Route | Onset of Action | Peak Plasma Concentration | Elimination Half-life | Duration of Action |
PO | Variable | 2-4 hr | 7-12 hr | 12 hr |
Beta-Lactam Antibiotics
The beta-lactam antibiotics are very commonly used drugs. They are so named because of the beta-lactam ring that is part of their chemical structure (Figure 38-4). This broad group of drugs includes four major subclasses: penicillins, cephalosporins, carbapenems, and monobactams. They share a common structure and mechanism of action: they inhibit the synthesis of the bacterial peptidoglycan cell wall.
Some bacterial strains produce the enzyme beta-lactamase. This enzyme provides a mechanism for bacterial resistance to these antibiotics. The enzyme can break the chemical bond between the carbon (C) and nitrogen (N) atoms in the structure of the beta-lactam ring. When this happens, all beta-lactam drugs lose their antibacterial efficacy. Because of this, additional drugs known as beta-lactamase inhibitors are added to several of the penicillin antibiotics to make the drug more powerful against beta-lactamase–producing bacterial strains. Each of the four classes of beta-lactam antibiotics is examined in detail in the following sections.
Penicillins
The penicillins are a very large group of chemically related antibiotics that were first derived from a mold (fungus) often seen on bread or fruit. The penicillins can be divided into four subgroups based on their structure and the spectrum of bacteria they are active against: natural penicillins, penicillinase-resistant penicillins, aminopenicillins, and extended-spectrum penicillins. Examples of antibiotics in each subgroup and a brief description of their characteristics are given in Table 38-3.
TABLE 38-3
SUBCLASS | GENERIC DRUG NAMES | DESCRIPTION |
Natural penicillins | penicillin G, penicillin V | Although many modifications of the original natural (mold-produced) structure have been made, these are the only two in current clinical use. Penicillin G is the injectable form for IV or IM use; penicillin V is a PO dosage form (tablet and liquid). |
Penicillinase-resistant drugs | cloxacillin, dicloxacillin, nafcillin, oxacillin | Stable against hydrolysis by most staphylococcal penicillinases (enzymes that normally break down the natural penicillins). |
Aminopenicillins | amoxicillin, ampicillin | Have an amino group attached to the basic penicillin structure that enhances their activity against gram-negative bacteria compared with natural penicillins. |
Extended-spectrum drugs | piperacillin, ticarcillin, carbenicillin, piperacillin/tazobactam | Have wider spectra of activity than do all other penicillins. |
Penicillins are bactericidal antibiotics, meaning they kill a wide variety of gram-positive and some gram-negative bacteria. However, some bacteria have acquired the capacity to produce enzymes capable of destroying penicillins. These enzymes are called beta-lactamases, and they can inactivate the penicillin molecules by opening the beta-lactam ring. The beta-lactamases that specifically inactivate penicillin molecules are called penicillinases. Bacterial strains that produce these drug-inactivating enzymes were a therapeutic obstacle until drugs were synthesized that inhibit these enzymes. Three of these beta-lactamase inhibitors are clavulanic acid (also called clavulanate), tazobactam, and sulbactam. These drugs bind with the beta-lactamase enzyme itself to prevent the enzyme from breaking down the penicillin molecule, although they are not always effective. The following are examples of currently available combinations of a penicillin and a beta-lactamase inhibitor:
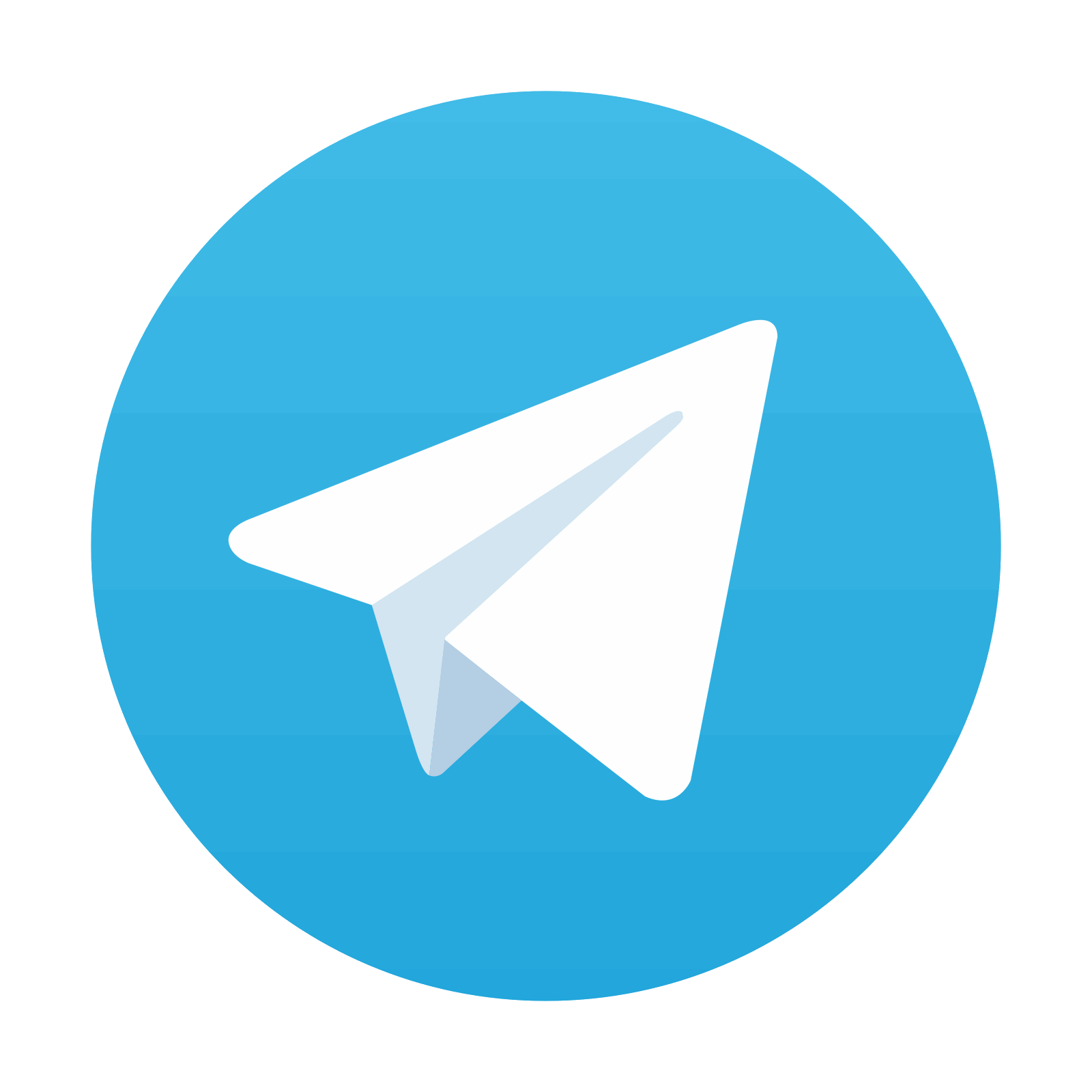
Stay updated, free articles. Join our Telegram channel

Full access? Get Clinical Tree
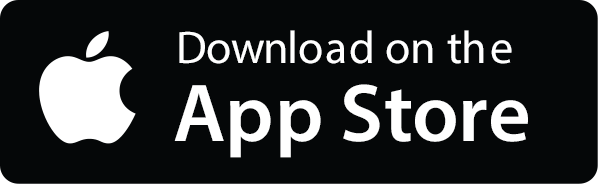
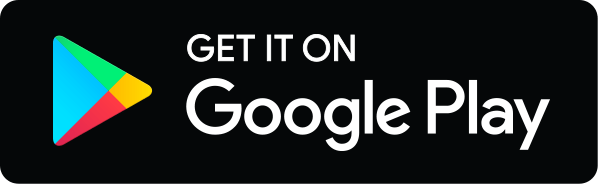